Services on Demand
Journal
Article
Indicators
-
Cited by SciELO
-
Access statistics
Related links
-
Cited by Google
-
Similars in SciELO
-
Similars in Google
Share
Ingeniería e Investigación
Print version ISSN 0120-5609
Ing. Investig. vol.30 no.2 Bogotá May/Aug. 2010
Chemical composition and mass closure for airborne particulate matter in Bogotá
Freddy A. Vargas1 and Néstor Y. Rojas2
1 Chemical Engineering. M.Sc., Environmental Engineering, Universidad Nacional de Colombia. Bogotá, Colombia. Researcher, Grupo de Investigación en Calidad del Aire. favargasc@unal.edu.co 2 Chemical Engineering. Ph.D., Environmental Engineering. Associate Professor, Department of chemical and environmental engineering, Universidad Nacional de Colombia. Colombia. Director, Grupo de Investigación en Calidad del Aire. nyrojasr@unal.edu.co
Abstract
Particulate matter, measured as PM10, is the most concerning airborne pollutant in Bogotá. Determining its chemical composition is important for understanding its potential effects and to estimate various sources' contribution to such pollution. This paper gives the results of characterising the ionic species, carbonaceous material, metals and crustal elements present in airborne PM10 in Bogotá. An ion charge balance and mass reconstruction were done for determining consistency between chemical characterisation and gravimetric PM10. The composition was different in each area; however, the fractions contributing most to PM10 were crustal, 37% to 42% was related to fugitive and suspended dust, 12% to 11% was related to carbonaceus fractions, 43% to elemental carbon, 34% for organic matter and 5% to 8% for ionic fractions.
Keywords: particulate matter, PM10, mass closure, ionic balance, Bogotá, chemical composition.
Received: may 26th 2009
Accepted: jun 15th 2010
Introduction
A chemical plant includes independent operating units such as Urban air quality is a most concerning issue because of its relationship to human health and quality of life (Fenger 1999). The evidence has shown that an increase in air pollutant concentration has been associated with increased morbidity and mortality indicators (World Health Organisation 2006). According to the records concerning Bogotá's air quality monitoring network, as well as official and independent reports (SDA 2008; MMAVDT and IDEAM 2007; Gaitán and Behrentz 2009), the pollutant having the most concerning concentration levels is particulate matter having an aerodynamic diameter smaller than 10 micrometers, known as PM10.Previous studies have addressed the chemical characterisation of PM10 in Bogotá, focusing on heavy metals (Pachón and Sarmiento 2008; Roa 1999; Páez 1998). Pachón et al., (2008) determining that elemental and organic carbon accounted for between 15% and 60% of PM10 mass and ionic species (sulphates were a particularly important fraction). However, the small number of samples analysed might have been associated with a high degree of uncertainty. Rivera and Behrentz (2009) found that ion concentration was between 3.5% and 8% of PM10 mass; they analysed the concentration of metals but did not analyse carbonaceous fractions which are the most significant fraction of PM10 mass.
This work was aimed at running a thorough chemical characterisation of PM10 having a reduced degree of uncertainty. Chemical characterisation included metals, ionic species and carbonaceous fractions. PM10 massconcentration was gravimetrically determined and mass closure from chemical analysis was performed to check its consistency, following ionic balance analysis.
Methods
Sampling
Two sampling sites meeting the US EPA criteria for neighbourhood-scale air quality monitoring (US-EPA 2008; US-EPA 2006) were selected in the Carvajal (Kennedy) and Suba residential areas in south-eastern and northern Bogotá. Although Carvajal is a residential area it has been chosen by many small industries because of its easy access to avenues having truck traffic. Differences in chemical composition would thus be expected between Carvajal and Suba PM10 samples. Two sets of 55 integrated 24-h samples were taken simultaneously at each site for two months in 2007. Two Harvard impactors were used for collecting PM10 samples on 47-mm quartz and PTFE filters at Carvajal, whereas one Hi-Vol sampler was used for collecting PM10 on quartz filters and one Harvard impactor to collect PM10 on 47-mm PTFE filters in Suba.
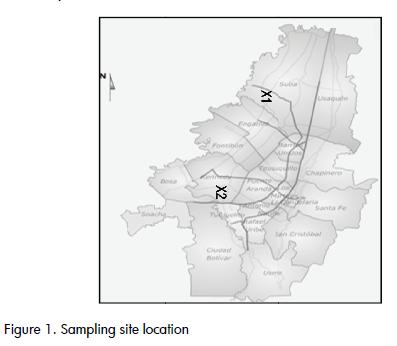
Filters were conditioned at 20±3°C and 45±5% relative humidity for 24-h and weighed on a Sartorius balance (10μg resolution for Hi-Vol filters) and a Sartorius microbalance (1µg resolution for 47-mm filters). Samples were then kept at -5°C to avoid volatilisation losses prior to chemical analysis.
Chemical analysis
- Energy-dispersive X-ray fluorescence spectrometry (EDXRF) was used for quantifying elements ranging from Na to U present in PM10 collected on PTFE filters, following US EPA Method IO 3.3 (EPA 1999) modified for using an Epsilon 5 spectrometer (PANalytical 2008).
- Thermal optical trasmittance (TOT) was used for determining the organic carbon (OC) and elemental carbon (EC) present in PM10 collected on quartz filters using SunLab equipment, following Georgia Institute of Technology's ASACA Lab procedures.
- Ion chromatography was used for quantifying cations (Na+, K+. NH4+ and Ca+2) and anions (Cl-, NO2-, NO3-, SO4=, oxalate=, formate- acetate-) present in PM10 collected on quartz filters, using Dionex300DX equipment, following the Georgia Institute of Technology's ASACA lab procedures.
- Mass closure The ion charge balance and the relationship between organic and elemental carbon were examined to check the chemical analysis' consistency. Mass closure was obtained following the model proposed by Lewis et al., (2003) and organic fraction contribution was determined following Turpin and Lim's recommendations (2001).
Results and discussion
Gravimetrically obtained particulate matter concentrations for the sampling period are shown in (Figure 2). The difference between the concentration using quartz and PTFE filters was significant for a few days (shown in the ovals in (Figure 2) due to unidentified operational errors.
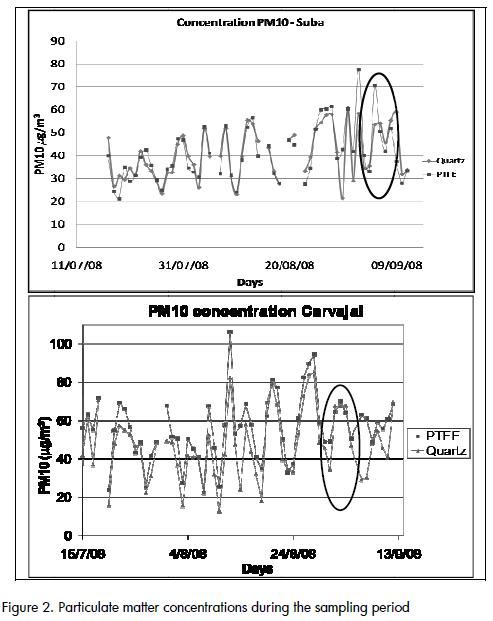
Concentrations obtained with PTFE filters tended to be higher than those obtained with quartz filters in Carvajal( Figure 3), which was probably due to the loss of fibres from the 47-mm quartz filters during filter handling. The difference occurred even when extreme values were removed from the data setsThis did not occur when handling Hi-Vol filters, which makes Suba concentrations more consistent when removing extreme values from the data sets. .
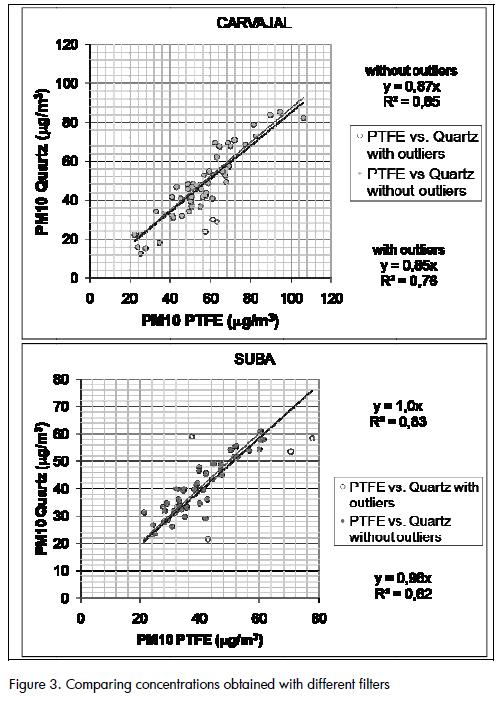
EDXRF results are shown in (Figure 4). grouped into high and low concentration levels. High relative concentrations of Al, Si, Ca and Fe suggest an important contribution by crystal material. Elements in the low relative concentration range (Zn, Cu, Pb) are normally associated with mobile and industrial sources. The presence of iodine (I) in Suba was unusual, since this element is not commonly reported as a component of PM10.
Zn and Pb concentration levels in Carvajal were in the same order of magnitude as those found in other Latin-American cities (Dawidowski 2007).
However, Pb concentration levels were two orders of magnitude lower than those found by Pachón and Sarmiento (2008) in Bogotá, at a Puente Aranda sampling site, this being the city's main industrial area, having a high density of metallurgy and metalwork facilities. Rivera and Behrentz (2009) also reported higher Zn and Pb levels at the Puente Aranda industrial site and lower levels in residential and commercial sites.
The OC/EC ratio was different at each sampling site in this study (Figure 5), which was attributable to different source contributions.
According to Seinfeld and Pandis (1998), OC/EC ratio values between 1 and 1.2 can be associated with combustion sources using low-sulphur diesel fuel. Zhang et al., (2009) have shown that the combustion of high-sulphur diesel produces a higher OC/EC ratio and higher carbonaceous fraction concentrations. In fact, OC/EC ratio and emission level change with driving patterns, engine load and mileage.
Figure 6 shows the mean ion composition of PM10. Ca+2 is the predominant cation, whereas SO4-2 and NO3- are the anions making the greatest contribution.
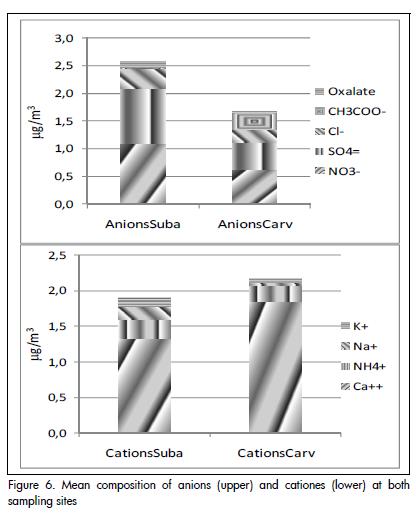
Ion contribution was between 5% and 10% of total PM10 mass at both sites, which agrees with Rivera and Behrentz's findings (2009).
No traps were used to avoid the entrainment of acid or alkaline gases (HNO3, H2SO4, NOX, SOX and NH4) in the filters, which could have resulted in overestimating ionic species. However, previous studies have shown that the concentration of such gaseous species is not high in Bogotá (Gaitán and Behrentz 2009). Although ammonium nitrate (NH4NO3) could have been lost from collected PM10, air temperature and humidity conditions in Bogotá during the sampling period (12.9°C, 83% relative humidity (MAVDT and IDEAM 2008a; MAVDT and IDEAM 2008b; MAVDT and IDEAM 2008c) would not have promoted such loss (Judith C Chow et al., 2005; Ashbaugh and Eldred 2004; Schaap et al., 2004).
An ionic charge balance was used to check the consistency of the ion analysis. It is reasonable to assume that particulate matter is neutral. Therefore, the sum of all cationic species measured as equivalent units should be equal to the sum of all anionic species (Maxwell-Meier et al., 2004) and a plot of cation equivalent units compared to anion equivalent units would show a 1:1 slope. Figure 7 however, shows that this condition was not satisfied when including the species analysed by ion chromatography.
An excess of cations at both sampling sites suggested that one or more anions were not quantified. The anionic deficit, calculated as being the difference between total cation equivalent units and total anion equivalent units, was found to have a strong association with Ca+2 (Figure 8). Anion deficit compared to calcium ion Such association suggested that the unquantified anion was CO3= and that CaCO3 there contributing to PM10 total mass.
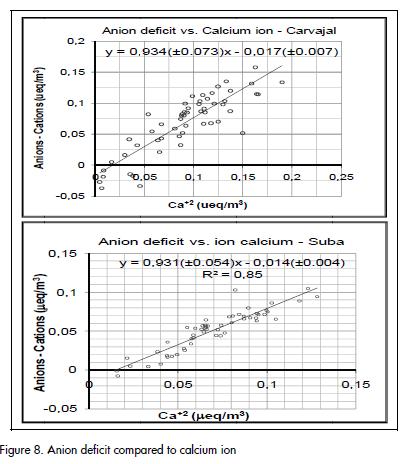
Mass closure
Mass closure calculations were made using the model suggested by Lewis et al., (2003), modified to take into account calcium carbonate from the ionic charge balance.
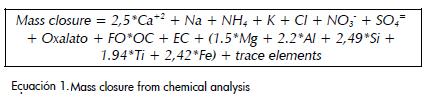
FO is the factor that quantifies the contribution of elements associated with organic carbon, including hydrogen and oxygen. This factor was assumed to be 1.6 for Carvajal and 1.8 for Suba, taking into account that both are within the range suggested for urban aerosols (1.6± 0.2) and that organic compounds would have a longer lifespan in Carvajal, which has an industrial influence, than in Suba, which has a rural influence (Turpin and Lim 2001).
A fairly good agreement was observed between gravimetric PM10 concentration and the result of mass closure from chemical analysis, as shown in Figure 9 and Figure 10. The correlation agreed with average results for PM10 studies (J C Chow et al., 2002; Andrews et al., 2000; Almeida et al., 2006; Vecchi et al., 2008). Outliers identified in Figure 10 corresponded to the same dates and the same group of data that showed differences between quartz and PTFE filters (Figure 2).
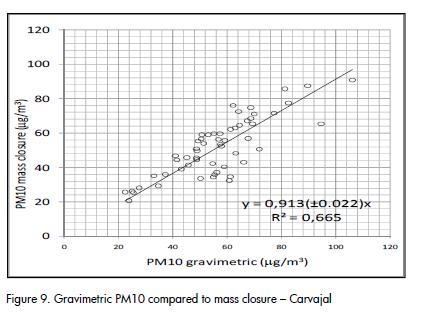
Based on mean gravimetrically-determined mass concentration on PTFE filters and mean chemical mass closure, mean PM10 composition was calculated at both sampling sites, as shown in Figure 11, where OM stands for organic material, calculated using the organic factor mentioned above.
The trace fraction in Figure 11 refers to metals and other low-concentration elements.
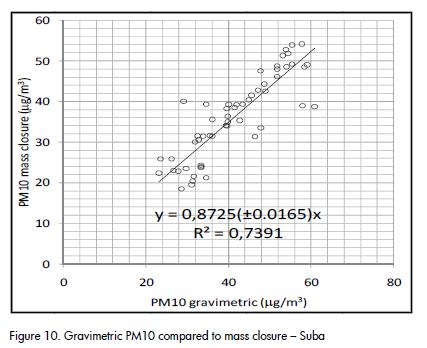
Crustal material, likely associated with suspended dust, makes a strong contribution to total PM10 mass. This kind of contribution is normally associated with coarse particles and would be significantly reduced if PM2.5 were analysed, as shown by Rivera and Behrentz (2009). Carbonaceous fractions made an even more significant contribution (50% to 65% of total PM10), agreeing with that reported by Pachón et al., (2008). Sources likely associated with these fractions are vehicles and industrial combustion sources such as boilers (Rivera and Behrentz 2009; Fandiño and Behrentz 2009; Rodríguez and Behrentz 2009). The ionic fraction was in the same range as that found in other studies conducted in Bogotá and was not as high as that found in American cities. The ionic fraction found by Pachón et al., (2008) was 9% of total mass at Puente Aranda, while Rivera & Behrentz (2009) reported 3.5% at Puente Aranda and 7% at a residential site. Unidentified material is commonly associated with moisture or a biased correction factor used for calculating organic material.
There were differences between Carvajal and Suba, first in PM10 concentration where Carvajal was higher than Suba; Suba showed lower levels of carbonaceous fractions and higher ionic content by composition. These differences were probably related to the kind of sources that affect each area in the city.
Conclusions
Carbonaceous material associated with industrial and mobile combustion sources accounted for the main contribution to PM10 at residential sites in Bogotá. Crustal material, associated with suspended dust was also significant.
The ionic charge balance results showed that carbonate ion should be analysed when characterising particulate matter to verify its neutrality. The ionic component of PM10 in Bogotá was lower than that found in American cities where thermal energy production increases the emission of ionic precursors into the atmosphere. The results of this study and its predecessors have shown that thorough chemical characterisation is useful for obtaining a better understanding of interactions between the chemical species that constitute particulate matter and the contribution of different sources. The use of tools such as the ionic charge balance and mass closure are key in checking internal consistency and validating chemical analysis results. Their use in further chemical characterisation studies is thus highly recommended, particularly if additional techniques (i.e. receptor models) are to be used for determining source contributions to particulate matter.
The resolution of EDXRF analysis for PM10 must be improved, either by adjusting instrument parameters or increasing the sampling time to collect higher particulate matter mass on each filter. Different sampling periods (i.e. day cf night) and their relationship to meteorological variables could help in understanding source contribution and the dynamics of particulate matter in Bogotá's atmosphere.
Acknowledgements
This work was supported by COLCIENCIAS and the Universidad Nacional de Colombia's National Research Office. The authors would like to express their sincere appreciation to Ted Russell and Jorge Pachón at the Georgia Institute of Technology for allowing us to use the TOT and IC instruments and contributing to our discussion on the results from this study.
Air Quality Guidelines: Global Update: Particulate Matter, Ozone, Nitrogen Dioxide, and Sulfur Dioxide. Copenhagen, Denmark: World Health Organization. 2005 [ Links ]
Almeida, S.M., C.A. Pio, M.C. Freitas, M.A. Reis, M.A. Trancoso., Approaching PM2.5 and PM2.5 - 10 source apportionment by mass balance analysis, principal component analysis and particle size distribution., Science of The Total Environment , 368, No. 2-3, 2006, pp. 663-674. [ Links ]
Andrews, E., P. Saxena, S. Musarra, L.M. Hildemann, P. Koutrakis, P.H. McMurry, I. Olmez, W.H. White., Concentration and Composition of Atmospheric Aerosols from the 1995 SEAVS Experiment and a Review of the Closure between Chemical and Gravimetric Measurements., Journal of the Air & Waste Management Association, 50, 2000, pp. 648-664. [ Links ]
Ashbaugh, Lowell L, Robert A. E., Loss of particle nitrate from teflon sampling filters: effects on measured gravimetric mass in California and in the IMPROVE network., Journal of the Air & Waste Management Association (1995), Vol. 54, No. 1, 2004, PP. 93-104. [ Links ]
Chow, J C., John G Watson, Eric S. E., E. Vega., Chemical composition of PM2.5 and PM10 in Mexico City during winter 1997., The Science of Total Environmental, 287, 2002, pp. 177-201. [ Links ]
Chow, J. C., John G. W., Douglas H. L., Karen L. M., Loss of PM2.5 nitrate from filter samples in central California., Journal of the Air & Waste Management Association, Vol. 55, No. 8, 1995, pp.1158-1168. [ Links ]
Dawidowski, L., Composición multielemental del material particulado, de los receptores a las fuentes., In I Congreso Colombiano de Calidad del aire, Manizales: Universidad Nacional de Colombia, 2007. [ Links ]
EPA., Determination of metals in ambient particulate matter using X-Ray Fluorescence (XRF) spectroscopy., EPA, 1999.. http://www.epa.gov/ttnamti1/files/ambient/inorganic/mthd-3-3.pdf. [ Links ]
Fandiño, M., Eduardo B., Actualización del inventario de emisiones atmosféricas provenientes de fuentes fijas en Bogotá a través de mediciones directas., M.Sc., thesis, Universidad de Los Andes, 2009. http://biblioteca.uniandes.edu.co/Tesis_12009_primer_semestre/525.pdf. [ Links ]
Fenger, J., Urban air quality., Atmospheric Environment 33, No. 29, 1999, pp. 4877-4900. doi:10.1016/S1352-2310(99)00290-3. [ Links ]
Gaitán, M., Eduardo B., Evaluación del estado de la calidad del aire en Bogotá., MSc thesis, Universidad de Los Andes, January. 2009. [ Links ]
Lewis, C., Gary N., Teri C., Ronald H., Source Apportionment of Phoenix PM2.5 Aerosol with the Unmix Receptor Model., Journal of the Air & Waste Management Association, 53, 2003, pp. 325-338. [ Links ]
MAVDT, IDEAM., Informe 163, Condiciones meteorológicas en Colombia durante junio de 2008 y proyecciones en el corto y largo plazo. IDEAM., August, 2008a. [ Links ]
MAVDT, IDEAM., Informe 162, Condiciones meteorológicas en Colombia durante junio de 2008 y proyecciones en el corto y largo plazo., IDEAM, July, 2008b. [ Links ]
MAVDT, IDEAM., Informe 164, Condiciones meteorológicas en Colombia durante junio de 2008 y proyecciones en el corto y largo plazo., IDEAM, September, 2008c. [ Links ]
Maxwell-Meier, K., R. Weber, C. Song, D. Orsini, Y. Ma, G. R. Carmichael, D. G. Streets., Inorganic composition of fine particles in mixed mineral dustpollution plumes observed from airborne measurements during., ACE-Asia, 2004. http://www.agu.org/pubs/crossref/2004/2003JD004464.shtml. [ Links ]
MMAVDT, IDEAM. Informe anual sobre el Estado del Medio Ambiente y los Recursos Naturales Renovables en Colombia: Calidad del Aire., IDEAM, 2007. http://www.ideam.gov.co/infoanual/PDFSeccionados/CalidadAirePreliminares.pdf. [ Links ]
Pachon, J., A. Russell, H. Sarmiento, B. Galvis., Identification of secondary aerosol formation in Bogota: a preliminary study., In. Portland: A&WMA, June, 2008. [ Links ]
Pachón, J., Sarmiento, H., Análisis espacio-temporal de la concentración de metales pesados en la localidad de Puente Aranda de Bogotá-Colombia., Revista de Ingeniería Universidad de Antioquia, No. 47, 2008, pp. 120-133. [ Links ]
Páez, C., Determinación de metales en las partículas del aire., M.Sc. thesis, Universidad de Los Andes, 1998. [ Links ]
PANalytical. Analysis of air filters according to EPA method I.O 3.3. PANalytical B.V., 2008. [ Links ]
Rivera, J., Eduardo B., Identificación de fuentes de contaminación por material partuclado en Bogotá., MSc thesis, Universidad de Los Andes, 2009. [ Links ]
Roa, M., Medición de metales en las partículas del aire., MSc thesis, Universidad de Los Andes, 1999. [ Links ]
Rodríguez, P., Eduardo B., Actualización del inventario de emisiones de fuentes móviles para la ciudad de Bogotá a través de mediciones directas., M.Sc. thesis, Universidad de Los Andes, 2009. [ Links ]
Schaap, M., G. Spindler, M. Schulz, K. Acker, W. Maenhaut, A. Berner, W. Wieprecht, et al., Artefacts in the sampling of nitrate studied in the "INTERCOMP" campaigns of EUROTRAC AEROSOL.. Atmospheric Environment, 38, No. 38, 2004, pp. 6487-6496. [ Links ]
SDA, Secretaría Distrital de Ambiente., Informe Anual de Calidad del aire de Bogotá - 2007., Informe. Bogotá D.C.: Secretaría Distrital de Ambiente, June, 2008. [ Links ]
Seinfeld, John H., S. N. Pandis., Atmospheric Chemistry and Physics: from Air Pollution to Climate Change.,1st ed., New York: John Wiley, 1998. [ Links ]
Turpin, Barbara, H. J. Lim., Species Contributions to PM2.5 Mass Concentrations: Revisiting Common Assumptions for Estimating Organic Mass., Aerosol Science and Technology 35, 2001, pp. 602-610. [ Links ]
US-EPA. Guidance on the Use of Models and Other Analyses for Demonstrating Attainment of Air Quality Goals for Ozone, PM2.5, and Regional Haze Draft 3.2. U.S. EPA; Research Triangle Park, 2006. [ Links ]
US-EPA., Probe and Monitoring Path Siting Criteria for Ambient Air Quality Monitoring - Appendix E to Part 58., Código de Reserva Federal, 2008. [ Links ]
Vecchi, R., M. Chiari, A. D'Alessandro, P. Fermo, F. Lucarelli, F. Mazzei, S. Nava, et al., A mass closure and PMF source apportionment study on the submicron sized aerosol fraction at urban sites in Italy., Atmospheric Environment 42, No. 9, 2008, pp. 2240-2253. World Health Organization. 2006. [ Links ]
Zhang, Jie, Kevin He, Yunshan Ge, and Xiaoyan Shi., Influence of fuel sulfur on the characterization of PM10 from a diesel engine., Fuel 88, 2009, pp. 504-510. [ Links ]