Servicios Personalizados
Revista
Articulo
Indicadores
-
Citado por SciELO
-
Accesos
Links relacionados
-
Citado por Google
-
Similares en SciELO
-
Similares en Google
Compartir
Acta Biológica Colombiana
versión impresa ISSN 0120-548X
Acta biol.Colomb. v.12 n.2 Bogotá jul./dic. 2007
EXPORT AND TRAFFICKING OF Plasmodium PROTEINS WITHIN THE HOST ERYTHROCYTE
Tráfico y exportación de proteínas de Plasmodium en el eritrocito hospedero
MARK F. WISER1, Ph. D. 1Department of Tropical Medicine, Tulane University School of Public Health. 1440 Canal Street, Suite 2210, SL17, New Orleans, LA. 701122824 504.988.2507 (voice), 504.988.7313 (fax) wiser@tulane.edu
Presentado por invitación el 24 de mayo de 2007.
SUMMARY
The export and trafficking of parasite proteins within the infected erythrocyte is a complex process and not well understood. Export of proteins from the parasite was previously speculated to involve a specialized compartment originally designated as the secondary endoplasmic reticulum of the Apicomplexa, or sERA. The properties of this Plasmodium export compartment are reviewed in regards to more recent observations about the trafficking of Plasmodium proteins within the host erythrocyte. In addition, a calcium ATPase unique to the Apicomplexa and with homology to the sarcoplasmicendoplasmic reticulum calcium ATPase is discussed in the context of this unique export compartment. It is also speculated that the Plasmodium export element, PEXEL, may play a role in targeting proteins to this parasite export compartment. Exported proteins are then proposed to move into the parasitophorous vacuole and those destined for the host erythrocyte are transported to the erythrocyte cytoplasm as soluble proteins. Chaperones probably play a role in escorting parasite proteins to their final destinations and assembly on the erythrocyte membrane.
Key words: secretory pathway, SERCA, protein trafficking, malaria, Plasmodium.
RESUMEN
El tráfico y exportación de proteínas del parásito dentro del citoplasma del eritrocito es un proceso complejo, que no es bien comprendido. Inicialmente se especuló sobre la manera como el parásito exporta éstas proteínas y se involucró un compartimiento que se denominó retículo endoplasmático secundario de los Apicomplexa (sERA). En este trabajo se revisan las propiedades de este compartimiento exportador de acuerdo con las observaciones más recientes del tráfico de proteínas de Plasmodium al salir del parásito. La discusión se enmarca dentro del contexto de este único compartimiento exportador relacionado con una ATPasa de calcio única de los Apicomplexa que presenta homología con la ATPasa de calcio del retículo endoplasmático sarcoplásmico (SERCA). Además, se especula que Plasmodium exporta elementos PEXEL, que podrían estar asociados con el direccionamiento de proteínas de Plasmodium hacia el compartimiento exportador y se propone que éstas proteínas van posteriormente a la vacuola parasitófora, y aquellas cuyo destino es la célula hospedera se transportan al citoplasma del eritrocito como proteínas solubles. Las chaperonas posiblemente escoltan las proteínas del parásito hasta su destino final y se ensamblan en la membrana del eritrocito.
Palabras clave: vía secretoria, SERCA, tráfico de proteínas, malaria, Plasmodium.
INTRODUCTION
The malaria parasite exhibits a complex life cycle involving a mosquito vector and a vertebrate host. During one stage of its life cycle the parasite infects erythrocytes. The blood stage is primarily responsible for the disease manifestations and generally establishes a chronic infection within the host. Like other Apicomplexa, the malaria parasite has specialized invasive forms (eg., merozoites) which are capable of entering the host cell. Invasion of host cells is accomplished through specialized secretory organelles called rhoptries, micronemes and dense granules (Cowman and Crabb, 2006). During parasite entry the merozoite forms a parasitophorous vacuole which is derived in part from the host erythrocyte membrane and the contents of the rhoptries (Soldati et al., 2004). Thus, the parasite does not lie within the cytoplasm of the erythrocyte, but is surrounded by a parasitophorous vacuolar membrane (PVM). In addition to these organelles that are specialized in the invasion process, there are other unique organelles within the parasite such as the food vacuole and apicoplast (Tonkin et al., 2006).
The malaria parasite also extensively modifies the host erythrocyte during the intraerythrocytic stage (Cooke et al., 2004b). For example, following infection the host erythrocyte becomes more permeable to low molecular metabolites and nutrients and new permeability pathways are induced in the host erythrocyte membrane (Kirk, 2004). This reflects the huge demand the actively growing and replicating parasite has for metabolites in the relatively impermeable erythrocyte. These new permeability pathways are probably the result of parasite proteins transported to the erythrocyte membrane (Baumeister et al., 2006).
Ultrastructural alterations are also observed in the host erythrocyte following infection. Well known ultrastructural alterations include the electrondense knobs on P. falciparuminfected erythrocytes and caveolavesicle complexes on P. vivaxinfected erythrocytes. The knobs on P. falciparuminfected erythrocytes play a role in the cytoadherence of infected erythrocytes to endothelial cells. This cytoadherence leads to a sequestration of the infected erythrocytes in the tissues and contributes to parasite survival by avoidance of the spleen and providing an environment of low oxygen tension. This sequestration of infected erythrocytes also plays a role in the pathogenesis of severe malaria.
Membranous structures are found throughout the host cell cytoplasm of infected erythrocytes. For example, Maurer's clefts are flattened lamellae of a single unit membrane usually located close to the erythrocyte membrane (Lanzer et al., 2006). Other membranous structures which appear to be extensions of the PVM are also found in the cytoplasm of the infected erythrocyte (Atkinson and Aikawa, 1990; Elford and Ferguson, 1993). These extensions of the PVM have also been referred to as the tubovesicular membrane (TVM) network (Elmendorf and Haldar, 1993a). Serial sections of infected erythrocytes suggest that the Maurer's clefts form a continuous membrane network which originates from the PVM at multiple sites and extends across the host erythrocyte (Wickert et al., 2004). It has also been suggested that the Maurer's clefts bud off from this membranous network and are distinct entities (Spycher et al., 2006).
In summary, the malaria parasite exhibits an unique cell biology with many specialized compartments that will require specific protein targeting and trafficking within the parasite (Tonkin et al., 2006). In addition, the parasite induces new compartments in the host erythrocyte and is able to specifically target proteins to distinct extracellular locations: the parasitophorous vacuole, the PVM (including membranous extensions continuous with the PVM), Maurer's clefts, and the modified host erythrocyte membrane. How the parasite induces these new compartments in the host erythrocyte and is able to specifically target proteins to extracellular locations is a central question in Plasmodium cell biology. This review will discuss a novel secretory compartment in the malaria parasite and its possible role in modifying the host erythrocyte.
A NOVEL SECRETORY COMPARTMENT IN PLASMODIUM
The malaria parasite is a eukaryotic organism and presumably exhibits a secretory pathway similar to other eukaryotic organisms. In this regard, components of the secretory pathway have been identified in the genome of Plasmodium (Carlton et al., 2002; Gardner et al., 2002). The first step in the export of proteins is generally the endoplasmic reticulum. However, many Plasmodium proteins destined for export into the host erythrocyte appear to accumulate in a compartment distinct from the ER following treatment with brefeldin A (BFA). BFA is a fungal metabolite that blocks secretion by leading to disassembly of the Golgi apparatus and an accumulation of exported proteins in the ER (Chardin and McCormick, 1999). Treatment of infected erythrocytes with BFA results in the accumulation of exported Plasmodium proteins in a single compartment located near the parasite periphery (Wiser et al., 1997; Wiser et al., 1999b). This compartment appears as either a flattened disk or has a tubular shape when examined by immunofluorsecence. Ultrastructural studies and analysis by density gradients indicate that this novel compartment is membrane bound (Wiser et al., 1999a). Several Plasmodium proteins, which are exported into the host erythrocyte, accumulate in this novel comparment located at the parasite periphery following BFA treatment (Table 1). Furthermore, proteins destined for different locations within the infected erythrocyte colocalize to the same compartment following BFA treatment (Wiser et al., 1997).
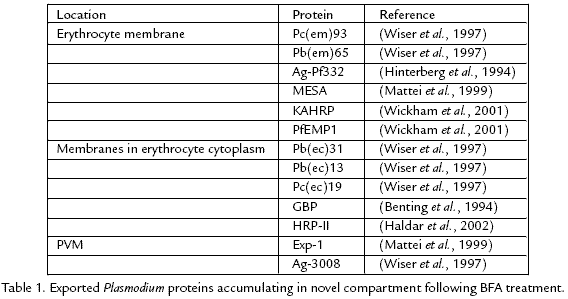
In contrast, merozoite surface protein1, a protein on the plasma membrane of the parasite, exhibits a diffuse mottled pattern throughout the cytoplasm of the parasite following BFA treatment, which is similar to the pattern exhibited by ER protein BiP (Wiser et al., 1997). This same reticular pattern has been previously reported for BiP (Kumar et al., 1991; Elmendorf and Haldar, 1993b) as well as the P. falciparum reticulocalbin homologue, a Ca2+binding protein localized to the ER (La Greca et al., 1997). This reticulated pattern and the ultrastructural localization of BiP and reticulocalbin to endomembranes throughout the parasite cytoplasm are consistent with the parasite ER being a loose network of vesicles as previously described (Langreth et al., 1978). Thus it appears that there are two BFAsensitive export pathways in the malaria parasite: one characterized by a diffuse reticular distribution and representing the classical ER, and the other characterized by a single compartment located at the periphery of the parasite.
This BFAinduced compartment at the parasite periphery is proposed to be involved in the export of Plasmodium proteins into the host erythrocyte. Proteins destined to be exported to the host erythrocyte traverse this Plasmodium export compartment instead of the ER. This novel export compartment may be part of an alternate secretory pathway distinct from the classical ER and Golgi. Although many exported Plasmodium proteins utilize this novel compartment, other mechanisms of protein export probably coexist since some protein export occurs via a BFAinsensitive pathway (Elmendorf et al., 1992; Moura and Pudles, 1999).
Several Plasmodium proteins are associated with a compartment with a similar morphology as the proposed Plasmodium export compartment in the absence of BFA treatment (Table 2). For example, several monoclonal antibodies raised against a membranous fraction isolated from P. falciparuminfected erythrocytes exhibited an immunofluorescence pattern similar to the novel BFAinduced compartment (Cortes et al., 2003). Colocalization studies confirm that the proteins recognized by these monoclonal antibodies are localized to the proposed Plasmodium export compartment. These colocalization studies indicate that this compartment is not simply an artifact of BFA treatment in that this compartment exists in the absence of BFA. Three distinct proteins of molecular masses 68, 45 and 22 kDa are localized to the Plasmodium export compartment and these three proteins are conserved between P. falciparum and the rodent malaria parasites. The identities of these proteins are not known. Knowing the identities of these proteins will provide some insight into the nature of this Plasmodium export compartment.
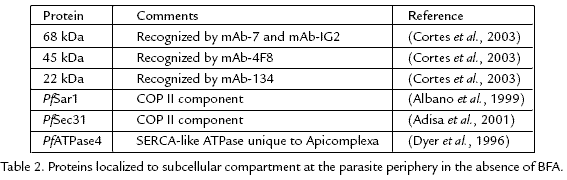
These monoclonal antibodies have been used to characterize the export compartment during erythrocytic stage schizogony. The structure recognized by these monoclonal antibodies is observed in the early ring stage and is continuously present throughout the entire blood stage (Cortes et al., 2003). The structure increases in size during the trophozoite stage as the parasite grows and may reflect an increase in protein trafficking as the parasite matures. During schizogony the compartment recognized by the monoclonal antibodies divides into smaller structures that are associated with the individual budding merozoites.
Two other proteins possibly localized to the Plasmodium export compartment are PfSar1 (Albano et al., 1999) and PfSec31 (Adisa et al., 2001). Both of these proteins are homologues of COPII components of which form coats around vesicles involved in ERGolgi transport (Wieland and Harter, 1999). Colocalization studies using antibodies against the COPII components and monoclonal antibodies discussed above reveal that there is substantial overlap between the compartments recognized by the antiCOPII antibodies and these monoclonal antibodies. However, the compartments recognized by antiPfSar1p and antiPfSec31p do not coincide exactly with the monoclonal antibodies (Cortes et al., 2003). The COPII components and the 68, 45 and 22 kDa proteins colocalize to the compartment along the parasite periphery. But the COPII components exhibit a more extensive distribution within the parasite in that there is a diffuse mottled labeling over the entire parasite. This diffuse mottled pattern is quite reminiscent of the ER and these COPII components partially colocalize with the ER markers BiP and PfERC (Albano et al., 1999; Adisa et al., 2001). These results suggest that COPII components are localized to both the ER and to the Plasmodium export compartment.
The accumulation of exported proteins following BFA treatment and the colocalization of COPII components to the Plasmodium export compartment suggests that it has similar properties as the ER. In addition, an ERlike calcium ATPase is speculated to be localized to this novel compartment (see below). These observations suggest that the Plasmodium export compartment may be a specialized domain of the ER or a separate ERlike organelle. The more extensive distribution of the COPII components in the parasite as compared to the 68, 45 and 22 kDa proteins is consistent with functionally distinct regions of ER. Similarities between this novel compartment and the ER have been previously noted (Wiser et al., 1997; Wiser et al., 1999b) and this compartment was called the secondary ER of Apicomplexa (sERA). These ERlike properties are also consistent with the proposal that this novel export compartment is the first step in an alternate secretory pathway specialized in the export of proteins into the host erythrocyte. Proteins destined for export into the host erythrocyte would be targeted to this special ER domain or ERlike organelle whereas proteins destined for compartments within the parasite would be targeted to other domains of the ER.
ERLIKE CALCIUM ATPASES OF APICOMPLEXA
Antibodies raised against a Ptype ATPase from P. falciparum, called ATPase4, label a flattened diskshaped structure at the parasite periphery (Dyer et al., 1996). Unfortunately these antibodies were no longer available and it could not be determined if this compartment is the same as the novel Plasmodium export compartment (Wiser et al., 1997). ATPase4 and another P. falciparum ATPase, called ATPase6, both exhibit homology to the sarcoplasmicendoplasmic reticulum calcium ATPase (SERCA). The observation that Plasmodium has two distinct SERCAlike genes and that both PfATPase4 (Dyer et al., 1996) and PfATPase6 (Kimura et al., 1993) are expressed during the blood stage is consistent with the hypothesis that Plasmodium has functionally distinct ERlike compartments. No localization data are available for ATPase6.
Searching all of the available sequence databases indicate that orthologs of both ATPase4 and ATPase6 are found in other Plasmodium species as well as other Apicomplexa. Othologs for both ATPase4 and ATPase6 were found in all Apicomplexa genomes which are completely sequenced. Phylogenetic analysis indicates that the ATPase4 orthologs form a clade distinct from the ATPase6 orthologs (Figure 1). Previous studies also indicate that ATPase6 is more homologous to SERCA and that ATPase4 may define a group of Ca2+ATPases which is specific to Apicomplexa (Krishna et al., 2001; Nagamune and Sibley, 2006).
Interestingly, antibodies raised against the Cryptosporidium ATPase4 ortholog recognize two distinct proteins of 160 kDa and 146 kDa on immunoblots and localization studies reveal two distinct patterns (Zhu and Keithly, 1997). One pattern is a diffuse perinuclear location, presumably the ER, and the other pattern is discrete vesicles near the parasite periphery at its apical end. These results suggest that the antibodies are recognizing two distinct proteins in two separate compartments. Furthermore, the sizes of the proteins recognized by the antibody are consistent with the predicted sizes of the ATPase4 and ATPase6 orthologs from Cryptosporidium. Cryptosporidium is more closely related to the gregarines and probably emerged early in Apicomplexan evolution (Zhu et al., 2000) suggesting that the duality of ERlike calcium ATPases may be a feature common among the Apicomplexa. Clearly more work on the locations and functions of the SERCAlike ATPases from the Apicomplexa is needed.
Other interesting observations in regards to Ca2+ATPases are the labeling of clefts within >P. vivax infected erythrocytes with monoclonal antibodies which recognizes SERCA from a wide variety of organisms (Bracho et al., 2002) and the histochemical localization of Ca2+ATPase activity to Mauers' clefts in P. falciparum (Caldas and Wasserman, 2001).
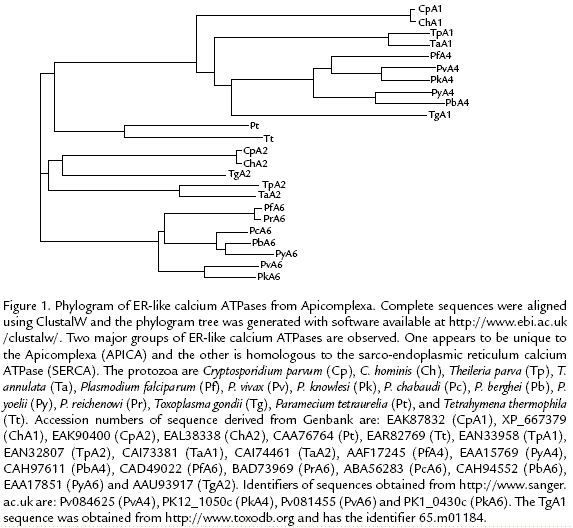
The SERCA ATPase from ciliates exhibits approximately equal homology to both ATPase4 and ATPase6 (Figure 1). The ciliates are a sister group to the apicomplexa and dinoflagellates within the Alveolata (Leander and Keeling, 2004). The ciliates probably only have one SERCA since the genome of Tetrahymena is completely sequenced (Eisen et al., 2006). Therefore the duplication of SERCA in the apicomplexa likely occurred after the divergence of the apicomplexa and ciliates. SERCA from the ciliates is localized to the alveolar sacs (Hauser et al., 1998), which are flatten membranebound compartments found just below the plasma membrane. In Apicomplexa the structures homologous to aveolar sacs are found in the invasive stages and are often called the inner membrane complex. Similarities between alveolar sacs and the ER have been previously noted (Länge et al., 1995; de Melo and de Souza, 1997).
EXTRAPARASITE TRAFFICKING
The observation that many parasite proteins destined for different locations within the host erythrocyte appear to utilize this novel Plasmodium export compartment raises some questions about the sorting process within the infected erythrocyte. Presumably proteins will move from this novel compartment into the parasitophorous vacuole. Some exported proteins are known to pass through the parasitophorous vacuole en route to their final destinations within the infected erythrocyte (Ansorge et al., 1996; Wickham et al., 2001). The juxtaposition of the novel export compartment with the parasite plasma membrane implies that proteins may move directly into the parasitophorous vacuole. However, there is no evidence of any transient direct connections between the export compartment and the plasma membrane.
Once within the parasitophorous vacuole the exported proteins need to be sorted and trafficked to their final destinations. The movement of proteins from compartments within the parasite to compartments in the host erythrocyte presents some topological problems and models to account for these problems have been proposed (Przyborski and Lanzer, 2005; Lingelbach and Przyborski, 2006). Many of these models involve a vesiclemediated transport within the infected erythrocyte. However, many exported Plasmodium proteins appear to be exported into the host erythrocyte as soluble proteins. For example, studies on the trafficking of P. falciparum erythrocyte membrane protein1 (PfEMP1) suggest that the protein is trafficked as a complex rather than in vesicles (Knuepfer et al., 2005a; Papakrivos et al., 2005). In addition, the Cterminal region of PfEMP1 binds to knobassociated histidine rich protein (KAHRP; Rug, 2006). These observations imply that PfEMP1 is exported into the host erythrocyte as a soluble protein and is directed to its final destination via interactions with other proteins. PfEMP3 is also trafficked as a complex with other proteins in the cytoplasm of the host erythrocyte (Knuepfer et al., 2005b).
In addition, several Plasmodium proteins that are localized to the host erythrocyte membrane, including KAHRP, bind to the submembrane cytoskeleton on the cytoplasmic face of the erythrocyte membrane (Lustigman et al., 1990; Wiser et al., 1990; Foley et al., 1991; Kilejian et al., 1991). These observations imply that a potential mechanism of trafficking within the infected erythrocyte involves the secretion of soluble proteins into the erythrocyte cytoplasm and targeting involves binding to other proteins. Interestingly, many of the proposed exported proteins are chaperones (Hiller et al., 2004; Sargeant et al., 2006) and several chaperones are found in parasitophorous vacuole (Nyalwidhe and Lingelbach, 2006). The role of these exported chaperones may be to escort secreted proteins to their final destinations and assist in the formation of supramolecular structures such as knobs. Host chaperones have also been shown to be associated with knobenriched erythrocyte membrane fractions (Banumathy et al., 2001).
Such a model for trafficking within the erythrocyte raises questions about how proteins are released from the parasitophorous vacuole. One possibility is that transporters are found on the PVM which transfer proteins from the parasitophorous vacuole to the erythrocyte cytoplasm. However, no such transporters have been identified on the PVM.
Pores on alveolar membranes of the malaria parasite have been described and these pores are speculated to be involved in protein transport (Raibaud et al., 2001). In addition, diffuse membranous structures found at the PVM have been proposed to be involved in the release of material from the parasitophorous vacuole into the erythrocyte cytoplasm (Lanners et al., 1999). Once released into the erythrocyte cytoplasm the exported proteins could then bind to their final destination or associate with chaperones which will assist in targeting the proteins to their correct destinations.
Several exported proteins destined for the erythrocyte membrane are transiently found on Maurer's clefts before arriving at their final erythrocyte membrane destination (Hinterberg et al., 1994; Wickham et al., 2001; Kriek et al., 2003). This suggests that the Maurer's clefts function as an intermediate step in the trafficking of proteins within the host erythrocyte. In particular, the KAHRP (Wickham et al., 2001) and PfEMP1 (Kriek et al., 2003) are known to be associated with Maurer's clefts before the final assembly of the knobs on the erythrocyte membrane. For example, PfEMP1 is synthesized and trafficked as a peripheral membrane protein to the Maurer's clefts and then subsequently inserted through erythrocyte membrane from the Maurer's clefts (Papakrivos et al., 2005). This trafficking to the Maurer's clefts and insertion of PfEMP1 into the erythrocyte membrane requires a Maurer's cleft protein called skeleton binding protein1 (SBP1) (Cooke et al., 2006; Maier et al., 2007). However, other erythrocyte membrane associated proteins do not require SBP1 for their trafficking to erythrocyte membrane. Therefore, SBP1 may play a specific role in the trafficking of PfEMP1 and its insertion into the host membrane. In addition, the cholesterol rich domains on the erythrocyte membrane are important for the insertion of PfEMP1 into the erythrocyte membrane (Frankland et al., 2006).
Another possibility is that proteins can move along the extensions of the PVM. Such a mechanism may be used by proteins which are resident within these PVM extensions and ultimately the Maurer's clefts. For example, GRA3, a Toxoplasma protein, integrates into the PVM following secretion of a soluble form into the parasitophorous vacuole (Ossorio et al., 1994), and a similar mechanism for the incorporation of P. falciparum Exp2 in the PVM has been proposed (Fischer et al., 1998).
TARGETING SEQUENCES
Another major question in regards to the trafficking of proteins to the host erythrocyte concerns targeting sequences. Some exported Plasmodium proteins contain canonical Nterminal signal sequences, whereas other exported proteins contain a hydrophobic region resembling a signal sequence that is recessed by up to 80 amino acids (Cooke et al., 2004a). This hydrophobic signal sequence is sufficient for targeting proteins to the parasitophorous vacuole but additional signals are needed for trafficking into the host erythrocyte. A short sequence motif necessary for transport beyond the PVM has been identified (Hiller et al., 2004; Marti et al., 2004). This Plasmodium export element (PEXEL) is located 1520 amino acids downstream of the hydrophobic signal sequence and has a consensus sequence of RxLxE/Q. Many of the genes contain an intron between the signal sequence and PEXEL with the PEXEL motif close to the beginning of the second exon. Not all exported proteins have this targeting motif though. SBP1 has neither a signal sequence nor the PEXEL, PfEMP1 has the PEXEL but no signal sequence, and EXP1 has a signal sequence, but no PEXEL. Such proteins may need to be escorted to their final destinations by other proteins with the proper signals.
Proteins destined for different locations within the infected erythrocyte contain the PEXEL. In addition, both soluble and membrane exported proteins have the PEXEL. This implies that the PEXEL functions at a step before the proteins have separated into distinct trafficking pathways. In other words, PEXEL probably functions in the secretory pathway before the parasitophorous vacuole. For example, PEXEL in combination with the signal sequence may target proteins to Plasmodium export compartment. As discussed above, the exported proteins are then transferred to the parasitophorous vacuole for subsequent sorting and trafficking. Thus, PEXEL may function twice in the secretory process. The first function is to target the protein to the Plasmodium export compartment and then later as a signal to exit the parasitophorous vacuole.
The PEXEL motif has not been identified in other apicomplexa and may be unique to Plasmodium (Sargeant et al., 2006). If PEXEL is involved in targeting exported proteins to the Plasmodium export compartment, then this compartment may also be unique to Plasmodium. Thus, the earlier designation of this compartment as the secondary ER of the apicomplexa (sERA) may not be accurate. However, all Apicomplexa do appear to have the unique SERCAlike calcium ATPase. Clearly more work is needed on resolving if PEXEL plays a role in targeting proteins to the Plasmodium export compartment and the function and location of the Apicomplexan calcium ATPase.
SUMMARY AND MODEL
The malaria parasite exports many proteins to distinct locations within the host erythrocyte. Many of these exported proteins appear to traverse a specialized compartment within the parasite that functions in the export of proteins into the host erythrocyte. This Plasmodium export compartment exhibits many ERlike properties and may be a specialized domain of the ER or a distinct ERlike organelle. The observation that Plasmodium and other Apicomplexa have two SERCAlike ATPases is consistent with two ERlike organelles. It is proposed that proteins destined for compartments within the parasite utilize the classical ER, whereas proteins destined for export into the host erythrocyte are targeted for the Plasmodium export compartment (Figure 2). Proteins then move from the Plasmodium export compartment into the parasitophorous vacuole by an unknown mechanism. Some sorting may occur within the parasitophorous vacuole. For example, proteins that are resident to the PVM or the extensions of the PVM and possibly the Maurer's clefts may move along these extensions. Proteins destined for the erythrocyte are likely exported into the cytoplasm of the host erythrocyte as soluble proteins. Some of these proteins may bind directly to their final destination such as the erythrocyte membrane. Others may associate transiently with Maurer's clefts or other membranes within the infected erythrocyte. Chaperones may also be involved in the trafficking of proteins to their final destinations and the assembly of supramolecular complexes such as knobs.

erythrocyte. Proteins destined for locations within the parasite are trafficked through the endoplasmic reticulum (ER) and sorted to the various compartments such as the apicoplast (Ap), food vacuole (FV), and parasite plasma membrane. Many exported proteins are targeted to the Plasmodium export compartment (PEC) instead of the ER in route to the parasitophorous vacuole (PV). The PEC could also be a specialized domain of the ER. Some proteins will be retained in the PV or associate with the PVM or the extensions of the PVM, whereas others will be transported into the host erythrocyte cytoplasm as soluble proteins. These proteins will then associate with membranous compartments within the erythrocyte or the erythrocyte membrane. Chaperones may play a role in the trafficking of exported proteins or their association with supramolecular structures. Many proteins, especially those associated with knobs, assemble on the Maurer's clefts or other membranes within the cytoplasm of the host erythrocyte before their final transfer to the erythrocyte membrane.
ACKNOWLEDGEMENT
I thank Gladys Cortés for her help in translating the summary into Spanish.
BIBLIOGRAPHY
ADISA A, REEDER J, ALBANO FR, FOLEY M, TILLEY L. Evidence for a Role for a Plasmodium falciparum Homologue of Sec31p in the Export of Proteins to the Surface of Malaria ParasiteInfected Erythrocytes. J Cell Sci. 2001;114:3377-3386.
[ Links ]ALBANO FR, BERMAN A, LA GRECA N, HIBBS AR, WICKHAM M, FOLEY M et al. A Homologue of Sar1p Localises to a Novel Trafficking Pathway in MalariaInfected Erythrocytes. Eur J Cell Biol. 1999;78:453-462.
[ Links ]ANSORGE I, BENTING J, BHAKDI S, LINGELBACH K. Protein Sorting in Plasmodium falciparumInfected Red Blood Cells Permeabilized with the PoreForming Protein Streptolysin O. Biochem J. 1996;315:307-314.
[ Links ]ATKINSON CT, AIKAWA M. Ultrastructure of MalariaInfected Erythrocytes. Blood Cells. 1990;16:351-368.
[ Links ]BANUMATHY G, SINGH V, TATU U. Host Chaperones Are Recruited in MembraneBound Complexes by Plasmodium falciparum. J Biol Chem. 2001;277:3902-3912.
[ Links ]BAUMEISTER S, WINTERBERG M, DURANTON C, HUBER SM, LANG F, KIRK K, et al. Evidence for the Involvement of Plasmodium falciparum Proteins in the Formation of New Permeability Pathways in the Erythrocyte Membrane. Mol Microbiol. 2006;60:493-504.
[ Links ]BENTING J, MATTEI D, LINGELBACH K. Brefeldin A Inhibits Transport of the GlycophorinBinding Protein from Plasmodium falciparum into the Host Erythrocyte. Biochem J. 1994;300:821-826.
[ Links ]BRACHO C, DUNIA I, DE LR, BENEDETTI EL, PEREZ HA. Traffic Pathways of Plasmodium vivax Antigens During Intraerythrocytic Parasite Development. Parasitol Res. 2002;88:253-258.
[ Links ]CALDAS ML, WASSERMAN M. Cytochemical Localisation of Calcium Atpase Activity During the Erythrocytic Cell Cycle of Plasmodium falciparum. Int J Parasitol. 2001;31:776-782.
[ Links ]CARLTON JM, ANGIUOLI SV, SUH BB, KOOIJ TW, PERTEA M, SILVA JC et al. Genome Sequence and Comparative Analysis of the Model Rodent Malaria Parasite Plasmodium yoelii yoelii. Nature. 2002;419:512-519.
[ Links ]CHARDIN P, MCCORMICK F. Brefeldin A: The Advantage of Being Uncompetitive. Cell. 1999;97:153-155.
[ Links ]COOKE BM, LINGELBACH K, BANNISTER LH, TILLEY L. Protein Trafficking in Plasmodium falciparumInfected Red Blood Cells. Trends Parasitol. 2004a;20:581-589.
[ Links ]COOKE BM, MOHANDAS N, COPPEL RL. Malaria and the Red Blood Cell Membrane. Semin Hematol. 2004b;41:173-188.
[ Links ]COOKE BM, BUCKINGHAM DW, GLENISTER FK, FERNANDEZ KM, BANNISTER LH, MARTI M et al. A Maurer's CleftAssociated Protein Is Essential for Expression of the Major Malaria Virulence Antigen on the Surface of Infected Red Blood Cells. J Cell Biol. 2006;172:899-908.
[ Links ]CORTES GT, WINOGRAD E, WISER MF. Characterization of Proteins Localized to a Subcellular Compartment Associated with an Alternate Secretory Pathway of the Malaria Parasite. Mol Biochem Parasitol. 2003;129:127-135.
[ Links ]COWMAN AF, CRABB BS. Invasion of Red Blood Cells by Malaria Parasites. Cell. 2006;124:755-766.
[ Links ]DE MELO EJT, DE SOUZA W. A Cytochemical Study of the Inner Membrane Complex of the Pellicle of Tachyzoites of Toxoplasma gondii. Parasitol Res. 1997;83:252-256.
[ Links ]DYER M, JACKSON M, MCWHINNEY C, ZHAO G, MIKKELSEN R. Analysis of a CationTransporting Atpase of Plasmodium falciparum. Mol Biochem Parasitol. 1996;78:1-12.
[ Links ]EISEN JA, COYNE RS, WU M, WU D, THIAGARAJAN M, WORTMAN JR et al. Macronuclear Genome Sequence of the Ciliate Tetrahymena thermophila, a Model Eukaryote. PLoS Biology. 2006;4:e286.
[ Links ]ELFORD BC, FERGUSON DJP. Secretory Processes in Plasmodium. Parasitol Today. 1993;9:80-81.
[ Links ]ELMENDORF HG, BANGS JD, HALDAR K. Synthesis and Secretion of Proteins by Released Malarial Parasites. Mol Biochem Parasitol. 1992;52:215-230.
[ Links ]ELMENDORF HG, HALDAR K. Secretory Transport in Plasmodium. Parasitol Today. 1993a;9:98-102.
[ Links ]ELMENDORF HG, HALDAR K. Identification and Localization of Erd2 in the Malaria Parasite Plasmodiumfalciparum Separation from Sites of Sphingomyelin Synthesis and Implications for Organization of the Golgi. EMBO J. 1993b;12:4763-4773.
[ Links ]FISCHER K, MARTI T, RICK B, JOHNSON D, BENTING J, BAUMEISTER S et al. Characterization and Cloning of the Gene Encoding the Vacuolar Membrane Protein Exp2 from Plasmodium falciparum. Mol Biochem Parasitol. 1998;92:47-57.
[ Links ]FOLEY M, TILLEY L, SAWYER WH, ANDERS RF. The RingInfected Erythrocyte Surface Antigen of Plasmodium falciparum Associates with Spectrin in the Erythrocyte Membrane. Mol Biochem Parasitol. 1991;46:137-148.
[ Links ]FRANKLAND S, ADISA A, HORROCKS P, TARASCHI TF, SCHNEIDER T, ELLIOT SR et al. Delivery of the Malaria Virulence Protein Pfemp1 to the Erythrocyte Surface Requires CholesterolRich Domains. Eukaryotic Cell. 2006;5:849-860.
[ Links ]GARDNER MJ, HALL N, FUNG E, WHITE O, BERRIMAN M, HYMAN RW et al. Genome Sequence of the Human Malaria Parasite Plasmodium falciparum. Nature. 2002;419:498-511.
[ Links ]HALDAR K, MOHANDAS N, SAMUEL BU, HARRISON T, HILLER NL, AKOMPONG T et al. Protein and Lipid Trafficking Induced in Erythrocytes Infected by Malaria Parasites. Cell Microbiol. 2002;4:383-395.
[ Links ]HAUSER K, PAVOLVIC N, KISSMEHL R, PLATTNER H. Molecular Characterization of a Sarco(Endo)Plasmic Reticulum Ca2+Atpase Gene from Paramecium tetraurelia and Localization of Its Gene Product Ot SubPlasmalemmal Calcium Stores. Biochem J. 1998;334:31-38.
[ Links ]HILLER NL, BHATTACHARJEE S, VAN OOIJ C, LIOLIOS K, HARRISON T, LOPEZESTRAÑO C et al. A HostTargeting Signal in Virulence Proteins Reveals a Secretome in Malarial Infection. Science. 2004;306:1934-1937.
[ Links ]HINTERBERG K, SCHERF A, GYSIN J, TOYOSHIMA T, AIKAWA M, MAZIE JC et al. Plasmodium falciparum: The Pf332 Antigen Is Secreted from the Parasite by a Brefeldin ADependent Pathway and Is Translocated to the Erythroycyte Membrane Via the Maurer's Clefts. Exp Parasitol. 1994;79:
[ Links ]KILEJIAN A, RASHID MA, AIKAWA M, AJI T, YANG YF. Selective Association of a Fragment of the Knob Protein with Spectrin. Mol Biochem Parasitol. 1991;44:175-182.
[ Links ]KIMURA M, YAMAGUCHI Y, TAKADA S, TANABE K. Cloning of a Ca2+Atpase Gene of Plasmodium falciparum and Comparison with Vertebrate Ca2+Atpases. J Cell Sci. 1993;104:1129-1136.
[ Links ]KIRK K. Channels and Transporters as Drug Targets in the PlasmodiumInfected Erythrocyte. Acta Trop. 2004;89:285-298.
[ Links ]KNUEPFER E, RUG M, KLONIS N, TILLEY L, COWMAN AF. Trafficking of the Major Virulence Factor to the Surface of Transfected P. falciparumInfected Erythrocytes. Blood. 2005a;105:4078-4087.
[ Links ]KNUEPFER E, RUG M, KLONIS N, TILLEY L, COWMAN AF. Trafficking Determinants for Pfemp3 Export and Assembly under the Plasmodium falciparumInfected Red Blood Cell Membrane. Mol Microbiol. 2005b;58:1039-1053.
[ Links ]KRIEK N, TILLEY L, HORROCKS P, PINCHES R, ELFORD BC, FERGUSON DJ et al. Characterization of the Pathway for Transport of the CytoadherenceMediating Protein, Pfemp1, to the Host Cell Surface in Malaria ParasiteInfected Erythrocytes. Mol Microbiol. 2003;50:1215-1227.
[ Links ]KRISHNA S, WOODROW C, WEBB R, PENNY J, TAKEYASU K, KIMURA M et al. Expression and Functional Characterization of a Plasmodium falciparum Ca2+Atpase (Pfatp4) Belonging to a Subclass Unique to Apicomplexan Organisms. J Biol Chem. 2001;276:10782-10787.
[ Links ]KUMAR N, KOSKI G, HARADA M, AIKAWA M, ZHENG H. Induction and Localization of Plasmodium falciparum Stress Proteins Related to the Heat Shock Protein 70 Family. Mol Biochem Parasitol. 1991;48:47-58.
[ Links ]LA GRECA N, HIBBS AR, RIFFKIN C, FOLEY M, TILLEY L. Identification of an Endoplasmic ReticulumResident CalciumBinding Protein with Multiple EfHand Motifs in Asexual Stages of Plasmodium falciparum. Mol Biochem Parasitol. 1997;89:283-293.
[ Links ]LÄNGE S, KLAUKE N, PLATTNER H. Subplasmalemmal Ca2+ Stores of Probable Relevance for Exocytosis in Paramecium. Aveolar Sacs Share Some but Not All Characteristics with Sarcoplasmic Reticulum. Cell Calcium. 1995;17:335-344.
[ Links ]LANGRETH SG, JENSEN JB, REESE RT, TRAGER W. Fine Structure of Human Malaria in vitro. J Protozool. 1978;25:443-452.
[ Links ]LANNERS HN, BAFFORD RA, WISER MF. Characterization of the Parasitophorous Vacuole Membrane from Plasmodium chabaudi and Implications About Its Role in the Export of Parasite Proteins. Parasitol Res. 1999;85:349-355.
[ Links ]LANZER M, WICKERT H, KROHNE G, VINCENSINI L, BRAUN BC. Maurer's Clefts: A Novel MultiFunctional Organelle in the Cytoplasm of Plasmodium falciparumInfected Erythroyctes. Int J Parasitol. 2006;36:23-36.
[ Links ]LEANDER BS, KEELING PJ. Early Evolutionary History of Dinoflagellates and Apicomplexans (Alveolata) as Inferred from Hsp90 and Actin Phylogenies. Journal of Phycology. 2004;40:341-350.
[ Links ]LINGELBACH K, PRZYBORSKI JM. The Long and Winding Road: Protein Trafficking Mechanisms in the Plasmodium falciparum Infected Erythrocyte. Mol Biochem Parasitol. 2006;147:1-8.
[ Links ]LUSTIGMAN S, ANDERS RF, BROWN GV, COPPEL RL. The Mature ParasiteInfected Erythrocyte Surface Antigen (Mesa) Associates with the Erythrocyte Membrane Skeletal Protein, Band 4.1. Mol Biochem Parasitol. 1990;38:261-270.
[ Links ]MAIER AG, RUG M, O'NEILL MT, BEESON JG, MARTI M, REEDER J et al. SkeletonBinding Protein 1 Functions at the Parasitophorous Vacuole Membrane to Traffic Pfemp1 to the Plasmodium falciparumInfected Erythrocyte Surface. Blood. 2007;109:1289-1297.
[ Links ]MARTI M, GOOD RT, RUG M, KNUEPFER E, COWMAN AF. Targeting Malaria Virulence and Remodeling Proteins to the Host Erythrocyte. Science. 2004;306:1930-1933.
[ Links ]MATTEI D, BERRY L, COUFFIN S, RICHARD O. The Transport of the HistidineRich Protein 1 (Hrp1) from Plasmodium falciparum Is Insensitive to Brefeldin A. In: Bock Gr, Cardew G, eds. Transport and Trafficking in the MalariaInfected Erythrocyte (Novartis Foundation Symposium 226). Chichester: Wiley; 1999. p. 215-230.
[ Links ]MOURA IC, PUDLES J. A Plasmodium chabaudi chabaudi High Molecular Mass Glycoprotein Translocated to the Host Cell Membrane by a NonClassical Secretory Pathway. Eur J Cell Biol. 1999;78:186-193.
[ Links ]NAGAMUNE K, SIBLEY LD. Comparative Genomic and Phylogenetic Analyses of Calcium Atpases and CalciumRegulated Proteins in Apicomplexa. Mol Biol Evol. 2006;23:1613-1627.
[ Links ]NYALWIDHE J, LINGELBACH K. Proteases and Chaperones Are the Most Abundant Proteins in the Parasitophorous Vacuole of Plasmodium falciparumInfected Erythrocytes. Proteomics. 2006;6:1563-1573.
[ Links ]OSSORIO PN, DUBREMETZ JF, JOINER KA. A Soluble Secretory Protein of the Intracellular Parasite Toxoplasma gondii Associates with the Parasitophorous Vacuole Membrane through Hydrophobic Interactions. J Biol Chem. 1994;269:15350-15357.
[ Links ]PAPAKRIVOS J, NEWBOLD CI, LINGELBACH K. A Potential Novel Mechanism for the Insertion of a Membrane Protein Revealed by a Biochemical Analysis of the Plasmodium falciparum Cytoadherence Molecule Pfemp1. Mol Microbiol. 2005;55:1272-1284.
[ Links ]PRZYBORSKI JM, LANZER M. Protein Transport and Trafficking in Plasmodium falciparumInfected Erythrocytes. Parasitology. 2005;130:373-388.
[ Links ]RAIBAUD A, LUPETTI P, PAUL REL, MERCATI D, BREY PT, SINDEN RE et al. Cryofracture Electron Microscopy of the Ookinete Pellicle of Plasmodium gallinaceum Reveals the Existence of Novel Pores in the Alveolar Membranes. J Struct Biol. 2001;135:47-57.
[ Links ]RAU M, PRESCOTT SW, FERNANDEZ KM, COOKE BM, COWMAN AF. The Role of KAHRP Domains in Knop Formation and Cytoadherence in P. falciparumInfected Human Erythrocytes. Blood. 2006;108:370-378.
[ Links ]SARGEANT TJ, MARTI M, CALER E, CARLTON JM, SIMPSON K, SPEED TP et al. LineageSpecific Expansion of Proteins Exported to Erythrocytes in Malaria Parasites. Genome Biol. 2006;7:R12.
[ Links ]SOLDATI D, FOTH BJ, COWMAN AF. Molecular and Functional Aspects of Parasite Invasion. Trends Parasitol. 2004;20:567-574.
[ Links ]SPYCHER C, RUG M, KLONIS N, FERGUSON DJP, COWMAN AF, BECK HP et al. Genesis of and Trafficking to Maurer's Clefts of Plasmodium falciparumInfected Erythrocytes. Mol Cell Biol. 2006;26:4074-4085.
[ Links ]TONKIN CJ, PEARCE JA, MCFADDEN GI, COWMAN AF. Protein Targeteing to Destinations of the Secretory Pathway in the Malaria Parasite Plasmodium falciparum. Curr Opin Microbiol. 2006;9(4):381-387.
[ Links ]WICKERT H, GOTTLER W, KROHNE G, LANZER M. Maurer's Cleft Organization in the Cytoplasm of Plasmodium falciparumInfected Erythrocytes: New Insights from ThreeDimensional Reconstruction of Serial Ultrathin Sections. Eur J Cell Biol. 2004;83:567-582.
[ Links ]WICKHAM ME, RUG M, RALPH SA, KLONIS N, MCFADDEN GI, TILLEY L et al. Trafficking and Assembly of the Cytoadherence Complex in Plasmodium falciparumInfected Human Erythrocytes. EMBO J. 2001;20:5636-5649.
[ Links ]WIELAND F, HARTER C. Mechanisms of Vesicle Formation: Insights from the Cop System. Curr Opin Cell Biol. 1999;11:440-446.
[ Links ]WISER MF, SARTORELLI AC, PATTON CL. Association of Plasmodium berghei Proteins with the Host Erythrocyte Membrane: Binding to insideout Vesicles. Mol Biochem Parasitol. 1990;38:121-134.
[ Links ]WISER MF, LANNERS HN, BAFFORD RA, FAVALORO JM. A Novel Alternate Secretory Pathway for the Export of Plasmodium Proteins into the Host Erythrocyte. Proc Natl Acad Sci U S A. 1997;94:9108-9113.
[ Links ]WISER MF, GRAB DJ, LANNERS HN. An Alternate Secretory Pathway in Plasmodium: More Questions Than Answers. In: Bock Gr, Cardew G, eds. Transport and Trafficking in the MalariaInfected Erythrocyte. Chichester: John Wiley & Sons, Ltd.; 1999a. p. 199-214.
[ Links ]WISER MF, LANNERS HN, BAFFORD RA. Export of Plasmodium Proteins Via a Novel Secretory Pathway. Parasitol Today. 1999b;15:194-198.
[ Links ]ZHU G, KEITHLY JS. Molecular Analysis of a PType Atpase from Cryptosporidium parvum. Mol Biochem Parasitol. 1997;90:307-316.
[ Links ]ZHU G, KEITHLY JS, PHILIPPE H. What Is the Phylogenetic Position of Cryptosporidium. Int J Sys Evol Biol. 2000;50:1673-1681.
[ Links ]