Introduction
The study and use of natural coagulants/flocculants in water purification have had a growing interest due to their advantages over chemical coagulants/flocculants, such as low toxicity, biodegradability, low cost, abundant sources of production and mainly elimination of the toxic risk to the human being (Peruco, Lenz, Fiori and Bergamasco, 2013; Saritha, Srinivas, and Vuppala, 2017).
The conventional use of inorganic coagulants such as aluminum sulfate and ferric chloride, generate problems with their cost, undesirable large volumes of non-biodegradable sludge and effects on human body. For ferric salts, careful control in dosification is necessary because excessive residual iron could produce colored water (Choy, Prasad, Wu, Raghunandan, and Ramanan, 2014). For aluminum, the toxic risk is related to the presumed increase in the intake at 4 mg Al/day from aluminum sulfate (Suay and Ballester, 2002; World Health Organization, 2011). It also affects the liver, heart, brain, etc. and is related to Alzheimer's degeneration (Hayder and Rahim, 2015). In addition, the use of aluminum sulphate requires a good pH regulation, otherwise, clarification process is poor and solubilized aluminum can cause alterations in the effluent quality, due to the re-stabilization of charged particles in water (Cogollo, 2011).
The total or partial substitution of the traditionally used coagulant, aluminum sulfate, with natural coagulants aims mainly to eliminate the toxic risks due to its biodegradation. But it also seeks to solve the problem of final disposal of the residual sludge and its incidence on plant communities, since these sludges have a poor dehydration capacity (90% water) and 40 to 50% unreacted aluminum (Peruco et al., 2013; Antov, Saban, Prodanovic, Kukic, Vasic, Dordevic, and Milosevic, 2018).
The coagulation and flocculation processes aim to remove particles and organic matter dissolved in natural water, in order to improve aesthetics and health aspects of water. The main mechanism of coagulation/flocculation of polymers is the sweeping action due to their high molecular weight and high number of segments in their chains (Mihelcic and Zimmerman, 2012; Silva, 2015).
Several polymers extracted from natural sources have been used as coagulants in natural water, such as Nirmali seed (80 % turbidity removal), Cassia angustifolia seed, mesquite bean, Cactus latifaria, chestnut and acorn. All of them have reached high turbidity removal from 70% to 93%. In contrast, Cactus opuntia spp. has reached only 50 % (Farhaoui and Derraz, 2016; Vijayaraghavan, Sivakumar and Kumar, 2011). Other studies assayed Carica papaya seeds (90%), Citrus sinensis peel (85%), Feronia limonia seeds (77%) and Tamarindus indica seeds (80 %), as natural coagulants with relevant values of turbidity removal (Choy et al., 2014).
The most studied natural coagulant is Moringa Oleífera and presents a high removal efficiency for natural waters of high turbidity and even for waters of low turbidity (< 5 Nephelometric Turbidity Unit, NTU) with 6,5 abs/m color, 765 Colony forming unit (CFU)/100 mL and 605 CFU/100 mL E. coli, with a reduction of 41,4, 76,8 and 81,8%, respectively, for a dose of 500 mg/L (Pritchard, Craven, Mkandawire, Edmondson and O'Neill, 2010). It must also be considered that the use of 5 % Moringa oleifera seeds does not affect measurements of hydrogen potential (pH), conductivity, alkalinity, cation and anion concentrations, but concentration of organic matter in the treated water can increase considerably, acting as precursor of trihalomethanes during desinfection with chlorine (Ndabigengesere and Narasiah, 1998). On the one hand, increasing the organic matter, nitrate and phosphate contents limits shelf life of coagulants and compromises its feasibility(Megersa, Beyene, Ambelu, and Triest, 2018). On the other hand, with the use of exudates gums from Samanea saman, a significant removal of fecal coliforms (99,7%) and total coliforms (99,8%) was achieved (González, Chávez, Mejías, Mas y Rubí, Fernández, and León de Pinto, 2006). This occurs because natural coagulants are not only water clarifying agents, but they also have antimicrobial properties in some cases (Saranya, Ramesh and Gandhimathi, 2014; Antov et al., 2018; Megersa et al., 2018).
For all the above reasons, the use of natural coagulants/ flocculants for purification has been widely studied in countries such as Spain, Serbia, Brazil, Iran, among others. However, in Ecuador there are few works carried out in this line of research, especially considering the reuse of organic food waste as a source of natural coagulants/flocculants (Bongiovani, Konradt-Moraes, Bergamasco, Lourenco, and Tavares, 2010).
In Ecuador, the generation of solid waste was 4,06 million metric tons in 2016, with a per capita generation of 0,74 kg/inhabitant/day and it was mainly constituted by organic solid waste (61 %) (Instituto Nacional de Estadísticas y Censos, INEC, 2016). The final disposal of solid waste is mainly in landfills (39 %), where there are several compounds of interest that can be extracted (INEC, 2016). With the use of biowaste, the useful life of sanitary landfills and the maintenance costs involved could be enhanced. Choy, Prasad, Wu, Raghunandan, and Ramanan (2015) identified at least 14 natural coagulants extracted from vegetables and legumes considered common. Likewise, It has been demonstrated that underutilized or untreated biomass (carboxycellulose nanofibers) can be used as an effective Cd (II), Pb (II) and other heavy metal ions removers for water purification (Sharma, Joshi, Sharma, and Hsiao, 2017; Sharma, Chattopadhyay, Sharma, Geng, Amiralian, Martin, and Hsiao, 2018a and Sharma, Chattopadhyay, Zhan, Sharma, Geng, and Hsiao, 2018b).
Consequently, it is proposed to conduct a study on the efficiency of the coagulant/flocculant activity of three natural polymers derived from organic solid food waste (plantain peel starch, orange peel pectin and tamarind seed extract) for the purification of water.
Materials and Methodology
The study was carried out in three experimental stages: 1) extraction and characterization of natural polymers, 2) evaluation of the coagulant and flocculant activity and 3) characterization of the residual sludges and their toxicological effect.
Extraction and characterization of natural polymers
Starch was extracted from the residues of plantain peel (Musa paradisiaca L). These residues also contained a small amount of unused plantain and rachis. The procedure was based on the methodology proposed by Hernández-Medina, Torruco-Uco, Chel-Guerrero and Betancur-Ancona (2008). Peels were selected, washed and milled in a Ramon cutter employing a ratio peel: 1 500 ppm sodium bisulfite solution (from Loba Chemie I.) of 1 : 0,5 (w/v) and pectinase at 0,2 mL/kg of peel. Then, the paste obtained was mixed in a Cripto Peerless EC-30 mixer with a ratio peel paste: 0,3 % citric acid solution of 1 : 0,5 (w/v). This last step was repeated three times. Then, the blend was allowed to stand for 12 hours and the supernatant was eliminated. The sedimented material was filtered through the No. 200 sieve using water (three washes) to eliminate fibers. The fraction that passed the sieve was decanted for 12 hours, and the lower white layer was separated and dried in a Blue M OV-500C-2 stove at 50 0C for 24 hours.
Pectin was extracted exclusively from albedo of orange peel (Citrus sinensis) based on the methodology proposed by Devia (2003). The following modifications were applied: acid hydrolysis at pH 1 with H2SO4 MERCK, filtration with cloth to remove insoluble solids, precipitation with ethanol 96 %, drying on a BLUE M OV-500C-2 stove at 35 °C for 12 hours, grinding in a Thomas 4275-Z10 mill and sieving through the No. 18 sieve.
Tamarind seed (Tamarindus indica L) extract was obtained from residues of wet pulping process, which also contained pulp and fiber residues; based on the methodologies proposed by García (2007) and Hernández, Mendoza, Salamanca, Fuentes, and Caldera (2013) with some modifications. Seeds were hydrated for 5 days to remove the skin; then, the almonds obtained were dryed in a Memmert UN55plus convection oven at 25-35 °C for 2 days. Dry almonds were grinded in a Thomas 4275-Z10 mill to obtain a fine white powder, which was put through the No. 18 sieve. Afterward, oil was extracted using a ratio powder: ethanol 96 % of 5 : 95 for 30 minutes. The solids were settled, separated and dried in the Memmert UN55 plus convection oven at 35 °C for 18 hours. Solutions of 5 %, 1 % and 0,1% (w/v) of defatted almond powder in 3 different solvents: distilled water, 0,5 M sodium chloride (from J. T. Baker) and 10 mM of ammonium acetate (from Loba Chemie) were prepared, heated to 65 °C and the supernatants were separated (tamarind seed extracts).
The extracted natural polymers were characterized by moisture, ethereal extract, protein, ash, crude fiber and total carbohydrates assays according to the Association of Official Agricultural Chemists (AOAC) methods: AOAC 925.10, AOAC 920.85, AOAC 2001.11, AOAC 923.03, International Cereal Chemists (ICC) #13 and Food and Agriculture Organization (FAO), respectively (Association of Official Analytical Chemists and Horwitz, 2000).
Particle size and zeta potential were determined in a particle size analyzer Brookhaven model 90 Plus in 1%w/w aqueous solutions of each polymer.
IR spectra were obtained in a transmission cell of Perkin Elmer Model Spectrum One Fourier Transform Infrared Spectrometer. Pellets of dried samples were prepared employing potassium bromide FTIR grade (from Sigma-Aldrich). The spectrum of pectin and starch were compared with the spectrum of commercial food grade citric pectin and potato starch (from Loba Chemie).
Scanning electron microscopy (SEM) images were obtained by coating the dried samples with a thin layer of gold using a sputter coating apparatus Spi Module at 4 mBar for 10 s. The coated samples were examined in a Tescan Model Vega LMU scanning electron microscope operating at 15 kV or 20 kV in the secondary electron imaging mode.
Evaluation of the coagulant and flocculant activity
Natural water sampling: Raw water was obtained from a water purification plant. Sampling point was located before addition of chemical coagulant and aluminum sulfate. After undergoing a primary treatment of decantation, parameters measured in situ were temperature, pH and conductivity with a pH/Cond 340i WTW equipment.
Physicochemical and microbiological characterization of natural and clarified water: Raw and clarified water were characterized in their physico-chemical and microbiological properties by standardized assays of turbidity, color, solids (totals, settleable, suspended and dissolved), pH, alkalinity, Chemical Oxygen Demand (COD), Biochemical Oxygen Demand (BOD5), hardness, aluminum and coliforms (APHA, 2005).
Coagulant and flocculant activity assays: Five chemical substances were used: starch, pectin, tamarind seed extract, aluminum sulfate (8,32%Al2O3) and polyacrylamide (PAM). PAM was used as a reference for commercial polymer only in flocculant activity.
Primary coagulant activity assay: it was carried out with an adaptation of the methodologies used by García (2007) and Ghebremichael, Gunaratna, Henriksson, Brumer, and Dalhammar (2005), with the purpose of using a sample volume of 10 mL. It consisted in preparing synthetic turbid water with kaolin adjusted to 10 and 280 NTU (average value and highest value were found in the water purification plant). Then, 10 mL of turbid water were placed in a test tube, adding several doses from 50 to 200 μL of coagulant substances and distilled water (for the control). The sample was homogenized and initial absorbance was measured in a Hach DR 2700 spectrophotometer at 500 nm (Absto), The solution was left at rest for 1 hour and 3 mL of supernatant were extracted. Final absorbance (Abst60) was measured and the percentage of coagulant activity was calculated by Equation (1).
Coagulating substances were aluminum sulfate, starch, pectin and the three solutions of the tamarind seed extract applied at 1 % and 5 % (w/v) for the two types of synthetic turbid water of 10 and 280 NTU, respectively.
Jars Test: For the evaluation of coagulant activity, coagulant substances used at 1 % (w/v) solution were: aluminum sulfate, starch and tamarind seed extract in water, sodium chloride and ammonium acetate. For the evaluation of flocculant activity, the coagulant substance was aluminum sulfate at 1 % (w/v) in combination with 6 flocculation aids substances: PAM, starch, tamarind seed extract in water, sodium chloride and ammonium acetate were used at 0,1 %, while pectin was applied at 0,01 %.
Jars test of 6 places equipment OVAN and 1 L beakers was used. The test was carried out using the raw water sampled, based on the American Society for Testing Materials ASTM D2035-13 standard (ASTM, 2013), with the following modifications: flash mix at 100 rpm for 1 minute, slow mix at 40 rpm for 20 minutes and sedimentation for 30 minutes. The floc was monitored using the Willcomb index and the Water Research Association (WRA) size comparator.
Parameters measured immediately were color in a Hach DR2700 spectrophotometer and turbidity in a Hach 2100P turbidimeter to calculate the percentage of removal according to Equation (2), where X is the value of the parameter measured before (initial) and after (final) test.
Willcomb index was: 0 (colloidal floc), 2 (floc visible, but very small), 4 (dispersed, well-formed floc, evenly distributed, but slow settling), 6 (floc clearly distinguishable, well formed, relatively large, but precipitates slowly), 8 (good floc, which precipitates easily) and 10 (excellent floc sediments completely, leaving the water crystal clear.). The WRA size comparator was: A (0,3-0,5 mm), B (0,5-0,75 mm), C (0,75-1,0 mm), D (1,0-1,5 mm). E (1,5-2,25 mm), F (2,25-3,0 mm) and G (3,0-4,5 mm) (Arboleda, 2000).
Characterization of residual sludge and their toxicological effect
Characterization of the residual sludge: Quantification of the residual sludge was carried out using the gravimetric method suggested by the American Public Health Association (APHA) 2540 F (APHA, 2005). Physico-chemical and microbiological characterization of sludge was carried out using the following standardized assays: moisture, conductivity, total, fixed, volatile, suspended and dissolved solids, density, pH, COD, aluminum and coliforms (APHA, 2005).
Acute toxicity tests with Allium cepa L and Lactuca sativa L: Mineral water were used as negative control. Their chemical analysis was: 80,06 mg/L Ca2+, 144,85 mg/L Mg2+, 135,23 mg/L Na+, 9,31 K+, 1,5 mg/L SO4 2-, 174,7 mg/L Cl- and 932 mg/L dissolved solids. A dilution factor of 0,3 was used (100, 30,10, 3 and 1 %v/v).
Acute toxicity tests with Allium cepa L and Lactuca sativa L were carried out based on the procedure suggested by Romero and Cantu (2008) to calculate the percentage of growth inhibition and half maximal inhibitory concentration (IC50) by the Probit method and the inhibition of elongation and germination, respectively.
Results
Coagulant substances and flocculation aids
Table 1 summarizes the main results obtained in extraction of each polymer.
Starch yield was 1,47%, which is higher than the 0,69% reported by Nasrin, Noomhorm, and Anal (2015). The reason is that the waste processed in this work did not only contain peels (92,11 %), but also unused plantain and pulp (7,89%). Furthermore, another factor that could influence was the lower moisture value of the sample (88,53 %) compared with the average of 92,5% reported by Ilori, Adebusoye, lawal, and Awotiwon (2007).
Pectin yield was 3,44 % (13,34 % DM), which is lower than the 9,83 % (15,92 % DM) reported by Devia (2003) and Aina, Barau, Mamman, Zakari, and Haruna (2012). Nevertheless, reported values in the literature correspond to flavedo plus albedo. This result could occur because processed albedo sample had a higher moisture of 74,2 % compared with the average of 63,7 % reported by Devia (2003), in addition to a possible loss of sample in filtratration with cloth or the influence of the degree of maturation of the orange.
Tamarind powder yield was 43%, while moisture content was 41,81 %, which is a value higher than the 11,3 % reported by Bagula, Sonawane, and Arya (2015). The reason is that tamarind residues came from wet pulping process and the residues contained 89% seeds and 11 % fibers and lint. 75,3 % of the seeds were completely covered (or their great majority) and 24,7% were without peel (or partially covered).
From the chemical analysis performed to natural polymers extracted, the following results were obtained. The starch had a moisture of 17,83 % with a purity of 77,7 % on a wet basis (94,56% DM). Although this percentage is high, it is lower than the 98,86% (DM) reported by Nasrin et al. (2015). This is possibly due to a poor drying that resulted in a moisture of almost twice the average value of 9,12% reported in the literature. Pectin powder had moisture of 14,56 %, constituted essentially by carbohydrates (73,13%), ashes (7,46%) and protein (4,82%). Tamarind seed powder with a moisture of 8,07% was lower than the 11,4% reported by Bagula et al. (2015). It was constituted mainly by carbohydrates (71,54%) and protein (16,35%), which coincides with those reported of 72,2 % and 15 %, respectively.
Results of pH, Z potential and particle size of the natural polymers are presented in Table 2.
Data in Table 2 evidence that aqueous solutions of polymers showed acid character. Pectin was the polymer with the lowest value (2,82), due to the presence of free carboxylic groups (Paredes, Hernández and Cañizares, 2015). Starch solution presented a value of 4,75, which is within the range documented by Demiate, Dupuy, Huvenne, Cereda, and Wosiacki (2000) from 3,0 to 6,0. Tamarind powder presented an acid pH of 5,59. Agarwal, Bhuptawat, and Chaudhari (2006) determined that when the pH is below 6, the surface charge of the tamarind powder is positive and that above this value tamarind seed would have a net negative charge.
Natural water had shown negative charged particles with Z potential values of-14 to-30 mV(VaneandZang, 1997). It can be observed that the main mechanism of action of the natural polymers is sweeping action and not charge destabilization, due to their negative values. This indicates that polymer chains are also negatively charged (Ndabigengesere and Narasiah, 1995; Li, Zhou, Zhang, Wang, and Zhu, 2008).
The particle size could have an influence on the efficiency of coagulation/flocculation since the main action of polymers is sweeping, however, no correlation was found.
Figure 1 presents the IR spectra of (a) starch/potato commercial starch, (b) pectin/commercial pectin and (c) tamarind powder.
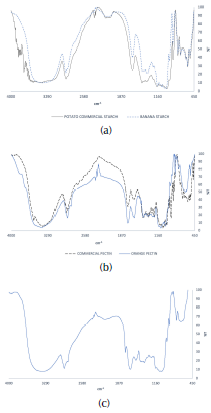
Source: Authors
Figure 1 IR spectra: (a) starch/potato commercial starch, (b) pectin / commercial pectin and (c) tamarind powder.
Starch FTIR spectra shows a broad band at 3 443,82 cm-1 corresponding to the OH stretching. The peak at 2 926,55 cm-1 indicates the C-H stretching, while the bands at 1 648 and 1 458 cm-1 are related to the O-H bendings of water and CH2, respectively. The bands from 764,98 to 1 174,18 cm-1 indicate the C-O bond stretching. The extracted sample shows same peaks in its FTIR spectra as the potato commercial starch (Xu, Kim, Hanna, and Nag, 2005; Bourtoom and Chinnan, 2008).
Pectin FTIR spectra shows a wide peak at 3 411,23 cm-1 dueto O-H vibrations, demonstrating that pectin is a polysaccharide. The band in 2 926,63 cm-1 corresponds to the stretch of the C-H of CH2 groups. The peaks between 2 000 and 2 300 cm-1 show uncharacteristic values, possibly indicating the presence of impurities. The bands that appear in 1 623,79 and 1 750,44 cm-1 indicate free carboxyl groups and carbonyls (C = O) of the ester and the acid. The peaks from 1 369,68 to 1 443,96 cm-1 correspond to the stretch vibration band of C-O-H. The peaks at 1 235,52 cm-1 show the asymmetric tension vibration band of the C-O-C and methoxy groups (-O-CH3). Finally, the presence of the C-O group is in the band between 1 000 and 1 100 cm-1 . In addition, the spectrum of pectin appears to be similar to that of commercial pectin, showing the same peaks at a similar wavelength (Chasquibol-Silva, Arroyo-Benites, and Morales-Gomero, 2008; Barreto, Pua, De Alba, and Pión, 2017).
In tamarind powder FTIR spectra, a band in 3 600 cm-1 reveals the existence of hydroxyl groups. The broad band in 3 000 to 3 200 cm-1 corresponds to amino and carboxylic acid groups. The bands in 1 653 and 1 541 cm-1 appear due to primary amine and nitro compound. Additionally, the peak in 1 064 cm-1 indicates primary alcohol (C-O) stretching (Patel and Vashi, 2010; Kaur, Jain and Tiwary, 2009; Pal, Sen, Mishra, Dey, and Jha, 2008).
SEM micrographics of (a) starch, (b) pectin and (c) tamarind powder are presented in Figure 2.
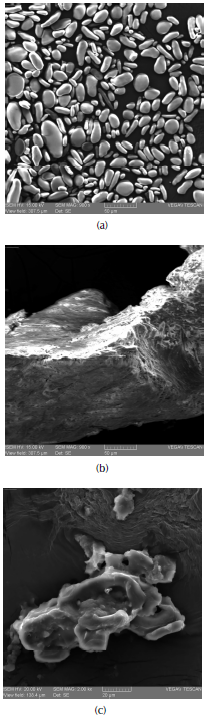
Source: Authors
Figure 2 SEM micrographics of polymers: (a) starch, (b) pectin and (c) tamarind powder.
Starch granules appeared with oval shape and different size, similar to that of potato starch (Hernández-Medina et al., 2008; Sujka and Jamroz, 2013). Pectine shows a porous, rough and irregular surface. Similar results have been presented by Mishra, Datt, and Banthia (2008) and Liu, Finkenstadt, Liu, Jin, Fishman, and Hicks (2007). Tamarind powder presents a porous surface, with irregular shape. This morphology have been reported in other studies (Patel and Vashi, 2010).
Evaluation of coagulant/flocculant activity
Narural water quality: Results of physico-chemical and microbiological analysis of natural water are summarized in Table 3. In general, raw water sampled quality for potabilization was good (color between 20-150 u Pt-Co, BOD5 between 1,5-2,5 mg/L) to excellent (pH between 68,5, turbidity between 0-10 NTU, coliforms between 50-100 NMP/100 mL), according to Silva (2015).
Due to coliform and color values, a conventional treatment was required according to the Texto Unificado de Legislación Secundaria de Medio Ambiente - TULSMA (Libro VI Anexo 1) (2015), to comply with the maximum permissible limits (MPL) for turbidity (5 NTU), color (15 u Pt-Co) and coliforms (< 1,1 NMP/100 mL) of the Norma Técnica Ecuatoriana del Instituto Ecuatoriano de Normalización - NTE INEN 1 108 (INEN, 2014).
Natural water analysis identified the presence of non-sedimentary solids (settleable solids < 0,1 mg/L), which are difficult to settle on their own. Alkalinity was low and it was a soft water (< 75 mg/L CaCO3) (Mihelcic and Zimmerman, 2012). Therefore, it could be concluded that coagulation/flocculation treatment was difficult in raw water sampled, mainly due to its low turbidity (higher dose of coagulant and sweeping action), in addition to low alkalinity.
Primary coagulant activity: The result was that coagulant substances have deficient or no coagulant activity, for low (10 NTU) and high (280 NTU) turbidity assay of starch, pectin, tamarind extract and even aluminum sulfate. For this reason, it is concluded that the assay did not yield reliable results, possibly due to errors when adapting the methodology.
Efficiency of coagulant activity: Pectin was not used as coagulant because it is hydrophilic and it has the same negative charge of particles that are intended to destabilize (Sánchez and Untiveros, 2004; Vaclavik and Christian, 2014). That is why it is difficult to use it as a coagulant, unless sweeping action is desired, which in this case is not possible due to the low turbidity of the raw water (5,32 NTU). Consequently, only the flocculant activity of pectin was analyzed.
Natural polymers had a poor turbidity removal (less than 1 %), possibly due to the their coagulation mechanism, which is mainly sweeping action as a result of molecular weight and polymer chains, and not necessarily the destabilization of charges. However, as the turbidity of the water is low, there are not enough particles to create the sweeping action (Mihelcic and Zimmerman, 2012).
In comparison, aluminum sulfate obtained a high removal of 86,0 % turbidity and 88,7% color, with an optimum dose of 65 mg/L. In addition, a final pH of 7,05 (15 0C) with a short floc formation time of 3 minutes indicate a rapid reaction rate. Floc was dispersed, well-formed and had slow sedimentation (Willcom index of 4) with a size between 0,5-0,75 mm (size WRA of B). The optimum pH for the dose of 65 mg/L of aluminum sulfate was between 7 and 8 units.
Although some natural polymers did not work appropriately as coagulants, they can be used as flocculation aids, as they showed turbidity and color removal up to 80 %. For example, M. oleifera seed extract is a polyelectrolyte, and it may be able to function as a flocculation aid, with an optimal dose of 10 mg/L in combination with 20 mg/L of alum (Bichi, 2013). Tannis has also been used as flocculation aid, achieving 96% in turbidity removal when used with alum (Ozacar and Sengíl, 2002).
Therefore, it was evidenced that natural polymers starch, pectin and tamarind seed extract did not have coagulant activity to real conditions of raw water, because of its low turbidity. However, their flocculating activity was evaluated as flocculation aids, using aluminum sulfate as coagulant.
Efficiency of flocculant activity: Optimal combinations of aluminum sulfate + polymers obtained equal or greater removal percentages of turbidity and color than those obtained using only this coagulant, with an evident reduction in consumption of aluminum sulfate. Likewise, the natural polymers presented the same or better characteristics of flocculation aids than commercial synthetic polymer PAM.
Optimal combinations of aluminum sulfate [mg/L] + polymer [mg/L] were: 50 + 0,2 for starch, 60 + 0,06 for pectin, 60 + 0,6 for tamarind in water, 60 + 0,5 for tamarind in sodium chloride, 60 + 0,2 for tamarind in ammonium acetate and 50 + 0,2 for PAM, as can be seen in Figure 3.
Compared with the 65 mg/L of aluminum sulfate used as coagulant without the addition of polymers, the use of flocculation aids allowed a saving of aluminum sulfate of 15 mg/L when using starch and PAM and of 5 mg/L when using pectin and tamarind extract in water, sodium chloride and ammonium acetate.
In general, optimal combinations of aluminum sulfate + polymers obtained a turbidity and color removal of around 88 and 92 %, respectively. Final pH was maintained between 7,0-7,1 and the formation time of the first floc was short, between 2 and 3 minutes, indicating a rapid reaction rate, as shown in Table 4.
Table 4 Main characteristics of natural water treatment when using aluminum sulfate and optimal combinations of aluminum sulfate + polymers
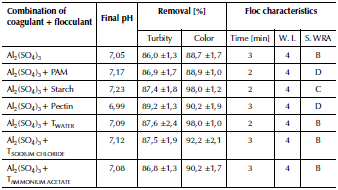
Source: Authors
There were differences in the size of the floc. The largest size obtained was 1,0-1,5 mm (T. WRA of D) when using pectin and PAM; followed by 0,75-1,0 mm (T. WRA of C) of starch; and, finally, a size of 0,5-0,75 mm (T. WRA of B) for the tamarind extract in water, sodium chloride and ammonium acetate. Among solutions in water, sodium chloride and ammonium acetate for the tamarind extract, there were no significant differences.
Feasibility of natural polymers used as flocculation aids. Combinations of aluminum sulfate + natural polymers obtained equal or higher removal percentages of turbidity and color than only using aluminum sulfate or aluminum sulfate + PAM, which showed the technical feasibility of their use. Social/environmental feasibility was observed, since the use of these polymers reduces consumption of aluminum sulfate, the possibility of generating residual aluminum and risks of adverse effects on humans health (Suay and Ballester, 2002). In addition, natural polymers compared to commercial synthetic polymer PAM have low toxicity, are safe for consumption and responsible with environment, reduce chemical dependency and reusing organic solid waste, no surface is needed to plantation. However, biodegradability in water must be studied in detail, because it can be a rich substrate for microorganisms that have not been completely removed (Choy et al., 2014). Economic feasibility was not observed, due to high extraction costs linked to low yield (1,47, 3,44 and 43%). Assuming a flow rate of 600 L/s for the same quality of raw water processed in this study during one day and considering exclusively the cost of the laboratory reagents, the most profitable options were the use of aluminum sulfate only or the combination of aluminum sulfate + PAM, with a saving of $ 115,11/day.
Clarified water quality: In general, clarified water presented a reduction in parameters of turbidity, color, conductivity, solids, pH, alkalinity, hardness and coliforms; while the settleable solids, COD and BOD5 remained practically stable.
Figure 4 shows that parameters of turbidity, color and coliforms had a removal greater than 85% and the rest of parameters obtained a reduction of less than 30%. In the case of polymers, a lower reduction in alkalinity was obtained than when using only aluminum sulfate. Final pH also did not have a marked reduction (10 %) and remained around 7,1. In this way, clarified water complied with the physical characteristics of drinking water regulations, with values lower than 5 NTU and 15 u Pt-Co, but not with parameter of coliforms, which can be removed through complementary filtration and disinfection operations.
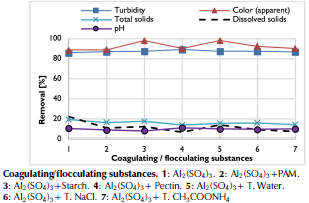
Source: Authors
Figure 4 Removal percentage of physico-chemical and microbiological parameters of clarified water.
Analysis of residual aluminum showed an increase with respect to the aluminum of raw water (0,1 mg/L). This increase was kept to margin of 0,2 mg/L normed by the Environmental Protection Agency (EPA) and the World Health Organization (WHO), except when only sulfate aluminum was used (0,21 mg/L) (Water Quality Association, 2013).
Toxicological evaluation of residual sludge
Quantification and characterization of sludges: Volume of residual sludge generated in each jar test was between 3 and 4 mL per liter of treated water, for both aluminum sulfate and combinations of aluminum sulfate + polymer.
Residual sludge moisture remained above 98%, which corroborates its poor capacity of dehydration (90 %moisture), as mentioned by Joshi and Shrivastava (2011). Although the density of the sludge is close to that of water, it is enough to allow it to settle; while the pH remained around 7.
From total solids, around 26% were volatile solids, thus the highest percentage is constituted by inorganic solids generated mainly by sediment flocs of aluminum sulfate. Likewise, values of COD (> 1 670 mg/L) and aluminum (> 275 mg/L) exceed values regulated by TULSMA (Libro VI Anexo 1) (2015) of 500 mg/L COD and 5 mg/L of aluminum and did not comply with the maximum permissible limits for the discharge to the sewer system.
Acute toxicity test with Allium cepa L: Acute toxicity test with Allium cepa L allowed to identify a toxic effect of residual sludge in cell division of radicular meristems by slowing mitotic activity and affecting normal elongation of onion root (Romero and Cantu, 2008). Average length of roots exposed to residual sludge was kept lower than 1,3 cm, which was lower than the length of 2,2 cm of roots exposed to negative control. This allows to state that the greater the dilution, the greater the length of the roots of each onion is. Inhibition percentage of roots length was between 40 and 86 %.
Calculation of IC50 was performed using Probit method and obtaining equations of the form Probit = a log (Concentration) + b. The values of R2 were between 0,92 and 0,98 indicating a good linear correlation, as shown in Figure 5. From the obtained equations, Probit equal to 5 (50% elongation inhibition) was calculated in order to obtain IC50.
IC50 values were 2,03% for aluminum sulfate, 2,73% for aluminum sulfate + starch, 1,5% for aluminum sulfate + pectin, 1,08% for aluminum sulfate + tamarind in water, 0,51 % for aluminum sulfate + tamarind in sodium chloride, 1,08 % for aluminum sulfate + tamarind in ammonium acetate and 0,61 % for aluminum sulfate + PAM.
This study did not contemplate a microscopic analysis of the toxic effect shown in mitotic activity by means of nucleolar alteration, activity of antioxidant enzymes and content of soluble proteins. However, there are studies that prove that aluminum is responsible for negatively influencing these variables, as indicated by Qin, Jiao, Zhang, Jiang and Liu (2010).
In summary, residual sludge had a genotoxic effect on onions roots, reaching the inhibition in growth of 50 % onions roots at low concentrations between 0,5 to 2 %.
Acute toxicity test with Lactuca sativa L: Acute toxicity test with Lactuca sativa L did not allow to identify a toxic effect in the inhibition of germination or elongation (radicle + hypocotyl), due to the high variability of results and superior elongation of seedlings exposed to residual sludges with respect to control elongation.
Variability of results was possibly due to an improper homogenization of the sample, which affected differently germination and growth of seeds of lettuce. Otherwise, higher elongation could be due to the fact that not only aluminum is found in the sludge, but also essential micronutrients such as magnesium, zinc, cadmium, calcium, among others. These micronutrients promote growth, as mentioned by Pavón, Pacheco and Cárdenas (2005), and mask the toxicity, although seeds adsorb aluminum and accumulate it as indicated by Panizza-de-León, Aldama-Ojeda, Chacalo-Hilu, Vaca-Mier, Grabinsky-Steider, Márquez-Herrera, and Durán-de-Bazua, (2008).
Inhibition percentage of elongation was less than 25 %, except for combinations of aluminum sulfate + starch (28,95%) and tamarind extract in sodium chloride (34,21 %). Inhibition percentage of elongation decreased when concentration of residual sludge decreased and it became zero for aluminum sulfate and combinations of aluminum sulfate + tamarind extract in water and ammonium acetate. Additionally, inhibition percentage in germination of seeds was on average low or none, as most of seeds germinated without problems; except for aluminum sulfate (1,67 to 11,67% inhibition) and combinations of aluminum sulfate + tamarind extract in sodium chloride and ammonium acetate (0,67 to 5,00% inhibition).
As no inhibition percentage in germination and elongation exceeded 12 and 35%, half maximal lethal concentration (LC50) or IC50 could not be determined. It was appreciated that the concentration at which no effect is observed (No Observed Effect Concentration, NOEC) and the lowest concentration at which the effect is observed (Lowest Observed Effect Concentration, LOEC) is around the concentrations of 1 and 3 % of residual sludge.
When natural coagulants are used alone in the coagulation process, sludges can be applied in agricultural soils. Feria-Díaz, Polo-Corrales, and Hernandez-Ramos (2016), studied the physico-chemical, nutritional and dangerous characteristics from a sludge obtained from coagulation of raw water with M. oleifera and found that the sludge appear as a soil improver, but not as a fertilizer, because of the deficiency of its macronutrients and fecal coliforms concentrations. Since the produced sludge cannot be disposed directly in the environment, some alternatives of treatment should be considered, e.g. the sludge could be used as partial substitute for clay in brick manufacturing. In the study carried out by Ramadan, Fouad, and Hassanain (2008), it was determined that 50 percent was the optimum sludge addition to produce brick from sludge-clay mixture. The sludge used in the study was from the clarification tanks where aluminum sulfate was used as coagulant. In addition, alum could be recovered from the sludge according to Boaventura, Duarte, and Almeida, (2000), who determined a recovery near 88% through an alkaline extraction process applied to a primary coagulation sludge. Actually, recovered alum can be used again as coagulant for the domestic or industrial wastewaters treatment, reaching about 70 % of COD removal in sewage and surpassing even the commercial coagulant (Ishikawa, Ueda, Okumura, Iida, and Baba, 2007). In the study conducted by Chu (1999), alum sludge was recycled using a chemical precipitation process to remove lead metal in wastewater and reached up to 94 % of efficiency.
Sludge allowed germination of the lettuce seeds, either because the mud also contains essential micronutrients or because the sludge is organic. This opens the possibility of using it as an organic fertilizer with controlled release of the main macronutrients: nitrogen, potassium and phosphorus, taking care of the aluminum sulfate concentration (Qin et al., 2010; Ferrari, Genena, and Lenhard, 2016). Likewise, it should be considered that the use of natural polymers could increase the organic load of the mud.
Therefore, natural polymers can be used for natural water treatment, since they are safe and eco-friendly. They generate a smaller quantity of sludge with higher nutritional content. In addition, the raw material is available locally. Since natural polymers do not consume alkalinity as alum, the use of chemicals for pH adjustments is not necessary. Natural coagulants are also non-corrosive, that is why costs of using natural coagulants are always reduced, compared to those of the traditional ones (Choy et al., 2014). The use of natural polymers also allows the elimination of possible negative impacts of synthetic polymers on human health, avoiding the appearance of residual monomers from manufacturing process and byproducts (Saranya et al., 2014; Ramesh and Gandhimathi, 2013).
Conclusion
Natural polymers extracted from waste materials: starch from plantain peels, pectin from orange peels and tamarind seed extract did not have coagulant activity to real conditions of raw water tested, due to its low turbidity (5,32 NTU). However, they presented equal or better characteristics as flocculation aids than commercial polymer PAM, with an average removal of turbidity, color and coliforms of 87%, 92 % and 96 %, respectively. In economic terms, natural polymers use was not viable. Additionally, residual sludge did not comply with the maximum permissible limits to discharge to sewage system and had a toxic effect on mitotic activity of onion roots, reaching an IC50 at low concentrations between 0,5 and 2 %.