Introduction
Sulfate ions are contaminants found in high concentrations in natural waters, as well as in effluents from different industrial processes, including mining acid drainage, acid effluents from copper smelters, water recovered from tailings ponds, and solutions for hydrometallurgical processes (Guerrero-Flores, Uribe-Salas, Dávila-Pulido, and Flores-Alvarez, 2018; Runtti et al., 2017; Silva, Lima, and Leão, 2012). Sulfate ions are not toxic, and sulfur is necessary for several living beings. However, when their concentration in water for human consumption exceeds 600 mg.L-1, they cause cathartic effects resulting in the purgation of the digestive tract. According to the World Health Organization (WHO), the maximum tolerable level of sulfate ions in drinking water is 500 mg.L-1. Nevertheless, some countries have advised lower values, such as Brazil and the United States of America, both recommending 250 mg.L-1 (Fernando, Ashane, Ilankoon, Syed Tauqir, and Yellishetty, 2018; Silva and Rubio, 2011). In Chile, Standard NCH 409/1 establishes that the maximum limit of sulfate ions in water for human consumption must be 500 mg.L-1.
Sulfate ion are also the main contributors to the 'mineralization' of water, thus increasing the conductivity and potential corrosion of the receiving bodies. These anions promote corrosion and pickling in pipes, structures, and equipment, making it difficult to recycle and reuse these waters (Dou et al., 2017; Silva et al., 2012; Cao et al., 2011; Rui, Yuanfa, Xingguo, and Jianhua, 2011; Moret and Rubio, 2003).
The search for suitable treatment methods for the removal of sulfate ions has been the subject of study by different researchers. Among the broad range of methods studied, biological treatments with sulfate-reducing bacteria, membrane filtration (reverse osmosis and electrodialysis), electrocoagulation, processes based on sorption phenomena (ionic exchange and adsorption/bioadsorption), and chemical precipitation are found (Rumjit et al., 2021; Öztürk and Ekmekçi, 2020; Mamelkina, Tuunila, Sillänpää, and Häkkinen, 2019; Hwang and Jho, 2018; Arahman, Mulyati, Lubis, Takagi, and Matsuyama, 2017; Călinescu et al., 2016; Guimarães and Leão, 2014; Bai et al., 2013; Silva et al., 2012).
Regarding treatment costs, chemical precipitation is the least expensive technique, depending on the reagent used and the effluent physicochemical characteristics (Öztürk and Ekmekçi, 2020; Dou et al., 2017; Telles, Granhen, and Lenzi, 2009). However, it requires an extra operational cost for the generated sludge disposal (Barakat, 2011). According to Ao, Cao, Hong, Wu, and Wei (2020), granular activated carbon (GAC) and anion exchange resins have been widely studied to remove sulfate from industrial wastewater. Nevertheless, GAC has a low adsorption efficiency, while anion exchange resins are too expensive to be utilized in wastewater treatment. On the other hand, Fernando et al. (2018) explain that the costs associated with sulfate ion removal using filtration/membrane processes are markedly higher than chemical precipitation. The precipitating reagents traditionally used for the removal of sulfate ions are calcium carbonate and lime to obtain gypsum, barium salts (BaS, Ba(OH)2, or BaCO3) to form barium sulfate, calcium hydroxide, and aluminum hydroxide, thus producing ettringite (Ca6Al2(SO4)3(OH)12-26H2O) (Dou et al., 2017; Cadorin, Carissimi, and Rubio, 2007). However, the precipitation of sulfate ions to form gypsum is not efficient for effluents with concentrations below 1 800 - 2 000 mg.L-1 (Cadorin et al., 2007). The formation of barium sulfate (barite) is widely used because it is efficient for the removal of sulfate ions in a wide pH range, and the formed precipitate (BaSO4) has a low solubility. However, the cost of barium salts is high, and barium compounds are carcinogenic (Telles et al., 2009). The production of ettringite for the removal of sulfate ions also allows reducing their concentration to values permitted by environmental regulations. However, for effective removal, an alkaline pH is required (Silva et al., 2012).
Several researchers have proposed to use polyaluminum chloride (PAC) as an alternative reagent, instead of the traditional reagents used for the precipitation of sulfate ions (Silva, Cadorin, and Rubio, 2010; Gao, Zhou, Yue, Liu, and Wang, 2006). PAC can be produced by adding a base to aluminum chloride until the empirical formula of Al(OH)n.Cl3- n (with n from 1 to 2,5) is obtained (Shen and Dempsey, 1998). PAC is composed of several species of aluminum, and, according to the time it takes for them them to react with the ferron reagent, they are generally divided into Ala (a monomer that reacts with ferron in 1 minute), Alb (oligomers and polymers that react with ferron between 1 and 200 minutes), and Alc (colloidal aluminum species that do not react with ferron) (Shen and Dempsey, 1998; Zhao, Liu, Xu, and Ni, 2009). Studies carried out to analyze the interaction of PAC with sulfate showed that the latter forms soluble complexes with Ala species, while Alb and Alc species react with sulfate to form crystals and amorphous precipitates, respectively. The content of each of the Al species depends on the basicity or molar ratio [Al/OH] (B value). For values of B = 0, the species are distributed in 90,1% of Ala and 9,9% of Alb; and for values of B = 2,5, the species distribution changes to 7,4% of Ala, 57,5% of Alb, and 35,1% of Alc (Xu, Wang, and Tang, 2004).
For the removal of aluminum precipitates containing sulfate ions, different methods of solid/liquid separation can be used, for example, sedimentation and dissolved air flotation (DAF). However, flotation compared to sedimentation is a more effective method since it has a higher treatment capacity (40 m3.m-2.h-1), produces less sludge volume, and is efficientfor turbidity removal (Jung, Park, Han, and Kim, 2017; Bahadori, A., Zahedi, Zendehboudi, and Bahadori, M., 2013). DAF is a solid/liquid separation method in which microbubbles (with a diameter between 10 and 100 microns) adhere to the solid particles suspended in the liquid, forming aggregates with a density lower than water. Microbubbles are formed by the pressure reduction of a water flow previously saturated with pressurized air, generally above 3 bar (Kurama, Karagüzel, Mergan, and Çelik, 2010). In DAF, the removal of clots or flocs does not necessarily require that they be hydrophobized, given that the formation of aggregates between particles/microbubbles can occur through one of the following mechanisms (Rodrigues and Rubio, 2007): a) bubble entrapment or bubble capture within the flocs or formation of aerated flocs (this phenomenon occurs when microbubbles enter and remain within a floc); b) entrainment of aggregates or flocs by ascending microbubbles (this mechanism occurs when the flocs are loaded onto the microbubbles or the ascending microbubbles remain below a descending floc).
This article reports the results of studies carried out for the removal of sulfate ions from synthetic effluents using PAC as a precipitating reagent and dissolved air flotation for solid/liquid separation.
Experimentation
Materials
A synthetic solution with a concentration of 1 800 mg.L-1 of sulfate ions was prepared by dissolving Merck sodium sulfate (NaSO4) (99% purity) in demineralized water, which had been previously acidified at pH 2. The pH was adjusted with hydrochloric acid (HCl) or sodium hydroxide (NaOH), according to the need of each test, and registered with a Thermo Scientific Orion Star A-series pH-meter. For the precipitation of sulfate ions, aluminum polychloride (PAC ECO-2300) from Ecofast S.A. was used, as well as the ECO-2600, ECO-8065, ECO-5650, and ECO-5350M (cationic), ECO-6750, ECO- 6700B, ECO-6700 M (anionic), and ECO-300 (non-ionic) flocculants produced by the same brand, in order to increase the mechanical resistance of the clots (precipitates formed between the different species of aluminum and sulfate ions). The formed flocs were hydrophobized with sodium oleate. The sulfate ion concentration was determined by the gravimetric method by precipitation with barium chloride. The residual turbidity of the clarified effluent was measured with a HANNA model HI 98703 turbidity meter. The characterization of PAC clots in the absence and presence of sulfate ions was performed in the scanning electron microscope (SEM) from ZEISS, model EVOMA10, and in the Malvern Zeta-Meter 4.0 equipment.
The precipitation tests of sulfate ions with PAC were performed in a glass beaker (500 mL) arranged on top of a magnetic stirrer. The precipitates formed were subsequently separated from the liquid by vacuum filtration using Whatman No. 42 filter paper.
DAF equipment was used for the flotation tests and consisted of a 3 L capacity PVC saturator and a 2 L capacity acrylic flotation cell. The saturator and the flotation cell are connected via a valve that has a plate with a 1 mm diameter center hole. The air to be dissolved was supplied by a Schulz compressor, model 10 BR of 2HP, 200 L capacity, and 9,8 kgf.cm-2.
Preliminary DAF tests (not reported here) showed that it is not possible to completely remove the precipitates, even though they were hydrophobized. Through visual inspection, it was determined that the clarified liquid had small precipitates in suspension. Therefore, it was necessary to incorporate a previous flocculation stage to improve flotation efficiency.
The tests were performed in the Jar Test FC4S equipment to determine the type and dose of flocculant. The results of the flocculation tests showed that the ECO-6700B (anionic) and ECO-300 (non-ionic) flocculants, dosed at 11 mg.L-1, formed larger floccules (visual inspection) and had a higher sedimentation rate. Subsequently, DAF tests were performed with the two selected flocculants. The flocculation stage was carried out under fast and slow mixing conditions in the flotation cell itself, provided by a Stuart Scientific mechanical stirrer, model SS3.
Precipitation test
Chemical precipitation with PAC was utilized because industrial effluents contain, in addition to sulfate ions, colloidal and suspended solids. Different positively charged polymeric species are produced during PAC precipitation, which are adsorbed without difficulty on the anions and negatively charged particles, forming voluminous clots that can be separated efficiently from the effluent by flotation. In the precipitation tests, 300 mL of synthetic solution contaminated with 1 800 mg.L-1 of sulfate ions were used. The effect of pH in the range of 4 to 10 and the effect of the [PAC: sulfate ions] ratio from 1:1 to 9:1 was studied. Tests were performed to determine the effect of pH with a ratio of 8:1, as well as its effect at pH 4,5. In all tests, the artificial effluent contaminated with sulfate ions was agitated at 100 rpm for 8 min, the necessary time to form the precipitates (precipitates formed between the different species of aluminum and the sulfate ions). An aliquot of supernatant liquid was collected and vacuum-filtered once the agitation was completed. The filtered liquid was subjected to chemical analysis to determine the residual concentration of sulfate ions.
Flotation test
DAF was used for solid/liquid separation due to the following advantages on sedimentation and filtration: low operating costs, high pollutant removal efficiency, and high treatment capacity.
Once the optimum parameters for the precipitation stage were defined, flocculation tests were performed to select the type of flocculant (anionic or non-ionic) that would produce the least turbidity in the clarified effluent. Subsequently, DAF studies were carried out to determine the optimum sodium oleate collector dosage from 0 to 25 mg.L-1 and the percentage of water saturated with air fed to the flotation cell (% recycling) from 10 to 30%.
The applied methodology was the following: a) the saturator was loaded with 2,25 L of drinking water (75% of the useful volume of the saturator), and 10 L.min-1 of air was dissolved during 3 600 s at a constant pressure of 4 kgf.cm-2; b) the polymeric species precipitates of aluminum and sulfate ions were formed applying the optimal conditions reached in the precipitation studies (pH 4,5 and [PAC: sulfate ions] ratio equal to 8:1); c) the solution was transferred to the flotation cell, and the clots were flocculated by applying 11 mg.L-1 of flocculant (determined in tests performed by Jar Test equipment) under fast mixing conditions (120 rpm) during 180 s (the speed was later reduced to slow mixing conditions of 30 rpm for 1 200 s); d) subsequently, the formed flocs were hydrophobized by applying sodium oleate; e) once the flocs were hydrophobized, the depressurization valve that feeds the flotation cell was opened, and 60 mL of air saturated with water (20% recycling) were added.
After 10 minutes of flotation, the time required for the ascent of the floc/microbubble aggregates of air to the top of the flotation cell, a sample of the clarified effluent was the bottom of said cell to determine the residual turbidity or residual sulfate ion concentration.
Results and discussion
Precipitation of sulfate ions with PAC
Effects of pH:Figure 1 shows the effect of pH on sulfate ion removal via precipitation using a [PAC: sulfate ions] ratio of 8:1. The Figure shows that, at pH 4,5, the concentration of sulfate ions is reduced from 1 800 mg.L-1 to 335 mg.L-1, which is equivalent to eliminating 81,4% (precipitating together with the colloidal species of PAC). Silva et al. (2010) and Xu et al. (2004) reported that, at pH 4,5, a higher proportion of sulfate ions is precipitated since Alb the is predominant species. The optimal pH range for Alb formation fluctuates between 3,5 and 5. In this pH range, the other aluminum species (Ala or Alc) tend to hydrolyze and transform into Alb, and the Alb content increases rapidly with the increment in the molar ratio [OH: Al] (Huang, Tang, Wang, D., Wang, S., and Deng, 2006).
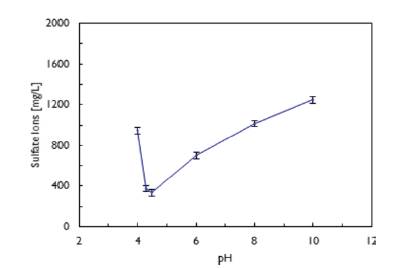
Source: Authors
Figure 1 Effect of pH on sulfate ion removal using co-precipitation using a [PAC: sulfate ions] ratio of 8:1.
Studies carried out by Wang, Sun, Xu, Tang, and Gregory (2004) showed that the percentage of Ala increases in the acidic region when the pH decreases, and in the alkaline region when the pH increases, which would explain why the percentage of sulfate ion removal decreases outside the pH range of 4 to 5.
Effect of the [PAC: sulfate ions] ratio in the precipitation of sulfate ions: In Figure 2, it can be seen that, by increasing the [PAC: sulfate ions] ratio, the final sulfate ion concentration in the filtered liquid decreases, whereas the sulfate ion concentration decreases from 1 800 mg.L-1 to 230 mg.L-1 when a ratio equal to 9:1 is used, which is equivalent to a removal percentage of 87%. Studies carried out by Silva et al. (2010) with different initial concentrations of sulfate ions, showed that their removal is a function of the [PAC: sulfate ions] ratio; as this ratio increased, the concentration of sulfate ions decreased. When they worked with a ratio of 8:1, the final concentration of sulfate ions was of the order of 500 mg.L-1 for an initial concentration of sulfate ions of 1 800 mg.L-1. According to these researchers, the efficiency of sulfate ion removal increases because the volume and size of the precipitates formed by PAC hydrolysis products increase as their concentration rises. Results reported by Amaral, Azevedo, Etchepare, and Rubio (2016) revealed that it is enough to use a [Al3+: S2 4 -] ratio equal to 4:1 to reduce the sulfate ion concentration from 1 800 to 500 mg.L-1.
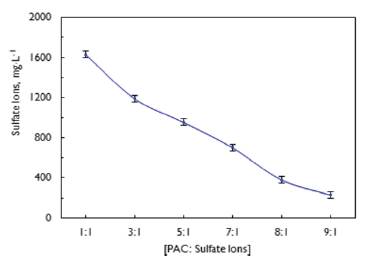
Source: Authors
Figure 2 Effect of the [PAC: sulfate ions] ratio on the final concentration of residual sulfate ions (initial sulfate ion concentration was equal to 1 800 mg.-1, and the pH was 4,5).
Morphological and chemical characterization of PAC precipitates and sulfate ions:Figure 3 shows the surface morphology of PAC precipitates in the presence of sulfate ions obtained from the Scanning Electronic Microscope (SEM) equipped to perform an Energy Dispersion Spectrometry (EDS) analysis of x-rays. The precipitates were formed from the co-precipitation of PAC and sulfate ions at pH 4,5 using a [PAC: sulfate ions] ratio equal to 8:1 and an initial concentration of sulfate ions equal to 1 800 mg.L-1. The Figure shows the area of a particle demarcated with a white outline, where the EDS analysis was conducted. The results of these analyses presented in Table 1 confirm that the precipitates are formed byaluminum, sulfur, sodium, chlorine, and oxygen from the PAC, Na2SO4, and NaOH reagents used. The iron traces probably come from external contamination and the carbon from the surface coating used to conduct the analyses.
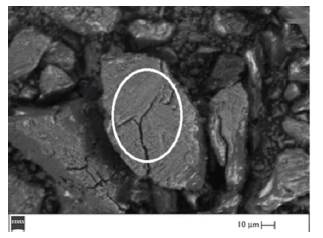
Source: Authors
Figure 3 Surface morphology of PAC precipitates in the presence of sulfate ions at a pH of 4,5 and a [PAC: sulfate ions] ratio of 8:1.
Table 1 Chemical analysis by EDS of PAC precipitates in the presence of sulfate ions

Note: The high carbon content in the analysis is due to the fact that the precipitates were deposited on a graphite conductive tape.
Source: Authors
Determination of the zeta potential: The zeta potential was determined as a pH function of the PAC precipitates in the presence and absence of sulfate ions to measure its effect on the electric double layer. As shown in Figure 4, at pH 4,5, the precipitates that were only formed with PAC have a zeta potential of the order of 40 mV, while the precipitates formed from PAC and sulfate ions have a zeta potential of the order of 29 mV. These values allow deducing that the sulfate ions are adsorbed on the precipitates formed with PAC, and the electric double layer is compressed as a consequence of this adsorption. The Figure also shows that the isoelectric point (0,0 mV) is obtained at pH 9 in the presence of sulfate ions, and pH 9,3 in their absence. According to Silva (2011), the alteration of the isoelectric point of the aluminum species as a function of sulfate ion adsorption reveals that the anion adsorption was partially specific on the internal Helmholtz level, which is of a chemical nature.
Dissolved Air Flotation
Flotation tests to select the type of flocculant: As noted, preliminary flotation tests showed that aluminum precipitates containing sulfate ions do not float completely. It was verified by visual inspection that the clarification contained a high proportion of suspended precipitates. The application of flocculants (anionic or non-ionic) allowed considerably reducing the number of suspended precipitates. The residual turbidity in the clarified liquid was significantly reduced with both flocculants, as shown in Table 2. The formation of large and porous floccules facilitates the trapping or capture of microbubbles, thus resulting in the formation of aerated floc-cules, which noticeably improves flotation efficiency (Olivera and Rubio, 2012). Different researchers reported that anionic and non-ionic flocculants are widely used to increase the size of micro-flocs formed with inorganic coagulants and facilitate their subsequent removal through sedimentation, flotation, or filtration techniques (Lee, Robinson, and Chong, 2014; Lee, Morad, Teng, and Poh, 2012; Verma, Dash, and Bhunia, 2012; Ahmad, Wong, Teng, and Zuhairi, 2008). The Table also shows that the non-ionic flocculant produces a clarified effluent with residual turbidity that is lower than the anionic flocculant (46 NTU, and 262 NTU, respectively). It was observed by visual inspection that the flocs formed with the non-ionic flocculant are larger than those formed by the anionic flocculant. This difference in size is possibly due to the different mechanisms of floc formation. According to Bratby (1980) for the case of anionic flocculants applied to colloidal dispersions destabilized by inorganic coagulants, the most suitable mechanism to explain the phenomenon that takes place during the formation of flocs is electrostatic patches, and for non-ionic or charged flocculants of the same sign as colloidal particles, the most suitable mechanism is polymer bridges. One of the main characteristics of this mechanism is the formation of flocs that are more resistant and larger than those generated by the electrostatic patch mechanism (Farrokhpay and Filippov, 2017; Gregory and Baranay, 2011). AtpH 4,5, amorphous aluminum precipitates containing sulfate ions have a positive charge (+29 mV).
Table 2 DAF tests used to select the type of flocculant
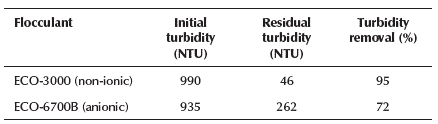
Note: The initial concentration of sulfate ions was 1 800 mg.L-1, the [PAC: sulfate ions] ratio was equal to 8:1, the pH was 4,5, the flocculent dose was 11 mg.L-1, the saturation pressure for DAF was 4 kgf.cm-2, and the recycle ratio was equal to 20%.
Source: Authors
Effect of the water flow saturated with air (% recycling):Figure 5 shows the results of the percentage effect of the water saturated with air fed to the flotation cell. The tests were performed using synthetic effluent contaminated with 1 800 mg.L-1 of sulfate ions, a [PAC: sulfate ions] ratio equal to 8:1, pH 4.5, ECO-3000 flocculant dose equal to 11 mg.L-1, and a saturation pressure in the FAD equal to 4 kgf.cm-2. As shown in the Figure, by increasing the percentage of recycling (water flow saturated with air fed to the flotation cell), the concentration of sulfate ions decreases. This decrease is probably due to the fact that the concentration of microbubbles and the air mass/solid mass ratio increase when increasing the volume of water saturated with air. Flotation efficiency increases when the number of microbubbles increases, due to the probability of collision, adhesion, and the capture of microbubbles in the porous structure of the flocs, thus decreasing their density and increasing their flotation velocity (Jung et al., 2017; Amaral etal., 2016; Ortiz-Oliveros, Flores-Espinosa, Jiménez-Domínguez, Jiménez-Moleón, and Cruz-González, 2012; Al-Shamrani, James, and Xiao, 2002). A removal rate of 70,3% was achieved when tests with 10% of recycling were performed, in comparison with the 81,1% achieved in tests with 30% of recycling.
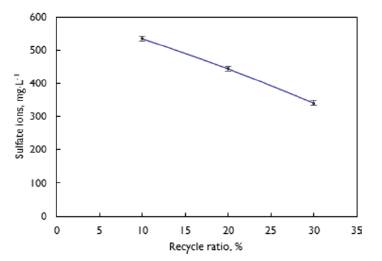
Note: The initial concentration of sulfate ions was 1 800 mg.L-1, the [PAC: sulfate ions] ratio was equal to 8:1, the pH value was 4,5, the dose of flocculant ECO-3000 was equal to 11 mg.L-1, the saturation pressure for DAF was 4 kgf.cm-2, and the recycling percentage was equal to 30%. Source: Authors
Figure 5 Effect of recycle ratio on the residual sulfate ion concentration in the clarified effluent in DAF tests.
Effect of sodium oleate concentration:Figure 6 shows the effect of the hydrophobization of flocs with sodium oleate on the sulfate ion removal percentage. The tests were performed under the same experimental conditions used to study the effect of the water flow saturated with air except for the recycle ratio, which was kept constant at 30%. The Figure shows that the application of the collector does not reveal a conclusive effect on the percentage of sulfate ion removal. The removal percentage remains at around 81% with and withoutthe addition of 10 mg.L-1, and the removal percentage decreases to 76 and 78% with the addition of 5 and 20 mg.L-1, respectively. Amaral etal. (2016) reported that the application of sodium oleate to hydrophobize flocs formed with PAC in the presence of sulfate ions does not modify the percentage of sulfate ion removal, remaining practically constant at around 80% for the different concentrations of collector applied.
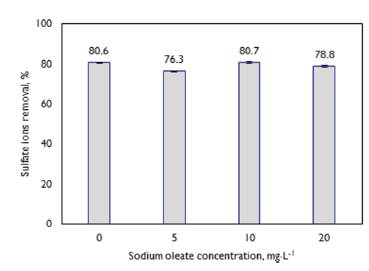
Note: The initial concentration of sulfate ions was 1 800 mg.L-1, the [PAC: sulfate ions] ratio was equal to 8:1, the pH value was 4,5, the dose of flocculant ECO-3000 was equal to 11 mg.L-1, the saturation pressure for DAF was 4 kgf.cm-2, and the recycling percentage was equal to 30%. Source: Authors
Figure 6 DAF tests to determine the effect of sodium oleate concentration on the percentage of sulfate ion removal.
Conclusions
The results of this study indicate that the co-precipitation of sulfate ions with polyaluminum chloride (PAC), followed by a flocculation stage with a non-ionic polymer and dissolved air flotation (DAF), proved to be efficient for the removal of sulfate ions from synthetic solutions.
Co-precipitation studies showed that sulfate ion precipitation is highly dependent on pH and the [PAC: sulfate ions] ratio. The concentration of sulfate ions is reduced from 1 800 to 335 mg.L-1 at pH 4,5 and with a ratio equal to 8:1. Higher or lower pH values increase their concentration, and higher values of the ratio decrease their concentration. Furthermore, the concentration of sulfate ions is reduced from 1 800 to 230 mg.L-1 at pH 4,5 and with a [PAC: sulfate ions] ratio equal to 9:1.
The results of the solid/liquid separation stage showed that, in order to efficiently remove the PAC precipitate containing sulfate ions, it is necessary to include a flocculation stage before flotation. The DAF studies showed that it is possible to reduce the concentration of sulfate ions from 1 800 to 350 mg.L-1 (80% removal) when solutions contaminated with sulfate ions were treated under the aforementioned experimental conditions ([PAC: sulfate ions] ratio equal to 8:1, pH 4,5, a non-ionic flocculant dose equal to 11 mg.L-1, saturation pressure equal to 4 atm, and 30% recycle ratio in the DAF).