Introduction
Increased involvement of renewable energy sources in distribution systems has grown in recent years as a response to environmental and energy policies, which has led to substantial changes in the traditional operation of power systems. Instead of big generators and extended transmission lines, now there are several small generators distributed around the loads in the distribution system. This new system configuration begets a new concept called 'microgrid'. According to the literature, a microgrid is an electricity distribution system containing generation and consumption that can operate in a controlled and coordinated way with the main grid (interconnected mode) or in islanded mode (Bollen et al., 2017a; CIGRE, 2018). Islanded mode implies that the microgrid can be disconnected and operated autonomously.
As described in Prabaakaran, Chitra, and Kumar (2013) and Kumar and Venkateshwarlu (2013), the increased penetration of non-linear loads and power electronic-interfaced distributed generation systems (DGs) can cause power quality (PQ) issues in microgrids. PQ issues in microgrids such as voltage magnitude variation (voltage band), voltage sags and swells, flicker, harmonics, unbalance, or real and reactive power problems have been analyzed in several papers in recent years. Figure 1 presents the number of publications related to microgrids and PQ studies based on the abstract and citation from the Scopus database.
Figure 1 also compares the number of papers that deal with microgrids (all topics), islanded microgrids (all topics), and PQ issues related to islanded microgrids in the time period between 2000 and 2020. Although the number of publications related to microgrids and islanded microgrids per year has increased (8 615 and 1 551 total papers up to 2020 respectively), it is evident that research on power quality issues on islanded microgrids is still scarce (219 papers up to 2020), thus representing 2,56% of all research about microgrids.
Additionally, Figure 2 shows the number of papers produced per country between 2005 and 2020. The countries with the highest publication of articles about islanded microgrids are the United States (454 papers), China (452 papers), and Iran (272 papers). The average ratio between publications analyzing PQ issues of islanded microgrids and the general issues of islanded microgrids is 11% (44 papers) for these three countries. Although countries such as Brazil have made less research (91 papers) than the leading countries, papers about power quality on islanded microgrids represent 31,8% of that country's total.
Research on power quality disturbances in islanded microgrids could be of great importance for countries such as Colombia due to two main aspects: first, Colombia has large non-interconnected areas that could hold potential for the implementation of remote islanded microgrids (López-García, Arango-Manrique, and Carvajal-Quintero, 2018); and second, in recent years, the government has developed policies to encourage the connection of renewable resources (UPME, 2014).
In this paper, several publications are classified and analyzed with the purpose of systematically identifying the impact of the most common PQ disturbances in microgrids in islanded mode, as well as the levels that can be reached for every disturbance and determining possible future research needs on this topic. Additionally, considering the fast growth of DGs with an electronic interface, this paper focuses only on microgrids containing non-inertial generation sources.
In the next section, the general characteristics of microgrids and their main elements are described. Subsequently, the reviewed papers are classified according to the PQ disturbances in islanded microgrids, and some comparative values for voltage and current harmonics, voltage variations, voltage unbalance, and frequency variations are given. Finally, relevant findings are summarized in a conclusion section.
General Characteristics of Microgrids
In Marnay et al. (2015), microgrids are classified into four types: customer microgrids, utility microgrids, isolated remote microgrids, and virtual microgrids. This classification is shown in Figure 3.
Customer microgrids are self-governed local distribution networks. Usually, their generation and consumption are downstream of a single point of common coupling (PCC). Customers own these microgrids; therefore, they can operate them relatively unrestricted by the network operator, but they usually cannot transfer (sell) energy to the distribution network.
Utility microgrids are technically similar to customer microgrids, but the main difference is that the utility owns the microgrid. Accordingly, customers must comply with existing utility regulation policies.
Isolated remote microgrids are similar to customer microgrids, but these grids are not able to operate while interconnected with the main grid.
Virtual microgrids cover DGs at multiple sites, but are centrally coordinated so that they can be presented to the power system as a single controlled entity.
For customer and utility microgrids, two operation modes are identified: the interconnected and the islanded modes. During interconnected mode, the main grid can improve their efficiency and reliability due to the integration of DGs near the consumers, as well as making them more resilient. On the other hand, the islanded mode supplies energy to the microgrids during main grid faults, but if this mode operates for a long time, reliability and power quality issues should be taken into consideration (Marnay et al., 2015; Sood and Abdelgawad, 2019).
PQ conditions are related to the main elements of microgrids, which are: DGs, loads, control systems, and energy storage systems.
DGs are the energy resources that feed the loads. These sources can be classified into equipment without inertia (100% electronic-based generators, such as photovoltaic systems and small wind turbines), or equipment with inertia (based on synchronous or asynchronous machines, e.g. mini-hydro generators). Microgrids with high amounts of generators without inertia may suffer from voltage and frequency stability problems. Moreover, photovoltaic systems and wind turbines are also sources of waveform distortion (Harmonics, interharmonics and supraharmonics) and other power quality disturbances (CIGRE, 2016).
Loads of residential, industrial, and commercial customers can be linear or non-linear devices. Nonlinear devices are those that draw a current waveform that is not proportional to the applied voltage. The most common non-linear loads are electronic equipment, like computers, compact fluorescent lamps, battery chargers, and adjustable frequency drives. Electronic loads are common sources of waveform distortion and flicker, which are the major power quality problems in distribution systems.
Control systems can be classified into three categories: i) control of the devices themselves (generators and/or loads); ii) control of the interaction between source and consumption (e.g. demand management); and iii) connection and disconnection schemes between the microgrid and the main grid. A control system has a great influence on the interaction between the elements of the microgrid, and, consequently, on the power quality status.
Energy storage systems (ESS) are a fundamental aspect of isolated microgrids, which allows the compensation of imbalances between generation and load to ensure the reliability of the energy supply. Storage options include batteries, flywheels, super (or ultra) capacitors, superconducting magnetic energy storage (SMES), compressed air, pumped hydroelectric, and thermal energy storage. Depending on the characteristics of the energy storage system, the behavior of the microgrid may vary.
Assessment of Power Quality Disturbances in AC Islanded Microgrids
The interaction between loads, DGs, and energy storage systems during islanded operations and transition states can lead to adverse impacts on the power quality of microgrid. A bibliographic review of the most common PQ disturbances studied in islanded microgrids during the last decade is shown in Figure 4. Some of the assessed PQ disturbances include harmonic and supraharmonic distortions, voltage variation as long-term and short-term flicker severity, voltage unbalance, and frequency variations. Given that the generation and load equipment are mainly electronic-based, voltage and current waveform could be affected.
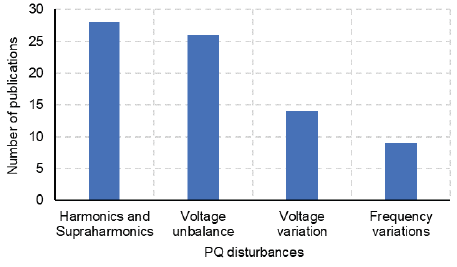
Source: Authors
Figure 4 Papers related to islanded microgrid between 2008 and 2018 per PQ disturbance.
Furthermore, higher volatility and stronger interactions between loads and distributed resources change the value of microgrid impedances in larger ranges with a tendency to weaken them, which will result in more pronounced, more frequent, and longer voltage variations, as well as higher susceptibility to PQ issues. In some cases, the microgrid can also present resonances at low frequencies, which might result in an undesirable increase of voltage harmonics (Bollen et al., 2017a, 2017b). According to Kaushal and Basak (2020), the effect of line impedance on power quality parameters is especially important when the microgrid's status changes from interconnected to islanded mode. As a result, voltage deviations are the main affected parameter.
In the next subsections, several studies on PQ issues in islanded microgrids are presented. The aim of these sections is to compare the PQ indexes of microgrids in interconnected and islanded modes and to identify which operation mode is more critical for the microgrid.
Harmonics and Supraharmonics
Waveform distortion is one of the most common electromagnetic disturbances in electrical systems. It is the deviation of voltages and currents from the ideal sine waveform. Periodic distorted voltages and currents are usually studied using the Fourier transform, which decomposes the original distorted signals into a linear combination of pure sinusoids at different frequencies. Using the Fourier transform, the total waveform distortion can be separated into:
Harmonics: spectral components with frequencies that are integer multiples of the fundamental frequency (50 Hz or 60 Hz in most electrical systems).
Interharmonics: spectral components with frequencies that are non-integer multiples of the fundamental frequency.
Subharmonics: spectral components with frequencies below the fundamental frequency.
Supraharmonics: spectral components in the frequency range between 2 and 150 kHz.
In order to assess those disturbances, indexes such as total harmonic distortion (THD) for voltage and current, and the individual magnitude for each harmonic are used. The THD is calculated as:
where U h is the magnitude of the harmonic component of order h, and U 1 is the magnitude of the fundamental component (component with the fundamental frequency). The THD can be also applied to current signals (THD¡).
Tenti, Paredes, and Mattavelli (2011); Padayattil, Thobias, Thomas, Sebastian, and Pathirikkat (2016); Agundis-Tinajero etal. (2018); and Garde, Casado, Santamaria, and Aguado (2015) report the THD u from simulations of different islanded microgrids with different load compositions (only non-linear loads, only linear loads, and a mix between linear and non-linear loads). These references show that microgrids with non-linear loads present higher THD u values than microgrids composed of only linear loads (see Table 1). According to Padayattil et al. (2016) the variation of the THD u values depends on the type of electronic-based generation and the amount of non-linear load connected.
Table 1 THDu(%) on islanded microgrid for different configuration generation - load
Generation | Load | THDu [%] |
---|---|---|
Electronic-based | Non-linear | 29,9 |
Linear | 3,48 to 15,76 | |
Mix | 3,57 to 41,67 |
Source: Authors based on references (Tenti et al., 2011; Padayattil et al., 2016; Agundis-Tinajero et al., 2018; Garde et al., 2015).
Some studies implement control strategies in order to reduce the THDu in those kinds of microgrids. Some of the proposed strategies include adding virtual impedance (Savaghebi, Shafiee, Vasquez, and Guerrero, 2015; Golsorkhi, Lu, Savaghebi, Vasquez, and Guerrero, 2016; Yang, Chen, Dong, and Han, 2015; Micallef, Apap, Spiteri-Staines, Guerrero, 2012; Blanco, Tardelli, Reigosa, Zanchetta, and Briz, 2019), harmonic controller (Zeng, Yi, Zhai, Zhuo, and Wang, 2017; Thai, Raisz, Monti, Ponci, and Ahmadifar 2018; Dehghani, Vahedi, Savaghebi, and Guerrero, 2012), compensations (Phan and Lee, 2019) Fuzzy-PI (Lhachimi, Sayouti, and Kouari, 2020) and artificial neural network (ANN) controllers (Kaushal and Basak, 2020). Table 2 shows the THDu variation of five islanded microgrids when different control strategies are implemented. In most of the cases, the THDu is reduced.
Table 2 THDu (%) on islanded microgrid for different configuration generation-load and different control strategies
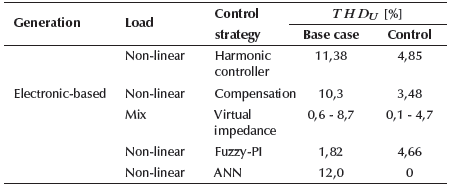
Source: Authors based on references (Golsorkhi et al., 2016; Yang et al., 2015; Micallefet al., 2012; Blanco et al., 2019; Zeng et al., 2017; Thai et al., 2018; Dehghani et al., 2012; Phan and Lee, 2019; Kaushal and Basak, 2020).
Additionally, some approaches, such as the one in Lhachimi et al. (2020), apply control strategies integrating IEEE standards in order to reduce the THDu levels in microgrids and accomplish regulation limits in both islanded and connected modes.
Studies by Laaksonen and Kauhaniemi (2008); Nomm, Ronnberg, and Bollen (2018a); Kulia, Molinas, Lundheim, and Fosso (2017); Zhou, Yuan, and Wang (2012); and Algaddafi, Brown, Gammon, Altuwayjiri, and Alghamdi (2016) discuss harmonic distortion in a microgrid when it operates in islanded and interconnected modes. A summary of the results is presented in Table 3 for the THDu index, Table 4 for the THD¡ index, and Table 5 for the first odd harmonic voltage magnitudes. For all the studied microgrid systems, generation sources are electronic-based and could include DGs and ESS. Additionally, four types of load are considered: non-linear load, linear load, motor, and mixed loads (linear and non-linear loads).
Table 3 Comparison of THDu (%) in interconnected (interc) and islanded (island) modes for different configuration generation - load
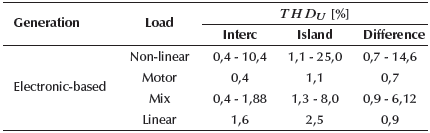
Source: Authors based on references (Laaksonen and Kauhaniemi, 2008; Nomm et al., 2018a; Kulia et al., 2017; Zhou et al., 2012; Algaddafi et al., 2016).
Table 4 Comparison of THD¡ (%) in interconnected (interc) and islanded (island) modes for different configuration generation - load.
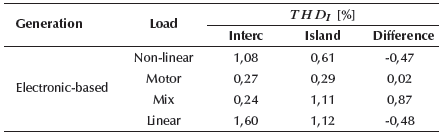
Source: Authors based on references (Laaksonen and Kauhaniemi, 2008; Nomm et al., 2018a; Kulia et al., 2017; Zhou et al., 2012; Algaddafi etal., 2016).
Table 5 Comparison of harmonic voltage (hv3 - hv15) in interconnected (interc) and islanded (island) modes for different configuration generation-load.
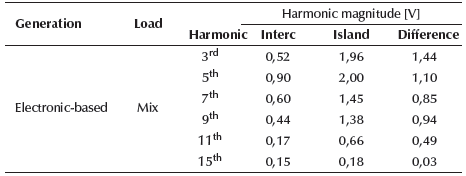
Source: Authors based on references (Nomm et al., 2019).
As shown in Table 3, THDu in islanded mode increases by up to 14,6% compared to the values in interconnected mode. Microgrids with only non-linear loads show the highest THDu values in both operation modes. According to Laaksonen and Kauhaniemi (2008), microgrid components such as filters and the switching frequency of converters directly affect harmonic distortion, especially if the load of the microgrids is completely non-linear.
Voltage distortion levels in islanded microgrids depend not only on the type of loads, but also on their size. In Hicks, Baghzouz, and Haddad (2018), THD y is 2,3% in a no-load condition islanded microgrid. When a non-linear load (980W) is connected, the THDu increases to 3,6%. However, when a larger linear load (1,9 kW) is connected to the microgrid, it reaches up to 6,9%.
In Table 4, the THD¡ values for interconnected and islanded modes are presented. Interestingly, THD¡ values not always increase during islanded mode. This is caused by the change in network impedance, which results in a different behavior between loads and generators. According to Laaksonen and Kauhaniemi (2008), Nomm et al. (2018a), and Zhou et al. (2012), when the microgrid has a mixed load, THD I values increase up to 0,87%, but if the load were completely linear, the THD I would have similar behavior or could even be reduced, as in the results obtained by Algaddafi, et al. (2016), in interconnected and islanded modes.
Another way to assess waveform distortions is through the harmonic voltage for each harmonic component. Table 5 presents the assessed harmonic voltage for the 3rd to the 15th harmonic. As a general result all harmonic components increase when the microgrid operates in islanded mode, up to 1,44 V (Nomm et al., 2019).
In Nomm et al. (2018a), THDu values are compared against regulatory limits. PQ standards as IEEE Std 519 (IEEE, 2014) and SS-EN 50160 (SEK, 2011) are applied. The study shows that, while in islanded mode, THDu exceeds the established limit, not only in magnitude but also in duration. This means that, within a 48-hour period, THDu values exceed the 8% limit for 10 hours. A comparison against compatibility levels provided in IEC 61000-2-2 as basis for EMC coordination was not performed.
Table 6 summarizes the maximum voltage magnitude in the supraharmonic frequency range interconnected and islanded operation mode in a microgrid with non-linear loads. Laaksonen and Kauhaniemi (2008) and Ronnberg et al. (2017) identified supraharmonics while in interconnected and islanded modes. Both studies show frequency components near the switching frequency (10-12 kHz) of the converters, thus jeopardizing microgrid operations due to possible resonances. Also, it is noted that during islanded operation, more components are present, and the magnitudes become higher by up to 3,5% compared to the interconnected mode.
Table 6 Comparison of the maximum harmonic magnitude (V) in the supraharmonic range in interconnected (interc) and islanded (island) modes for different configuration generation - load.
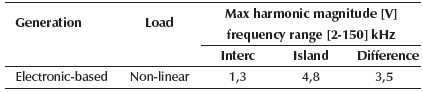
Source: Authors based on references (Laaksonen and Kauhaniemi, 2008; Ronnberg et al., 2017).
Voltage variations
The most common indexes to assess fast voltage variations include short-term flicker severity (P ST ), long-term flicker severity (P LT ), and the 3-second very short variations index (Ronnberg et al., 2017; Nomm et al., 2019), while slow voltage variations are usually quantified by the variation of 10 min values. Tables 7, 8, and 9 show the maximum value for each index when the microgrid operates in interconnected and islanded modes.
Table 7 Comparison of maximum P ST value in interconnected (interc) and islanded (island) modes for different configuration generation - load
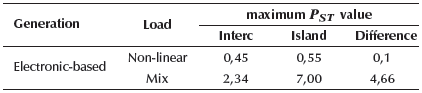
Source: Authors based on references (Ronnberg et al., 2017; Nomm et al., 2019).
Table 8 Comparison of P LT value in interconnected (interc) and islanded (island) modes for different configuration generation - load

Source: Authors based on references (Ronnberg et al., 2017; Nomm et al., 2019).
Table 9 Comparison of maximum very short variations (10 minutes and 3 seconds) in interconnected (interc) and islanded (island) modes for different configuration generation - load.

Source: Authors based on references (Ronnberg et al., 2017; Nomm et al., 2019).
According to Tables 7 and 8, higher values for short-term and long-term flicker were observed while in islanded mode. The P ST can increase up to 4,66 and, once again, the increase depends on the type of loads.
The results for 10-min variations and 3-second very short variations (VSV) obtained by Nomm et al. (2019) show that the 10-minute VSV values are less severe during islanded operation (see Table 9). However, the 3-second VSV values reach higher maximums during islanded operation. The possible cause of the 3s very short variations in islanded mode is the switching behavior of inverter operation due to solar irradiance variation.
In Ronnberg et al. (2017) and Nomm et al. (2019), microgrid measurements in interconnected and islanded operation modes are compared against regulatory limits, namely the values proposed in IEEE Std 519 (IEEE, 2014) or EN 50160 (SEK, 2011). For Ronnberg et al. (2017), voltage variation indexes are below regulatory limits, but Nomm et al. (2019) states that P LT values exceed the EN 50160 limit for a maximum of three weeks during a complete year. Both studies measured the same type of loads but received different results. For that reason, it is important to develop further and systematic measurement studies.
Voltage unbalance
The studies presented in Ronnberg et al. (2017) and Nomm et al. (2019) show that voltage unbalance in islanded microgrids is higher than in the interconnected mode. The results of both studies are summarized in Table 10.
Table 10 Comparison of the maximum voltage unbalance (%) in interconnected (interc) and islanded (island) modes for different configuration generation - load
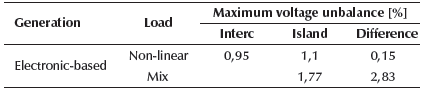
Source: Authors based on references (Ronnberg et al., 2017; Nomm et al., 2019).
Two operational modes, A and B, in islanded operation are studied in Nomm et al. (2019), depending on their short circuit impedance: mode A fluctuates between 0,1 and 1,0 Ω; and mode B between 0,1 and 2,0 Ω. Voltage unbalance is higher in operational mode B than in mode A with an increase of up to 2 times the values while in mode A. The same analysis for the other PQ parameters, namely voltage variation and harmonic distortion, showed similar results.
Frequency variations
Due to the interactions between loads and DGs, frequency variations in an islanded microgrid can be more pronounced, more frequent, and longer (Bollen et al., 2017b). For example, in Nomm et al. (2018b) measurements were performed in a microgrid for 48 weeks. As a result, 89% of the frequency variations were outside the 42,5-57,5 Hz band defined in EN 50160 (SEK, 2011) for islanded grids. Consequently, short interruptions occurred commonly, which might have some adverse effects on certain equipment.
In a network with high DG penetration, some of the DGs may be disconnected from it after a fault. During periods of high DG generation, this might result in local variations in system frequency. This is an evolving issue, and current distribution systems are not necessarily equipped to properly support system frequency, but depend on the interconnected system for load-generation balance (Bollen et al., 2017b). Studies such as Rodrigues et al. (2020) propose a novel frequency control, that allows the frequency setpoint to change adaptively throughout the evolution of the microgrid's islanded operation.
Conclusions
This paper presents a comprehensive literature review on studies about PQ disturbances in islanded microgrids. An increasing interest in this topic has been identified, especially regarding interconnected microgrids. Although the number of studies on this topic grows, there are still no systematic studies that analyze the impact of the different microgrid elements on PQ levels.
In most of the reviewed papers, the PQ of microgrids becomes worse during islanded operation, especially voltage distortion (harmonics and supraharmonics), voltage unbalance, and the frequency variations; these seem to have the highest difference between the interconnected and islanded modes. However, there is no systematic study to generalize the results obtained from the papers, as some contradict others.
The increase or decrease of the power quality indexes is linked to the type of generators, type of loads, control schemes, and the presence of ESS, filters, and compensation devices. In most cases, generation units are electronic-based, and the loads are non-linear. It seems that this generation-load scenario results in high voltage and current disturbances. However, not all reviewed cases specify the exact type of generator nor the power and the types of the supplied loads, so it cannot be determined which generation-load combination produces the highest distortions.
Changes in microgrid operation mode, such as from interconnected to islanded, cause variations in network impedance. None of the reviewed references thoroughly analyze the variation in network impedance, their frequency dependent characteristics. Consequently, typical values for microgrids are still unknown. Network impedance analysis is indispensable to understand microgrid behavior and the interaction between devices, as well as to propose limits for power quality disturbances and EMC coordination.
Most of the reviewed papers based their analysis on simulations or small laboratory-based microgrids. There is a lack of studies based on measurements of real islanded microgrids.
Finally, current international standards have yet established a comprehensive framework for EMC coordination in islanded microgrids. However, defining the corresponding limits is an paramount to ensure the reliable operation of electrical devices in islanded microgrids without loss of performance or damage.