Introduction and Background
As traffic loads continue to increase, researchers must constantly evaluate the structural behavior of pavements. Over the last decade, nanotechnology has evolved into a promising source of materials to help meet this problem. Within the field of nanotechnology applied to pavements, carbon nanotubes and carbon nanofibers (CNTF) stand out. Given the outstanding properties (as notably strength) exhibited by CNTF, this technology contributes to improved mechanical response in pavements.
A number of methods may be used to assess the dynamic properties of an asphalt mixture. Often, these methods study permanent strain accumulations, cyclic load response and resilient modulus (Mr). Tian et al. (1998) and Santagata et al. (2012) have carried out research on carbon nanotubes as an asphalt modifier; they observed changes in the asphalt's elastic and viscous moduli. These changes brought about by CNTF modification represent an economically feasible solution for pavement durability (Bai et al., 2003; Bergman et al., 2011; Hassani et al., 2005; Xiao et al., 2011).
Research has shown that modifying asphalts with CNTF structurally reduces asphalt layer thickness and, in turn, avoids higher quantities of aggregates. Naturally, CNTF modification can be said to result in positive environmental effects. Furthermore, the literature reports that carbon nanotubes boost mixture strength under permanent strains and enhance resistance to thermal cracking by virtue of improved bitumen elastic response (Mathews et al., 2007; Tarefder et al., 2005; Tian et al., 1998). Lucente-Schultz et al. (2009) discovered anti-oxidative properties in asphalts modified with CNTFs, and undoubtedly, diminished oxidation would result in better long-term pavement performance and translate into less frequent layer maintenance (Li et al., 2004; Park et al., 2010; Santagata, 2012).
With regard to the CNTF used herein, Nickel, Copper and Aluminum (NiCuAl) catalyst particles were used. The current article compares unpurified CNTF and purified CNTF (purified via HNO3 filtering) to determine which modification is most effective for improving HMA-2 (Type 2 refers to 1/2" maximum size of course aggregate mix) mechanical performance. The nanomaterial used for modification combines carbon nanotubes and carbon nanofibers. A conventional HMA-2 was employed as a control with which to compare the CNTF modified HMA-2s. For this investigation, analysis consisted of studying the material's physical and thermic properties with a Scanning Electron Microscope (SEM) and a thermogravimetric analysis (TGA).
As for the hot-mix asphalt studied, the HMA-2 is composed of coarse and fine aggregates, filler and a bitumen binder. Several design methods explain how to create an HMA-2, and the most common methods are as follows: Hubbard, Hveem, Asphalt Institute, SUPERPAVE and Marshall. For the present research, the Marshall method was employed to arrive at the optimum percentage of bitumen in the mixture as well as to manufacture the HMA-2 samples.
Looking at the HMA-2, the asphalt concrete (AC) pavement layer, which represents the top of the material, is directly exposed to traffic loads; vehicles exert high pressure and high tension stress on this layer. Therefore, the AC layer must have the highest elastic modulus values found in a pavement structure (Asi, 2005). AC can be divided into three macro-colloidal phase systems: solids (aggregates and filler), liquid (asphalt) and gas (air voids). While the solid phase provides the system's elasticity and resistance to shear stresses, the liquid phase grants viscoelastic properties and cohesion. For its part, the gaseous phase indirectly affects the system by changing its physical and mechanical properties (Bernier et al., 2012; Brown, 1995; Ahmadinia et al., 2011; Radziszewski, 2007).
Insofar as the testing performed here is concerned, rutting, Mr and fatigue tests form the basis of the present analysis. Accumulative permanent strain susceptibility in HMA-2 depends on mixture gradation, aggregate type, air voids, bitumen type and amount, ambient temperature and traffic load type and amount (Balghunaim et al., 2011; Mathews et al., 1992; Radziszewski, 2007; Tian et al., 1998; Weng et al., 2000). Because of the myriad factors affecting mixture strain susceptibility, it is difficult to predict rutting response by merely adjusting the bitumen's properties. Therefore, isolating bitumen influence on rutting implies rigorous control during mixture manufacture. If rigorous control is achieved, mixture design is considered constant and bitumen-modifier effects are identifiable (Alata et al., 2011; Anderson, 1996; Roberts et al., 1991). Research suggests improvements in rutting pathologies are related to increased coarse aggregate strength and angularity or changes in bitumen stiffness (Abdullah et al., 1998; Abdulshafi et al., 1988; Al-Hadidy et al., 2009; Wong et al., 2004).
In light of the fact that fatigue is mainly a function of bitumen properties and mixture air void content, background information becomes crucial for predicting HMA-2 fatigue behavior (Abojaradeh, 2003). Cracking pathology, generally associated with cyclical traffic loads, can also be understood in terms of temperature: low temperatures contribute to fatigue, and they are recognized as one of the principal failure mechanisms in flexible pavements. Physically, cracking is defined as a series of longitudinal or interconnected fissures (cracks) caused by vehicular repetitive loads over an AC surface layer. Fissures usually develop in areas affected by traffic loads that result in higher stresses or strains (Al Qadi et al., 2008; Hassani et al., 2005; Huang et al., 2005). For the most part, fatigue-induced cracking begins as small longitudinal fissures and then demonstrates an "alligator cracking" pathology (i.e. cracking that resembles alligator skin) (Alata et al, 2011; NCHRP, 2004; Petersen et al., 1994). Approaches to defining fatigue failure include trapezoidal samples to establish the role played by set displacement, in addition to external temperatures applied to an initial sample length with a determined frequency. Even though set displacement is sinusoidal, the resultant strain it occasions is pseudo-sinusoidal. The following equation, Equation 1 - Fatigue resultant strain (Tarefder et al., 2003) expresses this process:
Where εm (t) and εa (t) are the strain's average and amplitude over a period of time; both variables evolve more slowly than load pulse, ω. When the stress applied to maintain a constant strain is reduced by 50 %, the sample is considered to have failed (in X number of cycles). On a Log-Log graph, fatigue laws are determined by comparing constant displacement (load) and number of cycles to failure. The value at 1x106 cycles is defined as the material's fatigue strength (Bahia et al., 2001; Khiavi et al., 2013; Williams et al., 1955).
The elastic theory states that if the applied load is small (relative to material strength) and repeated numerous times, strain under each load repetition should exhibit low or zero deformation or strain and the material is theoretically regarded as elastic. As pertains to HMA-2 modified performance, elasticity is crucial insofar as the initial phase of load application entails a significant portion of "elastic" permanent and plastic strains for each load repetition. It is worth noting that plastic strains tend to decrease as load repetitions increase: between 100 and 200 repetitions, plastic strain is nearly recoverable. An elastic modulus based on a recoverable strain (εr) under repetitive loads represents the Mr; it is mathematically expressed as follows, Equation 2 - Elastic Modulus (Tian et al. , 1998):
Where σd is the axial stress of an unconfined compression test or the axial stress of a confined pressure in a tri-axial compression test (deviator stress). Generally speaking, tests for Mr are measured applying a Haversine wave form for load application (Balghunaim et al., 2005; Huang et al., 2005; Mathews et al., 2007; Tarefder et al., 2005; Van Dijk et al., 1977). Here, Nottingham Model equations can be used for frequency-temperature superposition (TTS) in Mr master curves, see Equation 3 - Nottingham Model Equations (Al-Qadi et al., 2008) and Equation 4 - Nottingham Model Equations (Al-Qadi et al., 2008):
Where X represents TTS, αT represents the modification factor as a function of temperature, f the frequency in Hertz, T the absolute temperature in Kelvin during testing and Ts the reference absolute temperature in Kelvin. The authors of this study used a Ts of 15 °C on account of the fact that it is the most common Mr design temperature for HMA-2 in Bogota, Colombia per the Rational Pavement Design Method (Fakhri et al., 2002; Ghanizadeh et al., 2013; Khiavi et al. 2013;.
Materials and Methods
The HMA-2 used here was made in accordance with the standards laid out in ASTM D6307 and INVIAS 450. Crushed aggregates come from the region of Coello, Colombia. Bitumen (or Asphalt Cement AC 60-70) 60/70 (as determined by the ASTM D 1586 penetration test) was extracted from vacuum bottoms in Barrancabermeja, Colombia. The CNTF modifiers were obtained by means of the Catalytic Vapor Deposition (CVD) technique at 700 °C using a NiCuAl catalyst with a Cu/Ni molar relation of 0,33. These modifiers were used in unpurified and purified states, with purification achieved via HNO3 filtering. CNTF were directly added to the bitumen at a rate of 2000 revolutions per minute and at a proportion of 2 % weight (relative to bitumen weight). These conditions were chosen based on the research conducted by Jimenez (2012) as part of a Colombian research group's (CECATA) an attempt to determine the optimum temperature, CNTF amount and frequency to modify bitumen/AC. In total, three mixture types were studied: conventional HMA-2, HMA-2 modified with unpurified CNTF and HMA-2 modified with purified CNTF.
HMA-2 dynamic characterization included rutting tests carried out with a wheel-tracking machine at a temperature of 60 °C, a pressure of 0,6 MPa and a frequency of 1 Hz (according to the standard NF P 98-253-1). Bitumen aging tests-RTFO and PAV-were performed with a dynamic shear rheometer (DSR); however, due to limitations imposed by the laboratory's capabilities, aging tests were not done on the HMA-2. Mr testing was done with the Nottingham Asphalt Tester (NAT) at 10, 20 and 30 °C, with frequencies of 2,5, 5 and 10 Hz and applying a Haversine waveform for load application. Concerning fatigue in four configured trapezoidal samples. Fatigue benchmarks were analyzed using a frequency of 10 Hz at room temperature (20 °C) and three displacement amplitudes (220 x 10 - 6 m, 150 x 10 - 6 m and 90 x 10 - 6 m), as outlined by the standard NF P 98-261-1 (Asphalt Institute, 1997; Johnson et al., 1998; Kim et al., 1992).
To conduct testing, three 30 x 30 x 5 cm samples were made for each HMA-2 to study rutting. Additionally, three Marshall briquettes were made for each HMA-2 to calculate Mr. Lastly, 12 trapezoidal samples were made for each HMA-2 to obtain fatigue laws.
Accurate aggregate characterization and gradation were of critical importance to ensure mixture quality (see Tables 1 and 2).
Table 1 HMA Aggregate Characterization
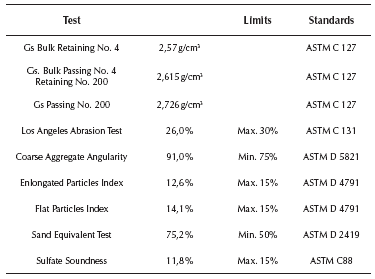
Source: Data obtained in the civil engineering materials laboratory of Pontificia Universidad Javeriana.
Results and Discussion
Readers should note that percentages discussed in this paper refer to the comparison of the two modified HMA-2s and the conventional HMA-2 (without CNTF modification). Figures 1, 2 and 3 show dynamic characterization results for the three HMA-2 types studied.
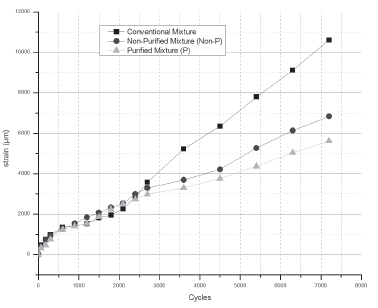
Source: Data obtained in the civil engineering materials laboratory of Pontificia Universidad Javeriana.
Figure 1 Critical Strain Cycles (Modified and Conventional HMA-2).
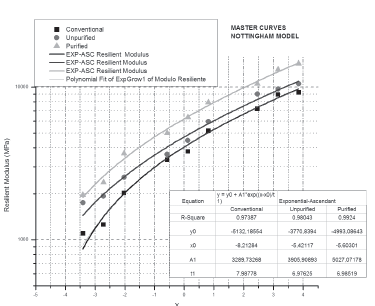
Source: Data obtained in the civil engineering materials laboratory of Pontificia Universidad Javeriana.
Figure 2 Nottingham Model Master Curves for Resilient Modulus (Modified and Conventional HMA-2) X = Frequency-Temperature Superposition.
Figure 1 displays critical rutting load cycles. Between 18002700 load cycles, rutting increased 31,0 % for HMA-2 modified with unpurified CNTF, 28,1 % for purified CNTF and 33,7 % for conventional mixture, these percentages are calculated with respect to the maximum strain registered. Between 4500-5400 load cycles, unpurified CNTF rutting increased to 49,6 %, purified CNTF increased to 41,15 % and conventional mixture increased to 73,5 %. Between 6300-7200 load cycles HMA-2 modified with unpurified CNTF increased to 64,4 %, HMA-2 modified with purified CNTF increased to 53,0 % and conventional mixture to 100% with respect to the maximum strain registered. To understand these rutting improvements in both modified mixtures, it is important to consider CNTF's capacity for increasing both the stiffness and elastic modulus of the bitumen. Bitumen properties were controlled through the rutting parameter |G*|/sin(δ) or the Multi-Stress Creep Recovery Test (MSCR) conducted with a DSR (Balghunaim et al., 1988; Brown, 1995; D' Angelo et al., 2007; Ozen et al., 2008; Radziszewski, 2007).
As previously mentioned, coarse aggregate properties (e.g. gradation) affect HMA-2 rutting (Roberts et al, 1996). For this reason, the authors of this study reiterate the need to maintain strict control of the HMA-2 manufacturing process and stress that similar samples with the same optimum percentage of bitumen must be created. In so doing, purified or unpurified CNTF become the only variables to account for when testing. Given that coarse aggregate properties and gradation, in addition to bitumen percentage, were constant, the results clearly indicate rutting changed as a factor of CNTF modification (Alvarez et al., 2006; Balghunaim et al., 2002; FHWA, 1995; Mathews et al., 1992; Moghaddam et al., 2014; Radziszewski, 2007; Tayfur et al., 2005).
Figure 2 presents Mr Master Curves as determined using Nottingham Model equations. Unpurified CNTF samples showed 11 % increases in Mr values, and purified CNTF samples showed 34 % increases. These results are consistent with observed rutting response, for purified CNTF tended to increase bitumen stiffness and elastic response. As a result, mixture recovery improved after a charge-discharge load application. To this end, researchers have studied the effect of purified CNTF on bitumen properties, finding an increase in elastic and viscous moduli (Abojaradeh, 2003; Chen et al., 2002; Fakhri et al., 2002; Johnson et al., 1998; Khiavi et al., 2013; Xiao et al., 2011). Yet, on the whole, research does not show unpurified CNTFs to significantly increase stiffness or viscous modulus, though such modification improves the elastic modulus (Al Qadi, et al., 2008; Roberts et al., 1991). Such an increase in elastic modulus is ideal for managing fatigue failure mechanisms: according to the elastic principle, high frequency load application generates improved elastic modulus values due to the fact that the plastic strain for each load repetition is inversely proportional to the increase in the number of cycles (Daniel et al., 2002).
As far as the Mr is concerned, the scientific literature has reached a consensus: Mr should be used to represent HMA-2 stress/strain relations given the type of load application that follows the Haversine principle (that is, closer to observed conditions) [confuse] (Abojaradeh, 2003; Alata et al., 2011; Caro et al., 2011; Tarefder et al., 2003).
Figure 3 depicts HMA-2 fatigue laws. Fatigue laws allow researchers to evaluate mixture fatigue behavior. These laws analyze the momentum strain that a trapezoidal sample can resist after 1 x 106 load cycles. Strain is represented by three displacements, 90 x 10-6, 150 x 10-6 and 220 x 10-6 m, and controlled using stress data given by sensors. With regard to fatigue results, HMA-2 modified with unpurified CNTF required a displacement of 210 x 106m to reach failure point, whereas HMA-2 modified with purified CNTF required a 154 x 10-6 m displacement. For comparison, conventional HMA-2 required 145 x 10-6 m. In other words, using HMA-2 modified with unpurified CNTF would result in considerable increases in pavement service life.
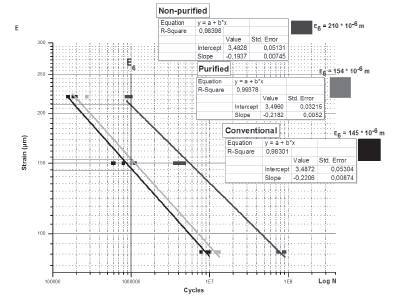
Source: Data obtained in the civil engineering materials laboratory of Pontificia Universidad Javeriana.
Figure 3 Fatigue Laws (Modified and Conventional HMA-2).
Slopes representing fatigue parameter p (a regression slope) were as follows: β = -0,19 for unpurified CNTF; β = -0,21 for purified CNTF; and, β = -0,22 for conventional. Unpurified CNTF fatigue was 44 %% better than that that of the conventional HMA-2, and fatigue for the purified CNTF produced results quite similar to those of the conventional HMA-2-a relative improvement of only 6% (Tarefder et al., 2001; Tian et al., 1993). Increases in fatigue for unpurified CNTF can be attributed to the effect of increased bitumen elastic modulus occurring without concomitant increases in the material's viscous modulus or stiffness. Catalyst impurities cannot be disregarded here, for they may prevent the bitumen from attaining a higher viscous modulus even though the catalyst results in a higher elastic modulus (Asi, 2002).
A physical event may also be partly responsible for this behavior: the unpurified CNTF's damping effect on cyclic load application allows the mixture to display a better elastic response (Al-Khateeb et al., 2004; Casey et al., 2008; Huang et al., 2005; Rogue et al., 1999; Santagata et al., 2012).
The literature associates improved fatigue bitumen parameter |G*|x sin(δ) with improved elastic modulus at a constant viscous modulus for low or room temperatures (Bernier et al., 2012; Brown, 1995). Since fatigue mostly stems from bitumen properties and optimum bitumen percentage, the prediction of a mixture's fatigue response ought to be done with a bitumen frequency sweep or a fatigue law determined by DSR testing (Balghunaim et al., 2001; Kim et al., 1992; Richards, 1959; Sousa et al., 1991).
Conclusions and Recommendations
Compared to conventional HMA-2, HMA-2 modified with CNTF offers improved durability and rutting resistance. When specifically comparing HMA-2 modified with purified CNTF and that modified with unpurified CNTF, the former is observed to have higher Mr values and offer more resistance to rutting. Improvements with regard to these two aspects of asphalt reduce asphalt layer thickness and decrease permanent strain accumulation for loads at higher temperatures (a short-term parameter that helps prevent rutting pathologies). However, HMA-2 modified with unpurified CNTF demonstrate better fatigue response, an integral variable in terms of long-term durability because it relates to thermal or load cracking at low or intermediate temperatures. In light of these observations, unpurified CNTF might prove a more suitable modifier if researchers aim to increase bitumen elastic modulus without significantly altering the viscous modulus.
Moreover, unpurified CNTF provides economic savings: it is cheaper than purified CNTF and its use avoids the time-consuming HNO3 purification process, which depends on specialized equipment and produces pollutants.
To conclude, the authors of the present study make three recommendations: i) further research into HMA-2 fatigue response at low temperatures using liquid nitrogen; ii) evaluation of HMA mechanical properties by way of carbon nanofibers and nanotubes as separate modifiers; and, iii) determination of the financial impact of different design methods and recovery programs on asphalt production and research.