Introduction
The production of electrical energy around the world is mainly related to the consumption of fossil fuels. Colombia, for example, is a country that depends to a great extent on the energy generated in thermoelectric plants. However, despite the fact that, over the years, efforts have been invested in the construction of power plants based on alternative energies, replacing the conventional coal routes has not yet been possible (Henao and Dyner, 2020). Additionally, situations such as those undergone by megaprojects such as Hidroituango (which, due to failures during the construction phase, is likely not to be completed) threaten the future security of Colombia's energy supply. Due to the above, and because Colombia has large coal reserves, the country continues to generate a good part of its electrical energy from this fossil fuel (Henao and Dyner, 2020; Vinascoa et al., 2014).
Most generation plants use pulverized coal for their production. However, some solid waste such as fly ash (FA), bottom ash (BA), boiler slag (BS), and flue gas desulfurization residues (FGD or synthetic gypsum) are discharged in large amounts when burning coal for energy.
These residues pose a potential risk to the environment if they are not treated or disposed of properly (Fytianos et al., 1998). Currently, fly ash (hereinafter FA) is the most abundant among the coal combustion products (Punshon et al., 2003). It is composed of fine particles that remain suspended in the airstream. FA makes up about 80% of the total ash produced in the aforementioned process; the remaining 20% is made up of the BA that fall and vitrify at the bottom of the furnace and is characterized by a coarser granulometry compared to FA (Asokan et al., 2015; Menéndez, et al., 2013; Siddique, 2010). Ashes can damage the environment and human health due to their heterogeneous composition, size, and physical and chemical properties (Jaramillo-Nieves et al., 2020; Wang et al., 2020). FA is susceptible to mixing with the environment due to its fine granulometry. Every year, coal-fired power plants produce between 600 and 800 million tons of FA around the world (Jayaranjan et al., 2014), out of which approximately 48% are recycled (Sushil and Batra, 2006). Some power generation plants choose to dispose of the FA in mounds and landfills, ignoring the harmful effects that this could cause: research has shown that landfill ash disposal activities are environmentally unsafe in the long term (Danker et al., 2011). The main dangers associated with FA arise from trace metals, metalloids, and potentially toxic concentrations with toxic effects on biota (Jackson et al., 2001). It is common to find traces of heavy metals (Cd, Cr, Zn, Pb) that can be leached into the water (Menéndez et al., 2013). Once dissolved, these spread to nearby lands, generating soil and water contamination. Additionally, they interfere with treatment and recycling methods on which several authors have been working (Lv et al., 2022). In soil improvement, for example, the physicochemical properties of FA and its alkaline nature make it a potential soil amendment, improving soil conditions and controlling its acidity. However, ashes represent a risk of contamination for the soil, plants, and groundwater due to its content of heavy metals (Pandey and Singh, 2010; Ram et al., 2006; Yao et al., 2015). In other treatment routes such as the manufacturing of cement mortars (Pedraza et al., 2015; Valderrama et al., 2011) and the production of geopolymers and hybrid materials (Chindaprasirt et al., 2014; Rivera et al., 2014; Martínez-López et al., 2015), their incorporation into ceramic pastes (Erol et al., 2008; Peng, 2004; Kockal, 2012), zeolite synthesis (Querol et al., 2002; Shigemoto and Hayshi, 1993; Murayama et al., 2002), and wastewater treatment can also cause interference due to contamination (Wang et al., 2020; Awoyemi et al., 2009; Dere Ozdemir and Piskin 2017; Tang et al., 2019; Wang et al., 2017). Although these elements are present in a relatively small fraction, they are of special interest due to their accumulation, long life, and high toxicity. Therefore, estimating their leaching potential is important to assess the possible environmental impacts associated with the reuse and disposal of FA (Fytianos et al., 1998)
Since the metal/metalloid ions contained in FA are not tightly bound, all of its heavy metals are susceptible to leaching to varying degrees. The aqueous phase extraction of these metals has been modestly studied, showing that AF leaching depends on several factors such as leaching time, temperature, pH of the medium, solid-liquid ratio, and FA source (Das et al., 2021 ).
However, FA leaching has been studied mainly for the recovery of trace metals from soft organic soils stabilized with this residue (Sauer et al., 2012; Das et al., 2021), as well as for the removal of impurities such as CaO and Fe2O3 with hydrochloric acid solutions at 80 °C under a liquid-solid ratio of 4,0 in 6,0 mol/L of acid (Lv et al., 2022).
Therefore, this study proposes an alternative for metallurgical use which allows for the valorization of this type of waste. This research analyzes the extraction of two of the heavy metals with the highest presence in the composition of an FA sample (Zn and Pb), through a process of agitation leaching. The experiments were carried out under ambient conditions, using three conventional inorganic acids (HCl, HNO3, and H2SO4), a salt (FeCl3), and a carboxylic agent (sodium citrate). This treatment aims to obtain a liquor rich in Zn and Pb for the subsequent recovery of metals through electro-recovery processes. On the other hand, a leached solid free of heavy metals is left to be applied as a secondary material.
Materials and methods
Materials
The FA sample used for the leaching experiments was obtained from a Colombian thermal power plant that uses mixtures of bituminous coal for its operation. The specific location, date of generation of the ashes, and their storage characteristics were not provided by the company.
Methods
Leaching methods and reactives: To carry out the leaching tests, conventional inorganic reagents were used, such as sulfuric acid (H2SO4), hydrochloric (HCl), and nitric acid (HNO3), as well as other alternatives such as ferric chloride (FeCl3) and sodium citrate (Na3C6H5O7.2H2O) at a concentration of 0,5 M. All solutions were prepared with JT Baker and Milli-Q deionized water. In some tests, it was necessary to modify the concentration of the solutions in order to determine its effect on the dissolution of the studied metals. The extraction process was carried out via stirring leaching (500 rpm), using mechanical stirrers without baffles (Model 50006-03, Cole-Parmer) with paddle-type propellers. An S/L ratio of 50 g of FA per liter of solution was used for 3 hours, at room temperature and pressure (1 022 bar; 17 °C). In citrate solutions, the pH was adjusted with the addition of sodium hydroxide (NaOH) and HNO3. The potential was monitored using a saturated Ag/ AgCl reference electrode (Oakton pH ORP 700 Benchtop Meter). Subsequently, the values were adjusted to the standard hydrogen electrode (SHE) for the corresponding thermodynamic analyses. Predominant area diagrams were elaborated using the MEDUSA software (Eriksson, 1979; Puigdomenech, 2004). The thermodynamic data of the metallic species in solution were contrasted with the NIST 46 database (NIST, 2004). The metal contents of the solutions were determined by microwave plasma atomic emission spectrophotometry (Agilent MP-AES), using the indications and calibration standards recommended by the manufacturer.
Sample characterization: The FA sample was chemically and mineralogically characterized. The chemical composition was determined by digestion with aqua regia (HCl: HNO3, 3:1). The results showed that iron was the most present element in the study sample (Table 1).
On the other hand, the content of trace elements such as Zn and Pb is due to their association with the inorganic fraction of coal, which, during its combustion process, vaporizes, condenses, and finally concentrates in fine ash particles in the form of oxides.
Mineralogical characterization was performed via X-ray diffraction (Panalytical X'pert Pro), using Bragg-Brentano geometry with a cobalt cathode. The ICDD database (International Center for Diffraction Data) made it possible to contrast the peaks shown in the diffraction pattern (Figure 1), which indicated that the FA was mainly composed of silica (SiO2) and alumina (Al2O3). The presence of these two was expected due to the dehydration suffered by kaolin in the coal combustion process. The iron content of the sample (Table 1) comes from both the pyrite (FeS2) inherent in the formation of the fuel and the iron oxide (Fe2O3), a product of the little oxidation of this sulfide during the combustion process:
Both the considerable presence of species such as SiO2 and Al2O3 and the absence of CaO in the FA sample indicate its silico-aluminous classification (F) (ASTM International, 2014), as well as the fact that the concentration of its relatively inert crystalline components is in a slow reaction phase, rich in iron and silica (Santaella, 2001).
Zn and Pb oxides are found in low concentrations, so they cannot be identified in the spectrum. However, it is possible to know its presence in FA due to its formation process:
Thermodynamic considerations
It was necessary to conduct a thermodynamic analysis for the carboxylic agent to identify the pH and potential conditions of the soluble and metallic species. The information provided by the prevalence diagrams indicates that, for Pb, at pH values 1 to 9, the formation of soluble species with potentials greater than -200 mV is favored. Under more alkaline conditions, the species formed are in a solid state. Zn, for its part, shows independence from the potential for species formation, while the favorable pH is within a range from 1 to 11. This can be seen in Figure 2.
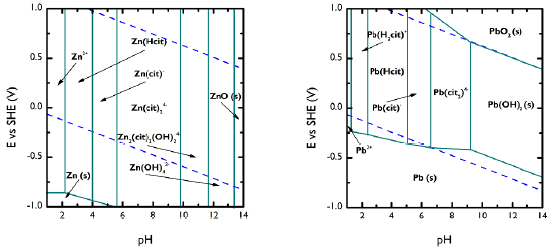
Source: Authors
Figure 2 Predominance diagrams for Zn (a) and Pb (b) in the presence of 0,5 M citrate. Designed with the MEDUSA software.
The above shows the citrate leaching ability of FA heavy metals across a wide pH range. Thus, three different values of pH were tested (4, 8, and 10) in order to determine their effect on the extraction of the metals of interest.
Results and discussion
Leaching tests
Leaching using HCl, HNO 3 , FeCl 3 and H 2 SO 4 : In hydrometallurgy, the capabilities of inorganic acids to chemically attack metallic elements is well known. Similarly, it has been reported that salts such as FeCl3 are favorable for eliminating heavy metals such as Zn and Pb (Guo et al., 2016). Figure 3 shows the performance of HCl, HNO3, H2SO4, and FeCl3 in metals extraction. Regarding Zn dissolution, it is evident that acidic media provide an adequate amount of hydronium ions, which is favorable for metal dissolution. However, the dissolution kinetics are different for the reagents tested. For the three acids, a greater rate of leaching was observed in the first hour, during which between 80 to 85% of Zn was recovered. The extraction with HCl and H2SO4 showed an asymptotic behavior, while HNO3 exhibited a progressive metals extraction of up to 98%. This can be attributed to the fact that, in the Zn-HCl and Zn-H2SO4 systems, the acid is quickly consumed, thus requiring a higher concentration of the solution to improve recovery.
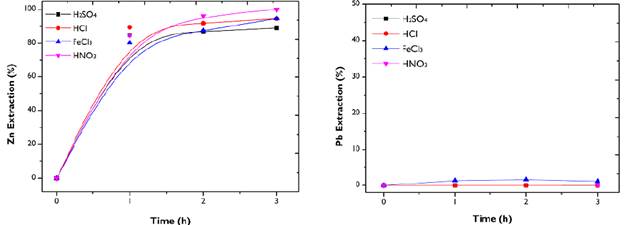
Source: Authors
Figure 3 Leaching of Zn and Pb from FA. Conditions: HCl, HNO3, FeCl3, and H2SO4- 0,5 M; 500 rpm; 50g/L.
On the other hand, the acidity of the chloride medium (pH 2,6) also allowed for a progressive recovery of the metal. However, after three hours, HNO3 was the one that achieved the best extraction of the non-ferrous metal. Due to the high presence of FeS2 in FA, the chemical systems of the three inorganic reagents have a lower lead leaching capacity. The presence of sulfur in the acidic medium gives way to the formation of solid-state sulfate species, which leads to the inhibition of the dissolution of the metal.
Pb chlorides resulting from leaching with FeCl3 are of low solubility, and their dissolution takes place at temperatures equal to or greater than 100 °C (Aguilar-Pérez et al., 1997). The low extraction of Pb with these agents is interesting, as it implies selectivity in leaching. Subsequent treatments can be proposed to detoxify the leached solid while obtaining a liquor rich in Zn.
Leaching using sodium-citrate
pH effect.: The influence of pH on the extraction of the metals of interest in the citrate solution was evaluated (Figure 4). With oxidation potentials in ranges from 400 to 600 mV that were recorded by the leaching tests, it was found that the dissolution of metals is independent of pH values.
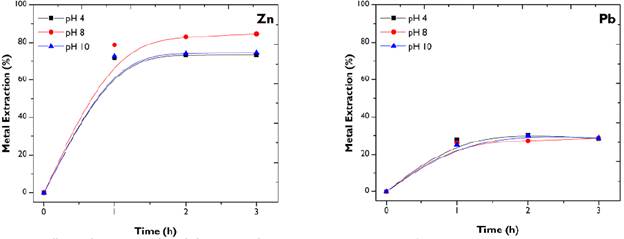
Source: Authors
Figure 4 pH effect on the extraction of metals from FA. Conditions: citrate 0,5 M; 500 rpm; 50g/L.
Likewise, it was observed that Pb is leached in the same way in the three media (acid, basic, or neutral). This characteristic creates the need for adequate FA treatment in order to avoid possible toxic effects on the environment caused by this heavy metal. Regarding Zn, tests at pH 8 indicate a slight improvement in metal dissolution, albeit only 6%. Due to economic and environmental factors, it is advisable to work under neutral conditions. Thus, a pH value of 8,0 was selected to continue the study.
Metal dissolution: The graphs indicate that the extraction of Zn and Pb combine the dissolution of two phases: the first corresponds to the spontaneous leaching of the oxides ZnO and PbO, while the second represents the slow dissolution of the sulfides inherent to coal, which fail to oxidize in the fuel combustion stage. For this reason, both Pb-Cit and Zn-Cit systems exhibit rapid dissolution kinetics, achieving beneficial extractions within the first hour. After this time, metal recovery becomes too slow (more for Zn at pH 4 and 10).
Sulfide leaching is more complex due to both their refractory nature and the formation of a passivating layer during the process (Santaella, 2001; Borda and Torres, 2022). Research has shown that it is possible to dissolve divalent Zn and Pb ions with citrate when these are associated with sulfides. However, additional time is necessary to obtain a better dissolution of the metals (Torres and Lapidus, 2020), as well as a higher molar concentration of citrate and the presence of hydrogen peroxide (Zárate et al., 2015). However, it has been shown that, in processes that combine the dissolution of two phases (in this study: oxides and sulfides), overall leaching kinetics can be affected (Borda et al., 2022).
Effect of citrate and HNO3 concentration
The degree of dissolution of metals was studied based on the variation of citrate and HNO3 concentrations from 0,5 to 0,1 M. The influence of the reagents' molarity was different for both systems (Figure 5).
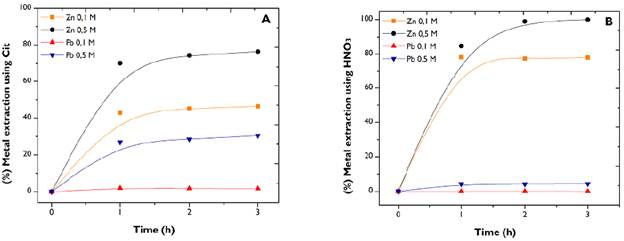
Source: Authors
Figure 5 Effect of citrate (a) and HN03 (b) concentration. Conditions: 500 rpm; 50g/L.
Although a greater metallic extraction was obtained when using the highest molarity of the agents, this occurred in different amounts and more noticeably in citrate. However, even though the reactions proceeded with an apparently concentration-dependent speed, the variation in the amount of species formed was not directly proportional to the agent's concentration, which is evidenced by the 28 and 26% increase in extraction with citrate and 17 and 4,3% with HNO3 for Zn and Pb, respectively. Although the metal recovery increases, it is not proportional to the increase in the molarity of the reactants.
The presence of leaching agents was higher than the available Zn2+ and Pb2+ ions. The concentration of the agents did not change appreciably over time, attributing a pseudo-first order to the chemical reactions taking place. Thus, the leaching kinetics did not reveal any dependence of rate concerning the concentration of citrate and HNO3 (Levenspiel, 1999; Smith, 1981).
Efficiency of Zn and Pb dissolution from solid leached with HNO3
HNO3 at 0,5 M achieved the highest Zn extraction (98%) compared to citrate under the same conditions (Figure 5). Note that the low dissolution of Pb is advantageous, as the selectivity towards Zn in the process facilitates its subsequent recovery. Leaching was required to detoxify the leached solid by removing the Pb from the residue. To this effect, the solution was filtered after three hours in order to subject the solid to a second leaching, this time using a fresh solution of sodium citrate with the initially established conditions (pH 8, 0,5 M).
The results show that the post-treatment reaches a 40% elimination of the Pb from the leached FA. As explained above, under these conditions, citrate can form soluble complexes only with the Pb ions present in oxide form. However, after previously extracting the Zn from the FA sample, the amount of reagent available to react with Pb sulfide compounds increases, which is reflected on a 10% improvement in metal removal in comparison with direct citrate leaching (Table 2).
Table 2 Efficiency of Zn and Pb dissolution from leached solid. Conditions: Leaching I with HNO3; Leaching II with citrate; 500 rpm; 30 g/L; 3 h.
Leaching I | Leaching II | |
Zn (%) | 98 | 98 |
Pb (%) | 0,04 | 39,45 |
Source: Authors
Iron extraction
The results of the chemical digestion revealed that the iron content in the study sample was 20,6%. Its presence in the FA sample is high compared to the percentage of trace elements such as Zn and Pb (Table 1). However, its extraction is not relevant in this study, as it is not considered as a contaminating or interfering element in the mechanical properties of the secondary material after FA treatment (Yao et al., 2015). The main issue with the extraction of iron lies the difficulty of its electrodeposition (Torres and Lapidus, 2017). Thus, in order to avoid drawbacks in the electrolytic recovery of Zn, it is preferable to minimize iron extraction.
Figure 6 shows the low dissolution of iron in the direct leaching with HNO3 and citrate, as well as the secondary leaching from the solid leached. The extracted metal content can come from the two iron phases present in FA: an easy to leach Fe2O3 and a somewhat more complex Fe2S (Figure 1). The fast iron extraction in the first hour is attributed to the dissolution of the oxide in the solutions, and, while the citrate leaching stops, HNO3 continues with very slow kinetics due to the dissolution of the sulfide species.
Conclusions
A method was presented to recover some heavy metals from thermal coal fly ash by a hydrometallurgical route under ambient conditions. Due to their characteristics, these metals can be potentially dangerous for the environment and extremely harmful for living beings. The results showed that inorganic acids and ferric chloride are more efficient for extracting Zn, as they achieve more than 90% recovery when compared to citrate. Their inefficiency in Pb recovery allows selective leaching of Zn. Two leaching stages are necessary to clean the residue: the first with HNO3 for Zn extraction and a subsequent one with citrate for Pb. It was shown that the dissolution of the oxide phases is fast, while that achieved by the sulfide phases is too slow and null in some cases.
This method achieves the removal of the Pb and Zn contained in the FA, yielding a residual product with non-toxic characteristics, which can be used as a secondary material.