Services on Demand
Journal
Article
Indicators
-
Cited by SciELO
-
Access statistics
Related links
-
Cited by Google
-
Similars in SciELO
-
Similars in Google
Share
Ingeniería e Investigación
Print version ISSN 0120-5609
Ing. Investig. vol.28 no.2 Bogotá May/Aug. 2008
Nayibe Guerrero1, Anyela Guzmán2, Diana Milena Montoya3, Carlos Londoño4 and Farid Chejne Janna5
1 Ingeniera química, Universidad Nacional de Colombia. Magíster, en Ingeniería de Materiales y Procesos, Universidad Nacional de Colombia. Ingeniera, INDISA S.A. nnguerre@unalmed.edu.co
2 Ingeniera química, Universidad Nacional de Colombia. Ingeniera, LAVADO TECNICO S.A. amguzman@unalmed.edu.co
3 Ingeniera química, Universidad Nacional de Colombia. Ingeniera, TERMILENO S.A. dmmontoy@gmail.com
4 Ingeniero químico, Universidad Nacional de Colombia. clondono@unalmed.edu.co
5 Físico, Universidad de Antioquia, Colombia. Ingeniero mecánico, Universidad Pontificia Bolivariana, Colombia. Profesor, Universidad Nacional de Colombia, sede Medellín. fchejne@unalmed.edu.co
ABSTRACT
The Flynn-Wall-Ozawa method was used for analysing coal-solid waste blend reactivity in an oxidising atmosphere. The presence of biomass strongly affected coal combustion kinetics when the blend contained more than 30% of it. Activation energy values (evaluated by different blends) were 28.7495 kJ/mol for 0% biomass, 31.3915 kJ/mol for 30% biomass, 39.0365 kJ/mol for 50% biomass, 102.431 kJ/mol for 70% biomass and 107.8075 kJ/mol for 100% biomass; these values were close to those reported in the literature. First-order kinetics correlated the data very well for the 100% coal sample and the blend having 30% biomass and 70% coal. Eighth-order kinetics were more suitable for correlating the experimental data for the 70% biomass-30% coal blend and the 100% biomass sample. Combustion was done without previous pyrolysis of the blends; however segregation of phenomena could be appreciated. This seems to indicate that combustion and devolatilisation are independent processes which should be taken into account when building equipment using these kinds of blend.
Keywords: co-combustion, coal, biomass, reactivity, thermal analysis.
RESUMEN
La reactividad de mezclas de residuos sólidos y carbón en atmósfera oxidante fue analizada usando el método de Flynn Wall Ozawa. La cinética de la combustión es fuertemente afectada por la presencia de biomasa, cuando la relación en la mezcla es superior al 30%. Los valores de la energía de activación evaluados para diferentes mezclas fueron: 28.7495 kJ/mol para 0% de biomasa, 31.3915 kJ/mol para 30% de biomasa, 39.0365 kJ/mol para 50% de biomasa, 102.431 kJ/mol para 70% de biomasa y 107.8075 kJ/mol para 100% de biomasa. Estos valores son cercanos a los reportados en la literatura. Se encontró también que para la muestra de carbón al 100% y la mezcla de 30% biomasa y 70% de carbón una cinética de primer orden correlaciona muy bien los datos; mientras que para la mezcla de 70% biomasa 30% carbón y la muestra de 100% biomasa, una cinética de orden 8 es más adecuada para correlacionar los datos experimentales. La combustión se hizo sin la pirólisis previa de las muestras; sin embargo, es posible apreciar una división del fenómeno. Esto parece indicar que la combustión y la devolatilización son procesos independientes, lo cual es importante en la construcción de equipos para este tipo de mezclas.
Palabras clave: co-combustión, carbón, biomasa, reactividad, análisis térmico.
Recibido: enero 22 de 2008
Aceptado: junio 23 de 2008
Introduction
Solid waste from human activities has caused environmental deterioration and poor health for communities living around sanitary landfills. Governments and the scientific community have thus proposed several alternatives for resolving this problem, such as the direct controlled combustion of organic waste solids mixed with coal as energy source (Defeche et al., 1980).
Controlled combustion of organic waste solids is a method which is widely used around the world as these residuals are transformed into inert material (ashes) and hot gases; a 70% reduction in weight and 80%-90% in total biomass volume may be achieved (Folgueras et al., 2003). A large amount of pollutants emitted by traditional incineration systems such as flying ash, sulphates, chlorhydric acid and substances such as dioxins and furans have made their use become restricted in many countries, especially European ones.
Coal and different types of biomass blends, like urban, forest and industrial solid waste, have being researched as being clean and economic energy alternatives allowing the substitution of traditional fossil fuel, thereby mitigating both the environmental impact brought by its use and partially resolving waste management. This blend reduces polluting gases such as nitrogen oxides and sulphur (Romero, 2002), improving combustion efficiency at the same time (Gayana et al., 2004).
Folgueras et al., (2003) carried out TGA and DSC analysis of coal and sewage sludge co-combustion. They studied bituminous coal combustion, three types of sewage sludge and their blends, in static air atmosphere using a 10°C/min heating rate constant from room temperature to 800ºC. The process was considered as a series of consecutive first-order reactions in this study using Arrhenius law for kinetic constant determination.
Biagini et al., (2002) studied the devolatilisation behaviour of several coals, biomasses and blends of rhem through thermo-gravimetrical analysis in a nitrogen atmosphere using isothermal and non-isothermal conditions. First-order kinetic mode was assumed for one reaction. The distribution of activation energy model was also used; thermal decomposition is considered to be an independent chemical reaction series in this model.
Calvo et al., (2004) studied rice-husk reactivity in N2, O2 atmospheres and blends of them by using TG analysis. They observed three main stages when they worked with the two gases. They used a first reaction model for finding out kinetics parameters and the Coast Redfern Model for determining the reaction order.
Vuthaluru et al., (2004) also used thermogravimetrical analysis for studying wood residuals with coal blend kinetics. It was found that there were no interactions in blends having a low proportion of biomass and it was proven that a first-order model reaction was suitable for correlating blend kinetics. This work was aimed at studying coal-biomass blend behaviour by using TG, DTG and DSC analysis and calculating kinetic parameters for combustion process, frequency factor A and activation energy E.
Experimental method
Sample preparation
Domestic solid waste from the metropolitan area of Valle de Aburrá in Medellín, Colombia, was gathered then dried in a forced convection oven at 50ºC and 60ºC. It was then milled until reaching mesh size 200 (particle diameter less than 0.074 mm) and then mixed with the same size coal from the Amagá region.
Characterisation
Tables 1 and 2 show proximate and ultimate analysis for coal and biomass.
Blend preparation
Five different coalbiomass blends were prepared with the following size ratios: 0% biomass-100%coal, 30% biomass-70% coal, 50% biomass-50%coal, 70% biomass-30% coal and 100% biomass-0%coal.
Combustion
Every blend was heated from 30ºC to 900 ºC in an oxidising atmosphere using a pure oxygen stream in a thermo-gravimetrical analyser (STA 409 CD Nestzch). Three heating rates were used: 5, 10 and 20ºC/min. An additional assay used a 100% biomass-0% coal sample blend in an inert atmosphere at 10°C/min as heating rate to observe behaviour during pyrolysis.
Kinetic model
Decomposition may happen when a sample is being heated; moreover, a kinetic study must be carried out for determining the kinetic parameters characterising such change.
The conversion is defined as:

The kinetics of any solid materials decomposition can be represented in the following way (Kristiansen et al., 1996):

and its kinetics can be described as:

Temperature dependence was described by Arrhenius equation:

Equations 3 and 4 were obtained in non-isothermal conditions, using the Chain rule:


where: β = dT / dt is the heating rate.
The model proposed by FlynnWall Ozawa was used for finding the kinetic parameters (Fraga et al., 2001); this method has the advantage that activation energy can be calculated without making any assumption about the reaction model. This method also allows activation energy to be obtained as a function of temperature and /or conversion (Vyazovkin et al., 1999).
An attempt was made to integrate as follows in FlynnWall Ozawas model:
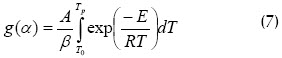
α0 = 0 was assumed when temperature was low and no reaction between T = 0 and T0. Doyles approach (Johannes et al. 2000, Sima et al., 2005) was used for obtaining the following expression:

A first-order reaction was supposed for finding A in the following expression:

The following was obtained after algebraic manipulation:

Several f(α) should be supposed and which function and frequency factor best correlate the data should be statistically determined (10).
Results and Discussion
It was observed from proximate and ultimate analysis that biomass had a greater amount of volatile matter than coal; it also had a lower high-heat value. These conditions greatly influence designing a combustion chamber. It had also to be taken into account that biomass was dried before any treatment because it had around 60% humidity on a wet base.
Assays carried out with 100% biomass using a 10°C/min heating rate with TGA and DTG equipment led to obtaining a devolatilisation profile (Figure 2). An exothermic process can be observed from the DSC curve when the biomass was subjected to an inert atmosphere, corresponding to biomass devolatilisation.
An additional peak in this process could be noted when the lost weight profile in an inert atmosphere was compared to that in an oxidising one. The first peak (around 260ºC) coincided with devolatilisation (as observed in the DSC profile). The first peak was presented in both atmospheres at the same temperature; total mass loss was practically the same. The second peak was due to the combustion process (from 320ºC).
Figure 1 shows that devolatilisation and combustion during biomass burn-off in an oxidising atmosphere are separate processes, maybe due to different kinds of volatile material having an ignition point higher than 320ºC.
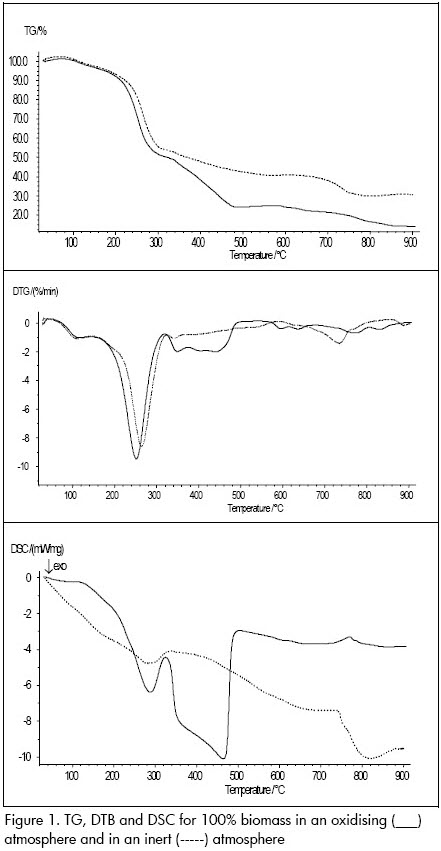
It can be noticed that the curves corresponding to blends having more biomass content (see Figure 2) presented greater bigger peak temperature values (a point where any change inside a sample was occurring); this could have happened due to complexity in biomass composition compared to the composition of the coal.
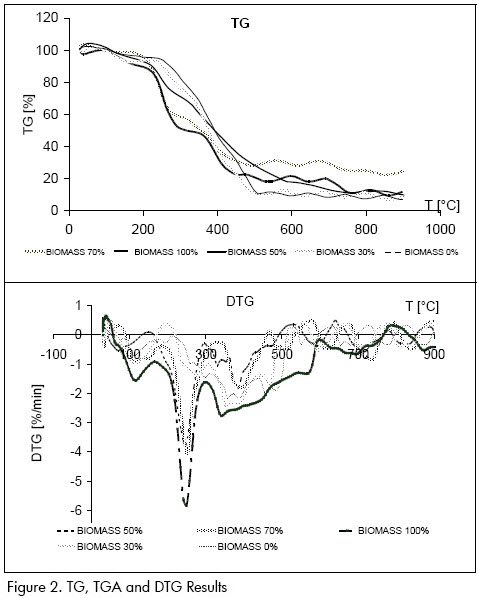
Displacements were observed toward more weight losses when biomass content was increased in the TG curve, being attributed to larger volatile material biomass content and that volatile material was released from biomass in less time than from coal. A displacement of peaks could be observed toward smaller temperatures in DTG curves and therefore at shorter times, this being attributed to thermal decomposition first beginning in blends having more biomass content.
Although the experiments were carried out in an atmosphere oxidising, two peaks defined in the curves having greater bio-mass content (above 30%) could be clearly noticed when comparing the results obtained with those reported in the literature and with the assay carried out in an inert nitrogen atmosphere for the 100% biomass-0% coal blend. One of them (200ºC-350ºC) could have been due to the volatilisation stage while the other one (400ºC-600ºC) could have been attributed to the blends combustion stage which appeared in the assay with O2.
Only a thermal event was observed for blends having 0%-30% biomass content (i.e. a complete combustion stage). This was more exothermal than the largest biomass proportion (Figure 3c: DSC profile). Similar behaviour was observed to the coal in blends having a low biomass ratio; it could be said that the behaviour of coal was rarely modified by adding small amounts of biomass (up to 30%).
Changing the heating rate did not present changes in the blends overall behaviour since they conserved the same tendency in thermal analysis curves for 5ºC/min, 10ºC/min and 20ºC/min rates. This factors effect on analysis was that the exothermal peaks moved toward higher temperatures with increased heating rate in the case of DTG and DSC while this factor had no clear influence on TG. However, it should be noted that the 10°C/min rate allowed the events being the object of our study to be observed more clearly (Figure 3).
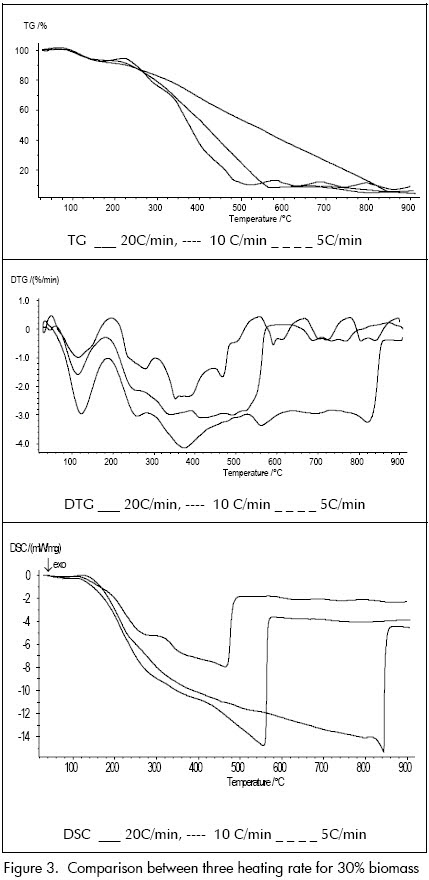
The activation energy given from Flynn-WallOzawas method was given by taking the slope of each straight line shown in Figure 4. This figure shows that although the lines are not completely parallel (due to the amount of reactions), activation energy was constant and almost the same for each conversion. The latter did not happen when biomass ratio exceeded 50% where two clearly differentiated areas appeared (respectively corresponding to volatilisation and combustion).
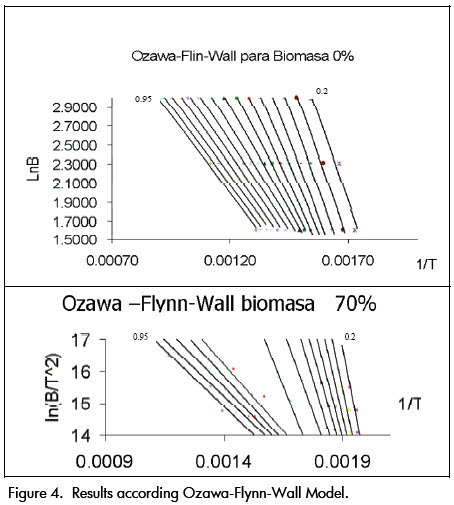
The values obtained for activation energy, evaluated using different blends, were 28.7495 kJ/mol for 0% biomass, 31.3915 kJ/mol for 30% biomass, 39.0365 kJ/mol for biomass 50%, 102.431 kJ/mol for 70% biomass and 107.8075 kJ/mol for 100% biomass. These values were close to those reported in the literature.
Equation (10) was used for calculating the frequency factor, finding that first-order kinetics correlated the data very well for the 100% coal sample and 30% biomass-70% coal blend, while eighth-order kinetics correlated the data better for 70% biomass-30% coal blends and the 100% biomass sample (see Figure 5).
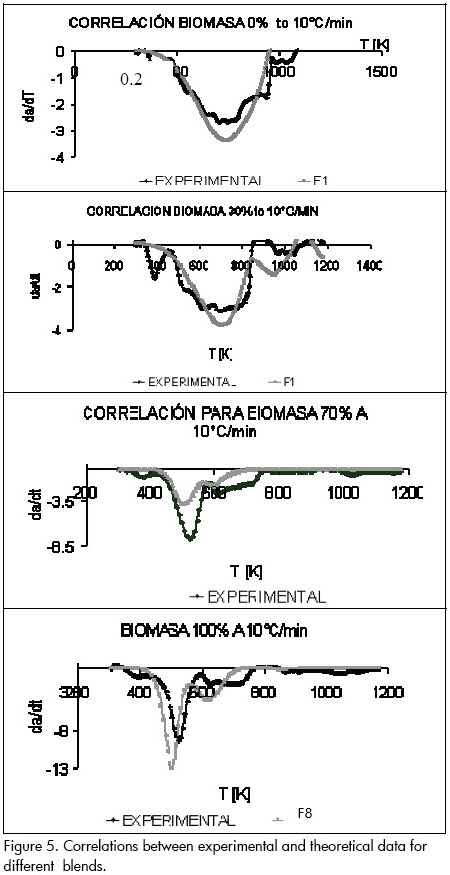
Conclusions
The FlynnWall Ozawa method was used for evaluating the kinetic parameters of biomass and coal blends during combustion, leading to successful results. Biomass in the blends led to segregation: devolatilisation was independent of combustion.
Adding low proportions of biomass to coal did not reveal interactions modifying coal combustion behaviour. However, combustion and devolatilisation were independent processes for blends having more than 30% biomass. The latter could cause a drop in industrial burners energy efficiency if it were not possible to use additional combustion air to burn-off the volatile material.
Coal-solid waste burner design should consider devolatilisation and combustion separately. A designer must consider a short time-scale for completing the overall process, one time-scale for completing the combustion of all volatile material released first and another time-scale for pyrolysed material. This means that larger combustion chambers must be built for solid waste or that mixed with coal for others which only use coal.
Nomenclature
A pre-exponential factor
E activation energy
m, mass in time t
mi initial mass
mf final mass
α conversion
f (α) reaction model
t time
T temperature
Acknowledgments
This work was sponsored by Universidad Nacional de Colombias Mining Faculty Energy Institute. The authors wish to thank Professor Fanor Mondragón from the Universidad de Antioquia in Medellín, Colombia, for his invaluable input and professor Marco Márquez for his guidance regarding the thermal analysis technique.
References
Biagini, E., Lippi F., Petarca L., Tognotti, L., Devolatilization rate of biomasses and coal- biomass blends: an experimental investigation., Fuel 81, 2002, pp. 1041- 1050. [ Links ]
Calvo, L.F.,; Otero, M., Jenkins, B.M., Moran, A., García, A. I., Heating process characteristics and Kinetics of rice straw in different atmospheres., Fuel Processing Technology, 85, 2004, pp. 279 291 [ Links ]
Defeche, J., Tratamiento De Los Residuos. Incineración y Valoración Energética de la Basura., Coloquio Sobre Residuos Sólidos, Sociedad Venezolana de Ciencias Naturales, 1980. [ Links ]
Di Blasi, C., Buonanno, F., Branca, C., Reactivities of some biomass chars in air. [ Links ]
Gayana, P., Adaneza, J., De Diego a, L. F., García, F., Cabanillas, A., Bahillo, A., HOAC, A., Veijonen, K., Circulating fluidised bed co-combustion of coal and biomass., Fuel 83, 2004, pp. 277286 [ Links ]
Folgueras, M. B., Díaz, R., Liberta, J., Prieto, I., Thermo-ravimetric analysis of coal and sewage sludge., Fuel 82, 2003, pp. 2051- 2055. [ Links ]
Fraga, G. L., Estudio Cinético, Dinanomecánico y termogravimétrico del Sistema Epoxídico BADGE (n= 0) /m´XDA, mediante las técnicas de análisis térmico: DSC, DMA y TGA., Construcción de un diagrama TTT, Tesis Doctoral, Universidad de Santiago de Compostela,. 2001. [ Links ]
Johannes, O. J., Kinetics Analysis using multivariate Non linear Regression., Thermal Anal, Cal. 60, 2000, pp. 641-658. [ Links ]
Kristiansen, A., Understanding coal gasification IEA., in IEA Coal Research, 1996, pp. 17-23. [ Links ]
Romero, S. A., Incineración de Residuos Sólidos Urbanos., Dpto. de Ingeniería Química. Facultad de CC. Químicas, Universidad Complutense de Madrid, 2002. URL: http://www.esi.us.es/AICIA/1997/inter.html. [ Links ]
Sima-Ella, E., Yuan, G., Mays, T., A simple kinetic analysis to determine the intrinsic reactivity of coal chars., Fuel, 84, 2005, pp. 1920-1925. [ Links ]
Vyazovkin, S., Wight Charles A. Model-free and model-fitting approaches to kinetic analysis of isothermal and no isothermal data Thermochimica Acta 340 341., 1999, pp. 53- 68. [ Links ]
Vuthaluru, H. B., Investigations into the pyrolytic behaviour of coal/biomass blends using thermogravimetric analysis., Bioresource Technology, 92, 2004, pp. 187 195. [ Links ]