INTRODUCTION
Bone marrow-derived dendritic cells (DCs) are the most potent antigen presenting cells (APC). These cells are specialized in antigen uptake, processing, transport to lymphoid organs, and antigen presentation to naive and resting T cells. DCs express a unique repertoire of cell-surface molecules including high levels of MHC class I and II, adhesion and costimulatory molecules, all of which participate in the activation of T and B cells. Since DCs are the only APC able to prime naive CD4+ and CD8+ T cells, they play a unique and central role in the development of specific immune responses (Banchereau and Steinman, 1998; Waisman et al., 2017). Due to their functional properties, DCs act as immune sentinels. Therefore, direct or indirect induced modifications of DCs by viral infection could be a strategy to escape from the host immune system. DCs are implicated in the pathogenesis of several viral infections such as measles (Schlender et al., 1996; Grosjean et al., 1997), dengue (Wu et al., 2000), HIV (Knight, 1995; Granelli-Piperno et al., 1998) and HTLV-l infection (Macatonia et al., 1992; Makino et al., 2000).
In the case of Hepatitis C Virus (HCV), it is a significant cause of chronic liver disease (WHO, 2018), one proposed mechanism of viral persistence is the ability of HCV to infect hematopoietic cells (Zehender et al., 1997; Laskus et al., 2000), including DCs (Navas et al., 2002). HCV infection of DCs could impair their functions, thus hampering virus elimination by the host immune system (Kanto et al., 1999; Yi et al., 2015).
Hepatitis C Virus is an enveloped positive-strand RNA virus; it belongs to the Flaviviridae family. Among HCV-encoded proteins, the highly conserved Core protein has been suggested to be responsible for the immunomodulatory properties of HCV (Ríos-Ocampo et al., 2019). A Core is a phosphorylated protein, which consists of two domains: an NH2-terminal domain of highly charged aminoacids (aa) and a COOH-terminal domain of hydrophobic residues. Two different forms of Core protein have been identified with molecular sizes of 21 kDa (p2l) and 23 kDa (p23). p23 (191 aa) is associated with the endoplasmic reticulum, and it is further processed to yield p21 (174 aa) which is the mature form of the Core protein. Although subcellular localization of p21 is predominantly cytoplasmic, it is also found associated with the nuclear membrane (Yasui et al., 1998).
In addition to a structural function (subunit capsid), a regulatory role has been suggested for Core (Fernández-Ponce et al., 2017). Interestingly, HCV Core protein ability to bind to the cytoplasmic domain of several members of tumor necrosis factor receptor family (TNFR-1, LTBR, Fas) was demonstrated suggesting a viral mechanism to disrupt the cytokine cascade and immune functions of the host cells (Matsumoto et al., 1997; Hahn et al., 2000). Furthermore, immune suppression, prolonged viremia, and increased mortality were described in mice infected with a recombinant Vaccinia virus expressing HCV structural proteins (Large et al., 1999).
Recombinant viral vectors expressing the Core protein and allowing its transfer into DCs could be useful tools for the analysis of the properties of this protein. Adenovirus (Dietz and Vuk-Pavlovic, 1998), Vaccinia Virus (Di Nicola et al., 1998), Avipoxvirus (Brown et al., 1999) and Retrovirus vectors (Specht et al., 1997) have been used to transfer genes into human DCs.
Semliki Forest Virus (SFV) is a mosquito-borne positive RNA virus belonging to the Togaviridae family, genus Alphavirus. There are several features of Alphaviruses that make them attractive candidates as vectors for the expression of heterologous proteins: broad host range, small genome size, RNA replication in various cell types and viral replicase which drives efficient RNA replication and transcription, resulting in a high level of heterologous protein synthesis (Henao et al., 2007).
The SFV has been reported to be able to transfer genes into various mammalian cell types (Yepes et al., 2006; Henao et al., 2007). The SFV expression system is based on a genomic SFV cDNA inserted into a plasmid under the control of the prokaryotic Sp6 promoter. The SFV structural genes have been deleted from the vector in order to allow insertion of a heterologous gene. Production of recombinant SFV particles is based on the co-transfection of cells with three different RNAs: i) the vector replicon which contains the genes coding for the nonstructural proteins nsP1 to nsP4 (transcriptase complex), the sub-genomic promoter followed by the heterologous gene, as well as the 5' and 3' ends of the genome which are required for replication. ii) an RNA coding for the capsid protein and iii) an RNA encoding the envelope proteins (Liljestrõm and Garoff, 1991). A strategy based on the expression of structural genes from two separate RNAs increases the biosafety of recombinant SFV vectors (Berglund et al., 1999).
The study aimed to express the HCV Core protein in human monocyte-derived DCs using an SFV vector and then analyze the effects of its expression on DCs functions.
MATERIAL AND METHODS
Cells
BHK-21 cells were grown in BHK medium (Gibco, Life Technologies, Scotland) Supplemented with 10 % tryptose phosphate broth, gentamicin 5 μg/mL (Thermo Fisher Scientific8, USA) and 10 % heat-inactivated fetal calf serum (FCS) (Valbiotech, France). Madin-Darby bovine kidney (MDBK) cells were maintained in minimal essential medium (MEM) (Thermo Fisher Scientific®, USA) supplemented with 10 % FCS (Valbiotech, France).
Stably transfected Hep-2 cells expressing human MxA protein, obtained from Institute of Medical Virology, University ofZurich, were maintained in Dulbecco's modified MEM (Thermo Fisher Scientific®, USA) containing 10 % FCS, Penicillin/Streptomycin and 500 μg of G418 per ml.
DCs were generated from human PBMC from healthy donors (Sallusto and Lanzavecchia, 2018). Monocytes were isolated by continuous flow centrifugation leukopheresis and counterflow elutriation (Faradji et al., 1994) and cultured in RPMI-1640 medium (Thermo Fisher Scientific®, USA) supplemented with 5 % heat-inactivated FCS, Penicillin 100 IU/ml-Streptomycin 100 UG/ml (Gibco), 1000 U/ml hrGM-CSF (PeproTech lnc, USA) and 200 U/ml rhIL-4 (Genzyme-Corporation, USA). On day seven, non-adherent cells corresponding to immature DCs (iDCs) were collected and analyzed by FACScan. Mature DCs (mDCs) were obtained by further culture of iDCs by co-culture with mitomycin C-treated CD40-L-transfected fibroblastic L cells (provided by Schering-Plough, Laboratory for Immunological Research, Dardilly, France) at a 1:4 ratio for 48 hours. Cells were harvested and analyzed by FACScan.
Antibodies
DCs were phenotyped with the panel of monoclonal antibodies (mAb) listed below and analyzed by Flow cytometry (FACScan, Becton Dickinson, USA). Data analysis was performed using the CELL Quest software (Becton Dickinson, USA). Monoclonal antibodies (mAb) used included anti-CD14-fluorescein isothiocyanate (FITC), anti-CD1a-Phycoerythrin (PE), anti-CD40-PE, anti-HLA-DR-FITC, anti-CD80 (B7.1)-FITC and anti-CD83-PE from lmmunotech (Marseille, France) and anti-CD86 (B7.2)-PE (Pharmingen, USA).
Human monoclonal antibody (mAb) B12.F8 specific for HCV core protein was generously provided by Dr. Mario Mondelli (Istituto di Clinica delle Malettie Infettive, University of Pavia, Italy). This mAb B12.F8 was obtained from a cell line established from peripheral blood mononuclear cells of an infected patient. Polyclonal mouse anti-human MxA protein clone 2Cl2 was obtained from Institute of Medical Virology, University of Zurich, Zurich, Switzerland.
Plasmids
Plasmids pSFV1, pSFV-helper-S2, and pSFV-helper-C were kindly supplied by Dr. Peter Liljestrom (Microbiology and Tumorbiology Center, Karolinska lnstitutet, Stockholm, Sweden). The sequence encoding the HCV Core protein (amino acids 1-191) was amplified from a vector containing a full-length cDNA clone of the HCV-H77 strain (Kolykhalov etal., 1997) provided by Dr. Charles Rice (Washington University School of Medicine, St Louis, MO, USA) and cloned into the BamHI site of pSFV1 yielding pSFV1-Core.
Recombinant Semliki Forest Viruses
In vitro transcription of RNA from linearized plasmid vectors pSFV1-Core, pSFV-helper-S2 and pSFV-helper-C was performed in the presence of m7G(5')ppp(5')G as a capping analog using Machine Sp6 large scale in vitro transcription kit (Ambion Inc, Austin, TX) according to the manufacturer's instructions. RNA was synthesized at 37 °C for two hours in 20 μ! reaction containing 1 μg of Spel-linearized plasmid DNA, transcription buffer (including DTT), ribonucleotide mix (NTP/Cap) and Sp6 enzyme mix (including RNAse inhibitor). The DNA template was removed by DNAse l treatment followed by RNA purification (Qiagen, Hilden, Germany). Synthetic RNA was analyzed by running on agarose-formaldehyde gel under denaturing conditions.
To confirm HCV Core expression from recombinant SFV RNA in cell cultures, BHK-21 cells were transfected with RNA SFV1-Core using the Effectene Lipofection kit (Qiagen, USA). Briefly, the day before transfection, 4x106 cells were seeded on 100 mm-diameter Petri dishes to provide a confluence of 80 % on the day of transfection. Transfection was performed as follows: 1 μg purified RNA pSFV1-Core was diluted in 150 μl ECL buffer enhancer was then added and the mix was incubated at room temperature for 5 min. 20 μl Effectene was added to the RNA solution which was subsequently incubated at room temperature for 10 min. The medium was removed from the Petri dishes; cells were washed with PBS and incubated with Effectene-RNA complex in the presence of 800 μl fresh complete medium. 6 hours after transfection, 4 ml complete medium was added to the cells. After 48 hours of incubation, transfection efficiency was determined by indirect immunofluorescence using an Axiovert 135 fluorescence microscope (Carl Zeiss, Germany).
Packaging of recombinant RNA encoding HCV Core into rSFV particles was performed as described previously (Liljeström and Garoff, 1991). 10 μg of in vitro transcribed RNA molecules from pSFV1-Core, pSFV-helper-S2 and pSFV-helper-C were co-transfected into BHK-21 cells using Effectene reagents as described above. After 24 hours of incubation, culture supernatants containing recombinant viruses were harvested. Indirect immunofluorescence analysis of infected BHK cells was performed in order to determine the titre of the recombinant virus stock (Liljestrõm and Garoff, 2001).
Immunofluorescence
After three washes with PBS, cell monolayers were fixed (3 % paraformaldehyde) and permeabilized (0.5 % Triton X-100, Sigma, USA). Human monoclonal anti-HCV Core mAb B12.F8 was diluted 1:25 in PBS containing 1 % bovine serum albumin (BSA) (Sigma, USA). FITC conjugated goat anti-human 1gG1 (Sigma, USA) diluted 1:50 in PBS 1 % BSA and Evans blue was used as the secondary antibody. Immunofluorescence was visualized under an Axiovert 135 fluorescence microscope (Carl Zeiss, Germany).
Gene transduction into DCs
Immature and mature DCs were infected with rSFV1-Core vector at the multiplicity of infection (MOI) of 10 and 20. DCs have incubated in MEM supplemented with 2 % FCS at 37 °C. After 1-hour incubation, recombinant virus particles were removed by centrifugation and replaced with fresh RPMI supplemented with GM-CSF and IL-4. Cells were further incubated for 96 hours.
HCV Core Protein detection
DCs or BHK-21 cells (1x106 cells) were harvested at 12, 24, 36, 48, and 72 hours after transduction with rSFV1-Core. Cell pellets were lysed in RIPA buffer (150 mM NaCl, 1 % NP4O, 50 mM Tris-HCI pH 7.5, 0.1 % SDS, 1 mM Phenyl methyl sulfonyl fluoride and 10 μg/ml Aprotinin) and incubated for 10 min at 4 °C. To remove cell debris, the extracts were centrifugated at 1050 g for 10 min; proteins were quantified using the Bradford assay. Five to 35 μl lysate was loaded on a 10 % Sodium dodecyl sulfate (SDS) polyacrylamide gel (PAGE) under reducing conditions. After migration, proteins were transferred onto a nitrocellulose membrane (Hybond-P; Amersham Pharmacia Biotech, Uppsala, Sweden). The membranes were blocked with 3 % BSA in PBS/0.1 % Tween 20 (Sigma, USA) overnight at 4 °C, incubated with anti-HCV Core mAb B12.F8 diluted 1:25. They were then incubated for 1-hour with a biotinylated anti-human IgG diluted 1:1000 and finally for 30 minutes with horseradish peroxidase-conjugated streptavidin diluted 1:1000 (Amersham Biosciences, USA). Proteins were visualized by chemiluminescence using the ECL system (Amersham Biosciences, USA) according to the manufacturer's instructions.
MxA protein detection
DCs or BHK-21 cells (1x106) were harvested at 24, 48, 72 hours after transduction with rSFV-Core. Cell extraction, protein separation on 10 % SDS-PAGE, transfer on to nitrocellulose membranes, immunostaining and visualization by chemiluminescence were done as described above. The MxA protein was detected using mouse polyclonal anti-MxA antibody 2C12. Stably transfected Hep-2 cells expressing human MxA protein and BHK-21 cells were included as a positive and negative control, respectively.
IFN- α dosage
Quantitative determination of IFN-a was performed in cell-free supernatants of DCs cultures using an in-house biological assay (Lebon et al., 1979). Briefly, supernatants were diluted two-fold in microtiter plates in a volume of 50 μl MEM supplemented with 10 % FCS. MDBK cells (104 cells/0.1 ml), which are sensitive to type 1 human interferon, were added to each well. After 18 hours incubation at 37 °C, cells were infected with Vesicular Stomatitis Virus (VSV) (30 plaque-forming units/0.1 ml/well). Inhibition of VSV multiplication was observed 24 hours later, and the interferon titres were determined by comparison with dilutions of an IFN standard.
RESULTS
In order to express the HCV Core protein into human monocyte-derived DCs using recombinant SFV and therefore evaluate the effect of this protein on DCs functions, the genomic region encoding HCV Core protein (aa 1-191) was cloned into SFV plasmid vector pSFV1 under the control of the subgenomic SFV promoter.
We first checked the expression of the HCV Core protein from pSFV1-Core-derived in vitro transcribed RNA after transfection into BHK-21 cells using the Effectene lipofection kit. Cells were tested for Core expression at 48 hours after transfection by indirect immunofluorescence with anti-HCV Core mAb B12.F8. As shown in figure 1a, HCV Core protein expression was readily observed in the cytoplasm of transfected BHK-21 cells.
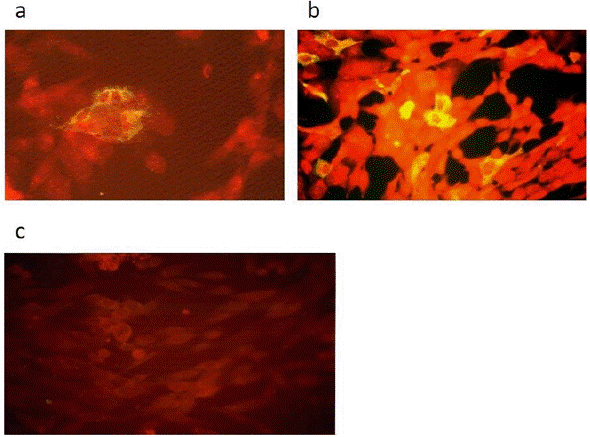
Figure 1 Detection ofHepatitis C Virus Core protein in BHK-21 cells by indirect immunofluorescence. Cells were fixed and incubated with human monoclonal anti-HCV Core antibody B12.F8 (provided by Dr. Mario Mondelli, University of Pavia) and visualized by indirect immunofluorescence (Axiovert 135 fluorescence Microscope, objective 40x). These micrographs show representative fields from the experiments. (a) BHK-21 cells transfected with pSFV1-Core derived in vitro transcribed RNA, (b) BHK-21 cells infected with rSFV1-Core recombinant particles at a MOI 0.1 and (c) BHK-21 control cells.
Subsequently, we investigated HCV Core protein expression from recombinant SFV particles in BHK-21 cells. RNA transcribed from the recombinant pSFV1-Core plasmid was packaged into SFV particles by cotransfection with SFV-helper-S2 and pSFV-helper-C in vitro transcribed RNAs into BHK-21 cells using the Effectene lipofection kit. After 24 hours, culture supernatants were harvested, and the resulting virus stock was used to infect BHK cells at a MOI of 0.1. Figure 1b shows the immunostaining analysis of infected cells with anti-HCV Core mAb B12.F8 performed at 48 hours post transduction. Also, cells were harvested at 12, 24, 36, 48, and 72 hours postinfection; expression of the HCV Core protein was analyzed at each time point by Western blot. Figure 2 shows a band of 21 kDa revealed with mAb B12.F8 (specific for residues 32 to 46 of HCV Core protein) at 12 hours after rSFV infection. HCV Core expression reached a maximum level after 36 hours and remained stable until 72-hours postinfection. A second band of 23 kDa, as reported previously (Yasui et al., 1998), was detected between 36 and 48 hours of infection.
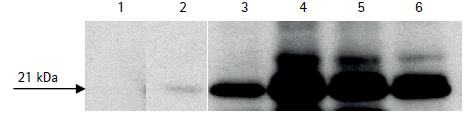
Figure 2 Hepatitis C Virus Core protein expression in BHK-21 cells after infection using recombinant SFV. Cells lysates were separated in 10 % SDS-PAGE, then transferred onto a nitrocellulose membrane and immunostained with human antibody anti-HCV Core B12.F8 (provided by Dr. Mario Mondelli, University of Pavia). The bands were visualized by chemiluminescence. Western blot analysis of mock-infected BHK-21 cells (lane 1) and BHK-21 cells infected using rSFV particles at 12, 24, 36, 48 and 72 hours after infection (lanes 2-6).
We subsequently performed the transduction assays using rSFV into human monocyte-derived DCs obtained as described in Material and Methods. After seven days of culture, a homogeneous nonadherent cell population with typical immature DCs morphology was obtained. Flow cytometry analysis confirmed the immature DCs phenotype: CD1a+, CD14-, CD40+, HLA-DR+ and costimulatory molecules CD83- and CD86low. Mature DCs generated by co-culture with mitomycin C-treated CD40-L-transfected fibroblastic L cells expressed high levels of HLA molecules, CD40, CD83 and CD86 (Fig. 3).
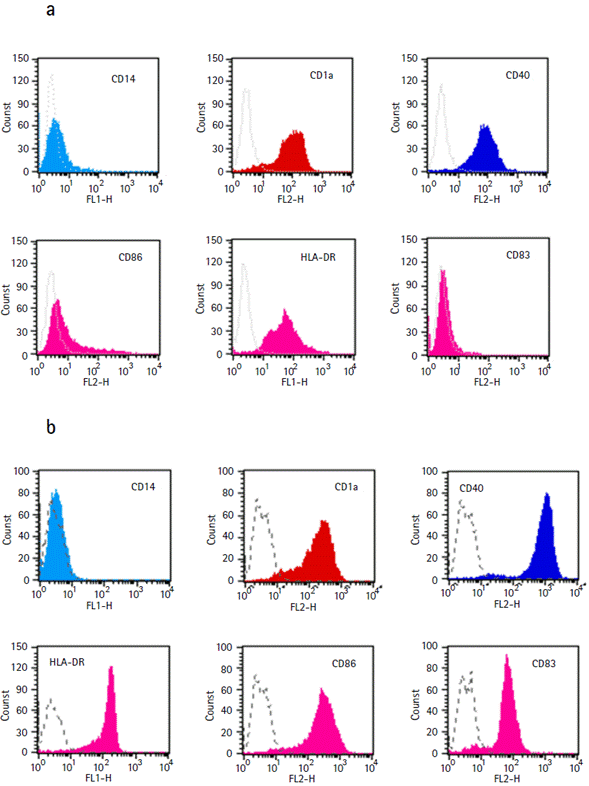
Figure 3 Expression of Dendritic Cells surface markers. FACScan (Becton Dickinson, USA) analysis of monocyte-derived immature Dendritic Cells: CD1a+, CD14-, CD40+, HLA-DR+, costimulatory molecules CD83- and CD86low (a) and mature Dendritic Cells: HLA-DR high, CD40 high, CD83+ and CD86 high (b).
DCs, immature and mature, were transduced with rSFV particles coding for the HCV Core protein at MOl of 10 and 20. Cells were harvested at 24, 48 and 72 hours postinfection for WB analysis using anti-HCV Core B12.F8 mAb. No Core protein expression could be detected in any DCs extracts (data not shown), even if the MOI was 100 and 200 times higher than the MOI used for BHK-21 cells. This result was confirmed in several experiments using DCs derived from human monocytes obtained from different donors.
We hypothesized that the absence of HCV Core expression in DCs following infection with the rSFV-Core might be due to the interference of the interferon system with SFV replication. Type I IFN quantification was performed using biological assay in cell free supernatants of DCs cultures harvested at 24, 48 and 72 hours after transduction. Type I IFN expression was induced in iDCs and mDCs transduced with rSFV as compared with mock-infected DCs. IFN was detected in iDCs supernatant at 24 hours postinfection and reached a maximum level at 48 hours. LPS-treated DCs showed a higher level of IFN production (> 400 UI) than iDCs as early as 24 hours postinfection. After 24 hours, IFN level decreased but remained high until 72 hours postinfection (Fig 4).
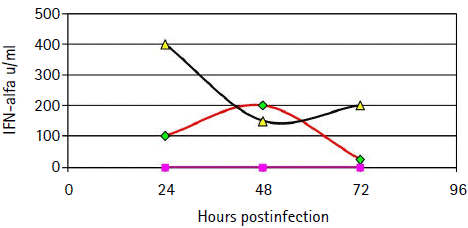
Figure 4 Time course oftype I IFN production in human monocyte-derived Dendritic Cells following rSFV infection. INF-α quantification was performed in cell free supernatants of mock-infected Dendritic Cells (∆), rSFV-infected immature Dendritic Cells (□) and rSFV-infected LPS-treated Dendritic Cells (▲) using an in-house biological assay. Briefly, supernatants were diluted two-fold in microtiter plates, before addition of MDBK cells. After 18 hours incubation, cells were infected with Vesicular Stomatitis Virus. Inhibition of Vesicular Stomatitis Virus multiplication was determined at 24 hours after Vesicular Stomatitis Virus infection.
The antiviral effect of type I IFN is mediated by several mechanisms. Since the IFN-inducible human MxA protein was previously reported to interfere with viral replication (Landis et al., 1998), we examined the time course of MxA protein expression in iDCs and mDCs postinfection. Cells were harvested at 24, 48 and 72 hours postinfection. Expression of the MxA protein at each time point was analyzed by WB with the polyclonal anti-MxA 2Cl2 antibody (Fig 5). High expression of MxA was induced in iDCs at 24 hours after SFV infection and remained stable until 72 hours postinfection while only a faint expression was detected in mock-infected DCs. MxA protein expression was also detected SFV-infected LPS-treated DCs (data not shown).
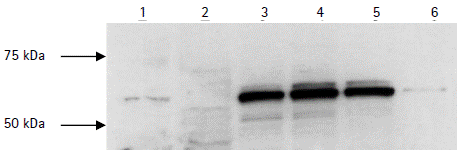
Figure 5 Western Blot analysis of MxA upregulation in immature Dendritic Cells following rSFV infection. Samples were separated on 10 % SDS-PAGE, transferred to a nitrocellulose membrane and immunostained with mouse polyclonal anti-MxA 2Cl2 antibody. The bands were visualized by chemiluminescence. Lane 1: Hep-2 cells expressing human MxA protein. Lane 2: BHK-21 cells. Lanes 3-5: immature DCs at 24, 48 and 72 hours following rSFV infection. Lane 6: mock-infected immature Dendritic Cells.
DISCUSSION
In this study, the expression of the HCV Core protein was observed in BHK-21 cells using an SFV vector. Two different forms of HCV Core protein (p21 and p23) were detected in transducted BHK-21 (Yasui et al., 1998). Moreover, a cytopathic effect was observed at 3-4 days after transduction, as previously described. However, we failed to detect any expression of HCV Core protein in both immature DCs and mature DCs using the same rSFV vector, even with MOI 100 and 200 times higher than the MOI for BHK-21 cells. Significant upregulation of IFN-a production was observed in supernatants of immature and mature human monocyte-derived DCs following rSFV transduction. Moreover, a strong induction of the MxA protein expression was detected in immature DCs. Morphology and viability were similar in mock-infected DCs and transducted DCs cultures suggesting resistance of the cells to the cytopathic effect of the virus. These findings suggest that, although HCV Core protein expression was not detected, activation of DCs and therefore upregulation of type I IFN system including IFN-inducible MxA proteins occurred following infection with the recombinant SFV vector.
It has been demonstrated that activation of DCs by viral infection and the presence of double-stranded RNA leads to upregulation of MHC class I and II, as well as adhesion and costimulatory molecules. Furthermore, activated DCs exhibit resistance to the cytopathic effect induced by infection with Influenza virus. This resistance is mediated by the production of type I IFN, which in turn induces MxA protein expression (Cella et al., 1999).
Cella et al., (1999) have demonstrated that expression of MxA by DCs in response to specific stimuli is correlated to their capacity to produce type l IFN (Cella et al., 1999). The molecular mechanism of Mx proteins action remains unclear, but their GTPase activity appears to be essential for their antiviral function probably by inhibiting viral Polymerase activity. Previous studies have demonstrated MxA protein ability to inhibit replication of several negative-strand RNA viruses (Pavlovic et al., 1990; Schneider-Schaulies et al., 1994; Frese et al., 1996; Zhao et al., 1996). It was demonstrated that the antiviral spectrum of MxA also included a positive-strand virus, SFV (Landis et al., 1998). Indeed, strong reduction of SFV protein level of expression and inhibition of viral mRNA synthesis was observed in infected Hep-2 cells which express MxA suggesting blockade of SFV replication (Landis et al., 1998). Furthermore, using the SFV replicon system, it was demonstrated that SFV nonstructural proteins are the targets of the MxA protein (Landis et al., 1998).
Moreover, significant upregulation of murine Mx-l and Mx-2 mRNAs, corresponding to two genes regulated by type I IFN, was reported in bone-marrow DCs after both infections with SFV particles and transfection with rSFV RNA replicon (Berglund et al., 1999). Although no heterologous protein (GFP) expression was observed, intracellular negative-strand RNA intermediates of rSFV were detected up to 48 hours postinfection. These findings demonstrate that although GFP could not be detected, DCs activation occurs likely by the initiation of the SFV replication cycle as observed in our study. These data corroborate a study carried out in mice, which showed infection of Langerhans cells with SFV resulted in upregulation of class I MHC antigens expression, consistent with DCs maturation and activation. This activation allowed subsequently the induction of a specific immune response (Johnston et al., 2000). In contrast, Osterroth et al., (2000) have observed expression of heterologous protein in DCs infected with rSFV. Although the reasons for this discrepancy are not fully understood, we suggest that maturation state of DCs and/or high multiplicity of infection as responsible for the heterologous protein expression (Osterroth et al., 2000).
Studies concerning novel vaccinal strategies using rSFV have demonstrated induction of strong humoral and cellular immune response after immunization (Zhou et al., 1994; 1995 ). Low heterologous protein synthesis level in DCs could be overcome by the uptake and presentation of exogenous material by these cells. Indeed, several studies have shown that DCs can efficiently take up apoptotic material from dying cells expressing heterologous proteins. This constitutes an alternative pathway for antigen presentation to T cells and subsequent development of a cellular immune response. However, only weak induction of specific CTL for HCV Core following immunization with rSFV particles was reported recently (Vidalin et al., 2000).
CONCLUSION
The results of this study demonstrated the transduction of human monocyte-derived DCs using rSFV; however, the expression of HCV Core protein was not detected even in conditions of MOI of 20. On the other side, the transduction induced the activation of DCs resulting in upregulation of IFN-inducible MxA protein which is involved in blocking SFV replication but reducing heterologous protein expression. Further studies are necessary to improve transduction into human DCs using Alphavirus vectors.