Introduction
Metals are considered to be one of the major pollutants of water resources around the world. They are used in various industrial processes, and several tons of waste per year end up in effluents that reach different water sources (Chakraborty et al., 2020; Bilal et al., 2021). Metals are found naturally in the Earth's crust and are essential in several biochemical processes. However, some of them, such as cadmium (Cd), lead (Pb), mercury (Hg), chromium (Cr), and copper (Cu), are toxic even at low concentrations and have no known specific biological role (Ballardo de la Cruz et al., 2015; Beltrán-Pineda and Gómez-Rodríguez, 2016; Mustapha and Halimoon, 2015). Metal toxicity leads to biological and chemical degradation within ecosystems, affecting environmental and public health (Suárez and Reyes, 2002; Bilal et al., 2021).
Pb is a persistent pollutant of environmental interest. It is biomagnified through the different links in the food chain and causes long-term damage (Bedoya-Vélez et al., 2019). It inhibits enzymatic activity and affects nucleic acid and protein conformation, as well as cell membrane functions (Jaros+awiecka and Piotrowska-Seget, 2014). It can replace metallic ions such as Zn, Ca, and Fe in enzymes that are important for microbial metabolism (Naik and Dubey, 2013). High concentrations of Pb have been found up to a value of 250 ppm (Jaros+awiecka and Piotrowska-Seget, 2014) in the effluents derived from industrial activities such as lead battery manufacturing, welding, metal refining, weapons manufacturing, the automotive industry, power plants, and waste incineration, among others (Fernández et al., 2020; Naik and Dubey, 2013).
On the other hand, Cr is a transition metal that is found in different oxidation states. The most common states are Cr (VI) and Cr (III). Hexavalent chromium, Cr (VI), is considered toxic and is soluble, but it is rare in nature given its high oxidation state. Trivalent chromium, Cr (III), is less mobile but is frequently present in soil and water samples (Cervantes et al., 2001; Mandina et al., 2013). For instance, concentrations of Cr (III) up to 450 ppm have been found in tanneries (Pinzón-Bedoya and Cardona-Tamayo, 2010), especially in small industries that lack physicochemical wastewater treatments. These levels exceed the maximum permissible limits established in national environmental legislation (Resolution 0631 of 2015) (Cardona-Guitiérrez et al., 2013). Cr (III) interacts electrostatically with anionic groups in DNA phosphates, producing DNA transcription errors and causing mutagenesis. Additionally, Cr (III) affects enzyme structures (Cervantes et al., 2001; Joutey et al., 2015; Viti et al., 2013).
The presence of toxic metals in water sources has driven the development of different water treatment methods. Conventional methods include membrane filtration, electrodialysis, reverse osmosis, nanofiltration, and ultrafiltration (Cervantes et al., 2001; Chakraborty et al., 2020; Joutey et al., 2015; Viti et al., 2013). However, electrochemical methods, ionic exchange, precipitation, and recovery by evaporation are expensive techniques and only remove 1 and 100 mg/L of metals (Özer and Özer, 2003). Some methods are costly due to equipment maintenance costs, and state-of-the-art technology is generally too expensive for companies that operate at a local scale. Thus, efforts have been made over the past several years to design alternative biotechnological processes that efficiently remove toxic metals and are easy to operate, widely available, and relatively cheap. One of the alternatives for industrial wastewater decontamination is the use of several types of biomass from plant-based agro-industrial waste as bio-adsorbents. Bioadsorption is defined as the ability to retain metallic ions (adsorbate) present in a liquid phase (solvent) on a solid surface (adsorbent) (Ballardo de la Cruz et al., 2015). For instance, elodea (Egeria densa, ELO) is a submerged freshwater plant that is found in lakes. This plant is able to take up nutrients from the body of water and perform photosynthesis even with low amounts of CO2 and light, which makes it highly efficient at colonizing different ecosystems, to the point where it has been deemed an invasive species in many bodies of water (Rimac et al., 2018; Yarrow et al., 2009). Currently, there are few studies on the use of ELO for metal removal, but, in one study, it was able to remove nearly 91% of Pb (II) from an aqueous solution (Hernández-Gómez et al., 2017).
Other potential bio-adsorbents are coconut husk, saw dust-based biochar, and seed composite, which have been used to remove metallic ions from mining effluents and water bodies with an effectiveness between 75 and 96,36% (Fernández et al., 2020; Mahour et al., 2022; Manyuchi et al., 2022). Cassia fistula seeds and three different surfaces of modified adsorbent materials retained between 13,22 and 129,3 mg/g of Pb (II) at pH equal to 5,0 and 30 minutes of contact (Hemavathy et al., 2021). Orange peel (OP) has been used as a bioadsorbent for Pb, Fe, Cu, Zn, and Cr, with adsorption percentages between 67,2 and 99,5%, where pH and particle size play an important role in the metal retention of the adsorbent surface (Cardona-Guitiérrez et al., 2013; Fernández et al., 2020; Patiño-Saldivar et al., 2021). The removal of contaminants from solutions has also been investigated using tea waste, modified diatomite and bentonite, and the Metal Organic Framework (MOF) to adsorb dyes, metals, and pharmaceutical compounds. For example, carbon from tea waste was able to remove 120,13 mg/g of methylene blue, and UiO- 66 MOF adsorbed 30 mg/g of Cr (VI) and 485,4 mg/g of the pharmaceutical waste diclofenac sodium (Patil et al., 2022; Sriram et al., 2022a, 2022b; Rego, Sriram, et al., 2021a; Rego, Kuriya, et al., 2021b; Uthappa et al., 2020).
The use of biomaterials provides multiple benefits, as it repurposes solid waste, aids in the management of invasive species, and eliminates pollutants of environmental concern. Furthermore, bioadsorbents are efficient materials in the removal of metals and have a high biodegradation capacity, which is an advantage when they must be disposed of after use (Ojuederie and Babalola, 2017). When considering the use of biomaterials within water treatment systems, it is important to consider their biodegradation and stability in the design features.
During biodegradation, the materials' intrinsic biodegradability behaves as a function of time due to the occurrence of reactions dependent on the interaction with microorganism communities, temperature, and humidity, among other factors (Pischedda et al., 2019). Microorganisms responsible for the biodegradation process can be present in plant matrices, as well as in the effluents to be treated. Through different mechanisms, the microorganisms fragment biomaterials into simpler compounds that are necessary for their metabolic processes (Polman et al., 2019). During biodegradation, the carbon present in a material is transformed or mineralized, aerobically or anaerobically, into products such as biomass, water, carbon residues, and carbon dioxide (CO2), as shown in Equation (1) (Chinaglia et al., 2018).
Respiration involves an electron transfer chain from one carrier to another, in which organic compounds (bioadsorbents) act as electron donors to the final electron acceptor (O2). This chain yields adenosine triphosphate (ATP) and CO2, among other substances. ATP provides the necessary energy to produce molecular compounds involved in growth, maintenance, and reproduction (Vanrolleghem et al., 1999). Biomass is the result of long-term microbial reproduction (Chinaglia et al., 2018), while CO2 and H2O tend to be the final products of mineralization. Aerobic respiration generates the chemical energy used by microorganisms for their vital functions through the use of organic substrates as electron donors and oxygen as the last electron acceptor (Vanrolleghem et al., 1999).
Respirometry techniques can therefore be used to calculate the cumulative production of CO2 over time, using traps with alkaline solutions such as sodium hydroxide (NaOH) and potassium hydroxide (KOH). The amount of CO2 generated via microorganism respiration is quantified by titrating the quantity of base that has not reacted with carbon dioxide (Castro-Aguirre et al., 2017; Pellizzari-Wielewski et al., 2014). This provides a quantification of the materials' biodegradability, which is linked to the ability of organic materials to maintain their physical and chemical properties over time (or biostability). Inevitable changes in the adsorbents' structure lead to a loss of the material's active function (Lemaire, 1997), which highlights the importance of considering these two aspects (biodegradability and biostability) for the scaling of real polluted water treatment systems using biomaterials.
Additionally, bacteria, depending on their morphology, have different mechanisms that influence pollutant absorption (Verma et al., 2021 ). Gram-positive bacteria interact with metals at the extracellular level through electrostatic attraction (Joutey et al., 2015; Suárez and Reyes, 2002) to exopolysaccharides conformed of macromolecules as polysaccharides, proteins, and nucleic acids (Naik and Dubey, 2013; Sajna et al., 2021). Functional groups such as peptidoglycan carboxylate and teichoic acid phosphate, components of the cell wall, act as anionic sites that bond with the metal cations Pb (II) and Cr (III) (Diep et al., 2018; Pérez-Bou et al., 2018; Ojuederie and Babalola, 2017; Yin et al., 2019). Gram-negative bacteria also have mechanisms of extracellular interaction with metals via through electrostatic attraction (Joutey et al., 2015; Suárez and Reyes, 2002), in which functional groups such as phosphoryl in lipopolysaccharide and the components of the external membrane are responsible for the union with metal cations (Pb (II), Cr (III)) (Jaros+awiecka and Piotrowska-Seget, 2014; Kadukova, 2016; Naik and Dubey, 2013).
The biostability of bioadsorbents is a poorly studied but important aspect of the design of wastewater treatment with alternative materials. Thereupon, the objectives of this study were 1) to isolate native microorganisms that were tolerant of metals such as Pb (II) and Cr (III), 2) to determine the biostability and biodegradation of plant-based ELO and OP bioadsorbents, and 3) to evaluate the contribution of tolerant microorganisms to metal removal from aqueous solutions.
Materials and methods
Obtaining the bioadsorbents
Orange peel (OP) was obtained from a local market, and elodea (ELO) was collected from Simón Bolivar Metropolitan Park. Both plant-based materials were washed with abundant water and dried at room temperature. The bioadsorbents were chopped into pieces no larger than 1 cm2 and dried again at room temperature. Then, the bioadsorbents were dried in an oven at 50 °C to constant weight. Each of the plant-based materials was ground in a hand crank mill and sifted to obtain a particle size between 2 000 and 500 um. The bioadsorbents were then stored in sealed plastic bags at room temperature (Castañeda-Figueredo et al., 2022).
Microbial characterization of the bioadsorbents
Microbial characterization of the plant-based materials was performed in order to identify native microorganisms in each bioadsorbent and establish whether they contained microbial strains resistant to Pb (II) and Cr (III). The microorganism concentration in OP and ELO was quantified using the plate count method. Macroscopic morphological characterization (morphotypes) was based on the shape and color of the colony. Subsequently, a test of the microorganisms' resistance to metals in the bioadsorbents over time was done. In this assay, a Pb (II) and Cr (III) solution at 450 mg/L was added to OP and ELO. After 2, 600, and 1 104 hours of contact between the bioadsorbents and metallic ions, the microbial concentration was determined using serial dilutions (from 1x10-2 to 1x10-6) and cultures via the plate spread method. Bacteria and fungi were isolated with nutrient agar (NA) (Ben-David and Davidson, 2014) and Sabouraud agar or potato dextrose agar (PDA), respectively. Cultured agars were incubated at 30±1 °C for 24 hours for bacteria and at 25 °C for 3 to 5 days for fungi. All tests were performed in duplicate.
The morphotypes that were most dominant and tolerant of Pb (II) and Cr (III) were selected to be individually isolated in NA using the streaking method, and they were preserved at 4 °C.
Biodegradation and stability study of the bioadsorbents
An incubation test was used to determine the biodegradation of ELO and OP as a function of the microorganisms' metabolic activity by quantifying CO2 production (respirometry tests). The bio-adsorbents were incubated separately in hermetic glass jars with a 500 mL capacity. At the bottom of each jar, two smaller jars or vials were placed: one (amber) with 10 mL of sterile distilled water to maintain the humidity of the medium, and another one containing 2 g of dried bioadsorbent and 8 mL of a Pb (II) and Cr (III) solution at 450 mg/L (M1) (Figure 1). Controls consisted of 2 g of sterilized plant-based material and 8 mL of distilled water (M2), an empty glass jar (M3), and 8 mL of the Pb (II) and Cr (III) solution (M4). The CO2 that evolved during the incubation test was trapped with 20 mL of NaOH 1 N placed in 30-mL vials on the upper part of the jar. All setups were evaluated in duplicate. The M1 setup was incubated for 46 days for ELO and 60 days for OP. Control tests M2, M3, and M4 were analyzed three times during the assay (beginning of the test, middle of the test, and end of the test), according to the time established for each matrix. All incubations were performed at room temperature (20±1 °C).
The CO2 that evolved during the incubation test was quantified by mixing 2 mL of NaOH from each sample with 5 mL of barium chloride BaCl2 0,5 M. The NaOH that did not react to the CO2 was titrated with hydrochloric acid (HCl) 0,5N (Equations (2)-(4)).
The stability of bioadsorbents in terms of the product lifespan was established according to Leejarkpai et al. (2011)). The theoretical amount of CO2 (ThCO2) in grams was computed according to Equation (5), and the biodegradation percentage for ELO and OP was obtained from the produced amount of CO2, as shown in Equation (6):
where ThCO 2 is the theoretical amount of CO2 that each bio-adsorbent can produce (g); M TOT is the amount of dried solids (g) added at the beginning of the test; C TOT is the ratio of total organic carbon present in the samples' dried solids; 44 and 12 are the molecular weights of CO2 and carbon, respectively; (CO 2 ) T is the accumulated amount of CO2 (g) released from each setup; and (CO 2 ) B is the accumulated amount of CO2 from the blank (g).
Biostability was estimated through a zero-order kinetic equation, as shown in Equation (7) (Pinchao-Pinchao et al., 2019).
where ( B ) is the percent biodegradation at time t, ( B 0 ) is the initial percent biodegradation, and k 0 is the constant velocity.
Pb (II) and Cr (III) bioadsorption
The bioadsorption of Pb (II) and Cr (III) over time was evaluated in ELO with and without the predominant and metal-tolerant morphotypes (B1, B2, and B3). ELO was used for this test because it had more microorganisms than OP. The bioadsorption of Pb (II) and Cr (III) was tested using three treatments (T1, T2, and T3) in 100 mL Erlenmeyer flasks. Treatment 1 (T1) consisted of 1 g of sterilized ELO with 45 mL of a Pb (II) and Cr (III) solution at 50 mg/L, and an aliquot (5 mL) of each bacterium with a cell concentration of 0,550 of absorbance -approximately 5,8x108 cells according to the McFarland scale (EB1, EB2, and EB3). The bacteria were isolated separately through colony suspension in peptone water, followed by a reading in the spectrophotometer at a wavelength of 600 nm. Treatment 2 (T2) consisted of 1 g of sterilized bioadsorbent with 50 mL of the metal solution (MEL). Treatment 3 (T3) was prepared with an aliquot (5 mL) of each bacterium and 45 mL of the metal solution (MB1, MB2, and MB3). In T3, the nutrient broth was provided as a carbon source to support the bacteria's metabolism. All of the treatments were kept at room temperature (20±1 °C) under constant agitation at 120 rpm for 5 days. Every 12 hours, two tests were retrieved for each treatment, and the solution was filtered through 0,45 um membranes. The filtrates were preserved with nitric acid (HNO3) at 65%, and the metals were quantified via atomic absorption spectrophotometry. For each assay, a microbial growth control was performed which consisted of a streaking culture in AN and Sabouraud agar or PDA. The control was verified every 24 hours throughout the test. The adsorbed quantity and removal percentages were calculated using the following Equations:
where q t is the amount adsorbed (mg/kg), m 0 is the initial mass of the metal ions, and m f is the final mass of metal ions.
The adsorption kinetics of Pb (II) and Cr (III) in ELO were fit to two linear forms of kinetic reaction models (i.e., pseudo-first-order and pseudo-second-order equations) (Tran et al., 2017).
where q e is the amount of adsorbate uptake per mass of adsorbent at equilibrium, k 1 (1/time) is the rate constant of the pseudo-first-order equation, and k 2 (mass/mass x time) is the rate constant of the pseudo-second-order model.
Statistical analysis
A one-way ANOVA (analysis of variance) was used to determine whether there were any statistically significant differences between the means of Pb (II) and Cr (III) bioadsorption and the results of plant-based materials biodegradation. The normality, homogeneity, and multiple comparisons of means were evaluated with Shapiro-Wilk, Levene, and Tukey tests. All statistical analyses were performed with the OriginPro software and considered an alpha-value of 0,05.
Results and discussion
Microbial characterization of the bioadsorbents
ELO had a higher abundance of microorganisms than OP (Figure 2). Without metals, the ELO had 6,84x106 CFU/g of bacteria and 7,35x105 CFU/g of fungi and yeast. After 2 hours of contact with the Pb (II) and Cr (III) solution, there was an 80% decrease in both groups. In the absence of metals, OP had 5,50x102 CFU/g of bacteria and 1,00x103 CFU/g of fungi and yeast, and there was an 86,0% decrease in bacteria and an 84,3% decrease in fungi and yeast after 2 hours of contact with metals.
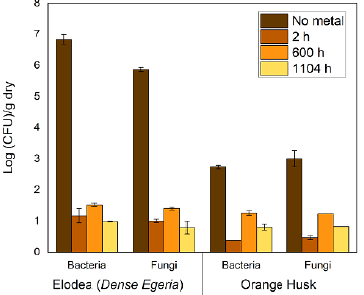
Source: Authors
Figure 2 Average count (log) for bacteria, fungi, and yeast in ELO and OP bioadsorbents in the presence and absence of Pb (II) and Cr (III) (450 mg/L)
Exposure to the Pb (II) and Cr (III) solution clearly inhibited microbial growth. After 2 hours of contact, toxic metals can disable cell enzymes, causing irreversible damage and modifying the active conformation of biological molecules (Beltrán-Pineda and Gómez-Rodríguez, 2015), leading to a decrease in the number of viable organisms.
Although the number of microorganisms decreased in the presence of metals over time, there was an apparent microbial adaptation in both bioadsorbents after 600 h of contact with the Pb (II) and Cr (III) solution. This demonstrates an active metabolism, in which the nutrients present in ELO and OP act as the main source of carbon and energy (Mehrotra et al., 2021 ). The easily biodegradable and available organic substrates stimulate microbial growth and respiration (Guerrero-Ortiz et al., 2012) since they are the source of carbon and nitrogen (Table 1) for microorganisms. Macronutrients used as a source of nitrogen are important elements for the synthesis of nucleic acids, phospholipids, protein, and stabilization of the cell wall in the microbial cell.
There was microbial growth in the presence of Pb (II) and Cr (III) over the full course of the test, possibly due to the development of tolerance and/or resistance mechanisms in the microorganisms. These aspects allowed the survival of several strains under the conditions of their environment (e.g., humidity, pH, temperature), including the presence of toxic elements (Yin et al., 2019; Muñoz-Silva et al., 2019). Based on the observed microbial growth in ELO over 60 days, three bacterial morphotypes were identified which were tolerant to Pb (II) and Cr (III). All three formed round colonies, which were pink (B1), yellow (B2), or white (B3). In the case of OP, there was little microbial growth compared to ELO.
With respect to fungi, there was mycelial growth with sporulation starting on day 19 for OP (with black pigments) and on day 25 for ELO (with white pigments and radial growth between 1 and 3 cm). The proliferation of fungi -especially those with morphologies with 1 to 3 cm of growth- can be problematic for filter design because they can plug filters, obstructing the flow of water to be treated. There may be viable alternative treatments using fungi that take advantage of their contaminant-adsorption properties (e.g., using pulverized biomass), but further investigation of those possibilities is needed.
Table 1 Nutrients present in ELO and OP on a dry basis are used by microorganisms as a source of carbon and other nutrients
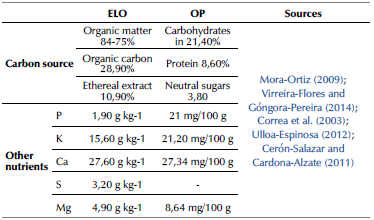
Biodegradation and biostability of the bioadsorbents
Measuring CO2 via respirometry tests is a reliable method for quantifying microorganisms' metabolic activity, as it measures microbial respiration, which is indispensable for the cell's vital functions. Figures 3a, 3b, and 3c show the CO2 evolution and microbial growth in the bioadsorbents ELO and OP over time, respectively. According to CO2 production, ELO presented the highest microbial activity. After 46 days of incubation, 1 439,9 mg of CO2 were found in M1 (ELO + metals at 450 mg/L), followed by M2 (ELO + sterile distilled water) with 114,4 mg of CO2. The control samples (M3 and M4) had a low production of CO2. As for OP, CO2 production was 114,4 mg in M1, 44 mg in M3, and 33 mg in M2 and M4 (Figures 3a and 3b) after 60 days of incubation. The amount of CO2 in ELO (M1) ranged between 200,75 and 870,65 mg from day 5 to day 19. Then, it showed high microbial growth, with bacterial counts of 106 up to 108. For OP, M1 showed an increase in CO2 from 11,0 mg to 544,5 mg between days 5 and 32 (Figure 3b). The values of CO2 for both bioadsorbents evidenced the active metabolism of bacteria, fungi, and yeast. Furthermore, there were bacteria present (3,6x103 CFU) in the sterilized (M2) ELO material, indicating the presence of high-temperature-resistant spores. However, by day 28, there was no microbial growth because no nutrients were included to allow their growth (Beltrán-Pineda and Gómez-Rodríguez, 2015). Meanwhile, OP did not show significant microbial growth on day 0. The behavior of CO2 over time is explained by bacterial growth, which was higher in ELO (Figure 3c).
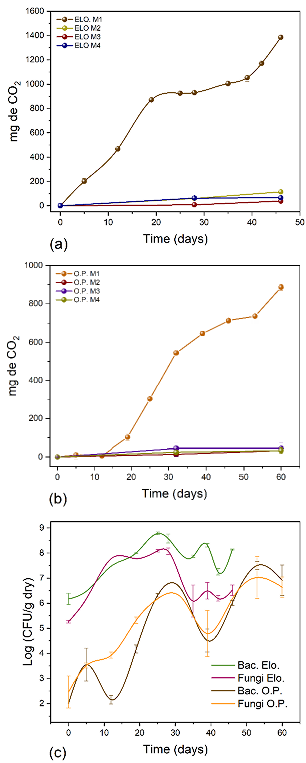
Source: Authors
Figure 3 Microbial growth (bacteria, fungi, and yeast). a), b) CO2 production from respirometry techniques in the presence of Pb (II) and Cr (III) at 450 mg/L; c) microbial growth in ELO and OP.
Guerrero-Ortiz et al. (2012) reported values of CO2 evolution of 649,44 mg over 30 days of incubation and 3 735,87 mg of accumulated C-CO2 from the biodegradation of organic fertilizers made from Lupinus montanus due to the material's biological activity. When evaluating rice chaff biodegradation under greenhouse conditions, Pedraza (2014) obtained values of 140 mg of CO2 and 233 mg of CO2 in an incubation period of 60 days. In this study, ELO was an easily biodegradable plant-based material in the presence of water. Furthermore, the amounts of microbial growth indicate an active metabolism, even in the MI treatment, in which they were exposed to high concentrations of Pb (II) and Cr (III) (450 mg/L), demonstrating that there were bacteria with tolerance and/or resistance mechanisms in both bioadsorbents (Bedoya et al., 2019). Such behavior is an indirect measure of metal degradation through catabolic reactions, as evaluated with respirometry techniques (Merchán et al., 2009). When ELO becomes saturated with the metal ions and therefore loses its bioadsorbent capacity, incineration is a suitable method of disposal.
When comparing the production of CO2 with microbial growth over time (Figure 3), there is a direct relationship with microbial development phases. There was an exponential phase between days 5 and 25 for ELO and between days 5 and 32 for OP (Figure 3a and 3b) during which the plant-based materials were easily biodegradable. This was followed by a stationary phase between days 25 and 30 for ELO and days 39 and 53 for OP in the CO2 production curve. Finally, there was a cell death phase. During the last few days of the test, there was a slight growth, since dead microbes can be a substrate for successive microbial populations (Pérez-Bou et al., 2018).
Figure 4 and Table 2 show the biodegradation of ELO and OP over time and their fit to a zero-order kinetic (Equation 7), respectively. Zero-order kinetics explain the biodegradation data of ELO by 92,74% and OP by 95,16%, according to the values of the coefficient of determination (R2). The product lifespan for ELO was 78 days or 2,6 months, and OP showed stability over 211,6 days or 7 months in the presence of the metal ions (Table 2). Biodegradation velocity for ELO is higher, which is possibly due to the absence of lignin in this plant's cell wall. On the other hand, OP can be regarded as a more biostable material in aqueous solutions due to the presence of lignin (3,2±0,4) (Cerón-Salazar and Cardona-Alzate, 2011). Lignin provides rigidity to the cell wall of the OP and is considered resistant to degradation, even more than cellulose (Polman et al., 2021). This enables a more complex structure in OP and a slower degradation (AbdelHamid et al., 2013; Pedraza, 2014). Pinchao-Pinchao et al. (2019) found biodegradation of natural fique (Furcraea andina) and cotton (Gossypium barbadense) fibers of 6,1 and 29,9 months, respectively.
Pb (II) and Cr (III) bioadsorption
ELO was chosen as the bioadsorbent in which to test the capacity of its associated microbes in order to remove Pb(II) and Cr(III) because it had more microbial growth, including strains that were tolerant to the metals of interest. According to Bojórquez et al. (2019), microbial population growth (i.e., increasing cell density) can increase the total availability of active sites for metal adsorption over time. Figure 5 shows the amount of Pb (II) and Cr (III) adsorbed in ELO with the presence (EB1, EB2, and EB3) and absence (MEL) of microorganisms, as well as the adsorbed quantity of metals by microorganisms (MB1, MB2, and MB3) over time. The adsorption kinetic data of Pb (II) and Cr (III) in ELO with and without microorganisms (EB1-EB3 and MEL) were well fitted by the linear form of the pseudo-second-order equation (R2 > 0,99). There is an affinity between bioadsorbent and metallic ions, which are attracted to the solid (Andrago, 2011) starting at 6 hours of contact. After 12 hours, small fluctuations occurred in the retention of Pb (II) and Cr (III) in ELO with and without microorganisms. At 12 hours, a state of equilibrium was reached between metals, ELO, and microorganisms. Hemavathy et al. (2021) reported that the pseudo-first-order equation fitted well to adsorption kinetic data of Pb (II) in Cassia fistula seeds. The pseudo-first-order model is only appropriate for the initial 20-30 minutes of the contact time between metal and bioadsorbent (Tran et al., 2017).
The adsorption capacities of Pb (II) at 120 hours of contact were 2 424,75, 2 257,25, 2 151,25, 152,25, 431,43, 115,88, and 2 256,88 mg/kg for EB1, EB2, EB3, MB1, MB2, MB3, and MEL, respectively. Moreover, the adsorption capacities of Cr (III) at 120 hours of contact were 2 273,63, 2 249,55, 2 264,80, 107,00, 85,95, 238,50, and 2 428,20 mg/kg for EB1, EB2, EB3, MB1, MB2, MB3, and MEL, respectively. These results showed that individually selected bacteria did not generate significant changes in metal concentrations, which limits their use for this purpose. ELO is a viable bioadsorbent for Pb (II) and Cr (III) removal, and it provides nutrients and substrate for microorganisms as well as metal adsorption sites. Martínez-Vásquez and Serna-Flórez (2020) studied the retention ability for Pb (II) and Cr (III) from adsorption kinetics with passion fruit rind (Passiflora edulis) and elodea (Egeria densa) in an aqueous solution. They found retention values for Pb (II) of 825,33 mg/kg and for Cr (III) of 666,43 mg/kg for passion fruit rind, and retention values for Pb (II) of 200,16 mg/kg and for Cr (III) of 114,83 mg/kg for elodea in an optimal time of 60 minutes. Hernández-Gómez et al. (2017) evaluated the bioadsorption ability of Pb (II) in synthetic solutions using elodea, pea pods, and coffee grounds as bioadsorbents. They found a greater efficiency in ion removal, with 91% for Elodea and 72,6% for pea pods.
The treatments EB1, EB2, and EB3 did not increase the removal of Cr (III) by the bioadsorbent. The bacteria labeled as EB1 increased Pb (II) adsorption in ELO (Table 3) with a p-value < 0,05. There was no significant metal removal by bacteria in treatment T3 (MB1, MB2, and MB3; p-value > 0,05). The treatment T3 showed the lowest adsorption of Pb (II) and Cr (III) in comparison with the MEL treatment (ELO showed high removal of metallic ions, 88,79-94,34%). Thus, the ELO-associated bacteria can persist in environments polluted with these metals, but they did not use Pb (II) and Cr (III) for their metabolic processes. This was further confirmed by growing cultures in nutrient agar to identify microbial growth for each treatment over time. For ELO treatments, there was growth (+), while, in the inoculated MB1, MB2, and MB3, growth was absent (-) (Table 3). This may be because, at a concentration of 50 mg/L, the metals saturated the cell membrane, disabling the metal resistance mechanisms (Jaros+awiecka and Piotrowska-Seget, 2014).
Conclusions
The study confirmed the potential of ELO and OP as bioadsorbents for metallic ion removal. Biostability and microbial characterizations demonstrated that OP had a longer lifespan than ELO (7,05 months vs. 2,61 months) and less microbial growth. Meanwhile, ELO contained a high abundance of native microorganisms that were resistant to Pb (II) and Cr (III). The abundant microbes in ELO degrade the plant-based material faster (higher CO2 production in the respirometry test). Furthermore, the microorganisms present in ELO did not contribute significantly to metal retention, meaning that it is mainly the plant material itself that is responsible for metal adsorption, except for the bacteria EB1, which led to a small but significant increase in Pb (II) adsorption. The pseudo-second-order model was observed as the appropriate fittest one for Pb (II) and Cr (III) adsorption in ELO. Finally, it is our opinion that OP is the better material for filter development to be applied in polluted water treatment systems.