Introduction
Chewing gum is a popular product around the world, and its consumption has been steadily increasing. Several studies claim that chewing gum has beneficial effects on oral health, weight control, and stress relief, among others, and it has even been proposed as an efficient mechanism for medication delivery (Takahashi et al., 2003; Jacobsen et al., 2004; Potineni, 2007; Thivya et al., 2021; Tijani et al., 2022). However, its insoluble fraction can not be digested by the consumer, and it is discarded to the environment as a waste. Around the world, about 250 000 tons of environmentally hazardous chewing gum waste are generated per year, according to Euromonitor International (Roy, 2021).
Chewing gum waste is an environmental problem, as currently available chewing gums are products made of substantially non-degradable elastomer (Roy, 2021). An important amount of chewed gum ends up on the ground, carrying harmful toxins and microorganisms (Saberi et al., 2018) that can severely affect the health of any living being upon contact or by accidental or systematic ingestion. All of this implies environmental and economic costs that the inhabitants and the planet must pay.
This waste also poses an economic problem. Cityauthorities and the people responsible for waste management must work hard to remove all of the chewed gum thrown to the environment. Such efforts are costly and not completely succesful. The removal of each bit of chewing gum thrown onto the pavement costs more than three times the average price of a piece of gum (Logan and Rudolfs, 1947; Oosthuizen et al., 2022). For example, in 2012, London spent more than £3 per piece of gum removed from the pavement, and three months steam-cleaning the 300000 pieces of gum that were thrown in an area of less than two miles of a street during the Olympic Games, while the consumer of such quantity of gum bought each piece for just a nickel, and threw that waste in a short period of time (BBC, 2010).
According to reports in The Telegraph (Rudgard, 2018) and The Guardian (Carter, 2005), governmental environmental protection entities of different countries charge taxes to the whole supply chain for single-use plastics (such as plastic bags) in order to diminish harms for the environment. It has already been considered that taxes to chewing gum consumption should be implemented as a way to recover some of the resources spent cleaning the gum waste.
Chewing gum waste also represents a social problem because it substantially decreases the utility and aesthetic value of public places (Simonek, 2011), generating untidiness and discomfort, as well as promoting adverse social behavior in the population. Among the tentative strategies to reduce the amount of chewing gum waste thrown onto the streets, some chewing gum waste collection campaigns have been proposed, as well as the use of improved cleaning methods (Liu and Jiang, 2003), the incorporation of anti-sticking agents into chewing gum formulations (Sozzi and Del Visco, 2011; Bullus, 2017), the production of bio-chewing Gum (Ribadeau-Dumas and Mentink, 2004; Palabiyik et al., 2018; Saberi et al., 2018), the making of wraps for their hygienic disposal (Simonek, 2011), and their use as a filling in the formulation of plastic blends useful for manufacturing chewing gum waste collection containers (Bullus, 2017). The latter implies having to throw the chewed gum into special containers, rather than into ordinary trash bins without any appeal for the consumer. However, none of these strategies has contributed significantly to solving the pollution problem.
After the chewing, the base gum remains as a non-degradable residue. This is the main ingredient that manufacturers keep as reserved information (which is commonly not shared or declared). The base gum is a supremely soft food-grade chewable plastic made of polymers, plasticizers, and resins, which is not biodegradable. In the United States, Butadiene-styrene rubber, Isobutylene-isoprene copolymer (butyl rubber), paraffins (obtained by Fischer-Tropsch process), natural or synthetic petroleum waxes, polyethylene, polyisobutylene, and polyvinyl acetate are some of ingredients approved for the making, formulation, and production of chewing and bubble gum (Fritz and Elias, 1980).
In this context, our work aims to study the feasibility of modifying the properties of chewing gum waste by means of a chemical reaction carried out under conditions similar to those of cross-linking rubber by vulcanization, in order to take advantage of some similarities between chewing gum and rubber regarding their structure and properties. It is expected that the cross-linking modifies the polymeric properties of chewing gum residues, as in the vulcanization of natural material (i.e., through the use of sulfur, one of the most efficient and widely employed industrial methods), generating desirable characteristics in the products at a low cost. Therefore, the polymeric structure of the chewing gum base could be modified by cross-linking in a rubber vulcanization-like process to produce a less polluting material, which might have suitable properties for some applications.
Since the discovery of vulcanization, several researchers have highlighted the effect of the filler, the plasticizer, and the anti-oxidant on the process. A free-radical mechanism for rubber cross-linking was originally suggested by Flory (1953), Shelton and McDonel (1960) and Kruzelak et al. (2017). Other evidence suggests an ionic mechanism that leads to the formation of macromolecules by sulfur bridges (C-Sx-C), which is initialized by sulfur (the cross-linking agent) with zinc salt (the accelerator) to produce a type of perthio-salt [activator-Sx-Zn-activator]. This induces the formation of a rubber-sulfur bond, removing hydrogen from the methylene groups located in alpha to an unsaturation in the structure of the natural rubber (Niyogi and Varma, 2007). However, as it probably involves a complex sequence of reactions, the mechanisms and the chemistry involved in the rubber cross-linking process using sulfur have not been fully understood and are still a subject of prime interest.
In rubber vulcanization, different types of accelerators are used to obtain better use properties and shorter process times. The cross-linking process catalyzed solely by the action of zinc salts (activators) can take up to 4 hours (Bevilacqua, 1959; Nieuwenhuizen, 2001; Sae-oui et al., 2007; Bornstein and Pazur, 2020). In the cross-linking with sulfur, the selected accelerator determines the type of network structure produced, as well as the specific properties of the material obtained (Markovic, 2009). The basic characteristics of the accelerators used in rubber vulcanization are usually similar and are composed of one or two sulfur atoms located between a pair of organic groups.
There are primary and secondary accelerators responsible for increasing the reaction speed in the vulcanization of rubbers, e.g., cyclohexyl benzothiazole sulfenamide (CBS) and tetramethylthiuram disulfide (TMTD). The former is a primary accelerator that, at normal vulcanization temperatures (408 - 413 K), increases the speed of the process; and the latter is a secondary accelerator that acts as a sulfur donor which favors the stabilization of the torque, generates a high cross-linking density, and provides vulcanized rubbers with a resistance to thermal degradation (Saeoui et al., 2007). Binary accelerator combinations have also been widely used, as they provide higher curing rates in rubber vulcanization (Kim et al., 2011 ; Wang et al., 2019).
This paper presents information on the laboratory-scale obtainment of a material from chewing gum waste subjected to a process similar to that of the sulfur vulcanization of natural rubber, and it provides some data on its preliminary characterization by ATR-FTIR, rheometry, Shore A hardness, and density measurements taken in order to determine the curing time, as well as to analyze, through the results of these experiments, the changes generated by the process in the chewing gum waste properties. It is considered that a treatment like this could help revalue this waste by finding an appropriate use for the produced material.
The evolution of the whole process was monitored via a series of mid-infrared ATR-FTIR (attenuated total reflectance with Fourier Transform) spectra of aliquots isolated from the reaction medium every 10 minutes for 4 hours, as well as through sequential measurements of torque during the process (from the raw material until the final product was obtained). The absorbance of the bands in all ATR-FTIR spectra were normalized with regard to the absorbance of the band at 2 953 cm-1, as it was practically constant in all spectra. The fundamental bands of the ATR-FTIR spectra were analyzed in terms of their maximum absorbance wave number and intensity changes, as manifested by the normalized absorbance during the process, using a descriptive statistical analysis in order to get information about changes caused by this reaction in the functional groups present in the structure of the material.
Methodology
All of the rubber vulcanization-like processes were carried out from raw material obtained via a collection campaign of chewing gum waste discarded from Colombian commercial brands, which was carried out at Universidad EAN (Bogota, Colombia) at the beginning of 2019. The chewing gum wastes were sanitized with 1% sodium hypochlorite and by autoclaving (at an internal pressure of 98 to 103 kPa and a temperature of 373 K to 393 K), and they were dried properly before subjecting them to the vulcanization-like process.
The drying of the gum was carried out by making thin sheets in a roller mill with a thickness not greater than 0,3 mm and subjecting them to air drying for 6 hours. The kneading and formation of a new sheet was repeated to regenerate the area exposed to drying in order to foster the loss of water trapped between the polymer chains. The drying cycles were repeated until no changes were observed in the O-H stretching band (v O-H) of the ATR-FTIR spectrum. The base formulation for the rubber vulcanization-like process used in this study is summarized in Table 1.
Table 1 Base formulation of chewing gum waste compound (weight per 100 parts of chewing gum). Natural rubber was used for control.
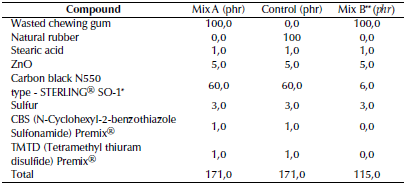
Source: Authors
* Rubber® furnace industrial-grade carbon black, from Cabot Corporation
** Mix B was the only one monitored via ATR-FTIR
Sample preparation for rheometry
Mix A and B were prepared using a 300 mm laboratory mixer roll mill at a roll speed ratio of approximately 1 100 rpm and a roll temperature of 293-303 K. The processing time after the addition of each component of Mix A was approximately 2 minutes. Rheometric characteristics were evaluated using a Prescott MDR MINI Oscillating Disk Rheometer, in accordance with the ASTM-D5289 (2019) standard test method. The hardness of the samples was measured using the PTC 306 L equipment according to ASTM-D792 (2020), while density was measured according to ASTM-D2240 (2021).
Mix B was made without the addition of accelerators (CBS and TMTD) and with a lower amount of sulfur in order to slow down the process and monitor the changes of the functionalgroups presentin the mixviaATR-FTIR, improving the quality of the baseline of the spectra. It was subjected to heating for 4 hours at a temperature of 413 K, and samples of the reaction medium were taken every 10 minutes for the subsequent acquisition of ATR-FTIR spectra.
ATR-FTIR analysis
Each sample (aliquot isolated from the reaction medium) was cooled in liquid nitrogen to stop the reaction before acquiring its ATR-FTIR spectrum at room temperature with a Shimadzu IRTracer-10 spectrophotometer equipped with a DLATGS detector (deuterated L-alanine doped triglycine sulfate), a mid-infrared (IR) source, and a single-reflection attenuated total reflectance (ATR) zinc selenide (ZnSe) crystal. Each spectrum was collected by placing a portion of the material on the round crystal window. The ATR-FTIR spectrum of each sample was recorded in 32 scans in the range of 340 cm-1 - 4 000 cm-1, with a spectral resolution of 2 cm-1. This, with regard to a previous background spectrum acquired with a constant distance from the sample to the optic unit (guaranteed by the holding arm of the sample spacer device) in order to minimize any errors caused by non-uniform contacts when acquiring the spectra. The ATR-FTIR spectrum of each sample was recorded via the LabSolutions IR Data Collection software and analyzed in triplicate.
The entire vulcanization-like process of chewing gum waste was carried out in duplicate, starting from a variety of different chewing gum wastes. Thus, for this study, a total of 150 ATR-FTIR spectra were acquired, i.e., three spectra for every aliquot isolated from the reaction medium throughout the process.
Data processing
The baseline of each spectrum was adjusted with variable slope and offset, and an advanced smoothing with three algorithms (moving average, Savitsky-Golay, and percentile filter) was carried out. The spectrum was filtered by removing the cosmic spikes that can be present in all photoelectric readout systems as random unidirectional sharp spikes that distort spectra and may affect the results of later statistical analyses (Barton and Hennelly, 2019). An average ATR-FTIR spectrum of the n-replicates acquired of each aliquot isolated from the reaction medium throughout the process was calculated. All processing (including the conversion of spectrum data to .csv files) was made using the Spectragryph software (https://www.effemm2.de/spectragryph/index.html21/04/2023). Statistical analyses were conducted in R version 4.0.3 with RStudio version 1.3.1093, Windows 10+ (64-bit).
Descriptive statistical analysis of the ATR-FTIR spectrum
All of the ATR-FTIR spectra were normalized for the analysis, with the aim to make them comparable. 25 average spectra, each with 3 734 point data (wave number, absorbance), were studied. 13 bands present in all of the ATR-FTIR spectra were selected for the analysis. These bands were characterized by the wave number corresponding to its maximum absorbance and assigned according to previously published tables (Anderson et al., 2004; Silverstein et al., 1991; Stuart, 2004). It was assumed that each point data of a band (well-defined wave number, absorbance) in any spectrum correlates with that of the band located at the same wave number in any other spectrum belonging to the series of ATR-FTIR spectra under analysis (Smith, 2011).
The absorbance of the band at 2 953 cm-1 served as a reference to normalize the data of each spectrum, as it showed the least variation in all of the spectra acquired during the study. This normalization allowed comparing the spectra of the series, attenuating the effects caused by the penetration of the IR beam in the sample while acquiring each spectrum, thus avoiding interpretation bias in the results. For each of the 13 selected bands, the mean of the maximum absorbance wave number and its corresponding absorbance were calculated, as well as the dispersion range and the standard deviation of the mean. The normalization process was based on Equation (1 ), where a¿ j represents the absorbance of the band appearing at the -th wave number in the i-th spectrum for i = 1, • • • , 25 and j = 1, • • • , 3 734; ā2 953cm-1 denotes the average absorbance of the band at
: 2 953 cm-1 in the total series of the spectra; a
i2953cm-1
corresponds to the absorbance of the band at
: 2 953 cm-1 for each i-th spectrum; and α*
i, j represents the normalized absorbance for the corresponding band at the j-th wave number. The input for the statistical analysis was a 25x3 734 matrix of normalized absorbance values.
The Pearson correlation coefficient was calculated according to Equation (2) in order to show the probable relationships between bands attributed to certain functional groups and particular bonds in the literature (Anderson et al., 2004; Silverstein, et al., 1991; Stuart, 2004).
where xi and yi are the values of absorbance for the bands x and y at the i-th spectrum; n is the number of spectra; x and y are the mean of absorbance value for each band; and sx and Sy are the corresponding standard deviations. This coefficient takes values between +1 and -1. Values close to +1 indicate a positive linear correlation (director increasing), and values close to 0 evidence no linear correlation. Those close to -1 are indicators of a negative linear correlation (inverse or decreasing). To evaluate whether the coefficient obtained was statistically significant, the hypothesis H0: p = 0 was evaluated with a significance of 5%. The cor.test function from the stats library of R was used for contrasting the hypothesis.
Results and analysis
Table 2 Test on unvulcanized and vulcanized products
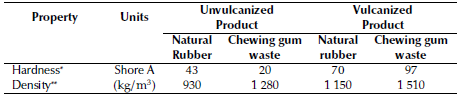
Source: Authors
* Measurement uncertainty ±0,03. ** Measurement uncertainty ±0,5kg/m3
At the industrial level, vulcanization has been extensively used for cross-linking natural rubber, and a vulcanizationlike process has not been applied to chewing gum waste, which is typically disposed of as useless polluting trash. This report shows the results of applying said process on chewing gum waste, which was carried out under conditions similar to those of industrial rubber vulcanization and monitored via rheometry measurements and ATR-FTIR spectrometry from the raw material until the final product was obtained, including the aliquots isolated from the reaction medium every 10 minutes during the whole process. This was done in our laboratory under the expectation of obtaining a cross-linked product with different physical-chemical properties, perhaps suitable for a more profitable use than that of the chewing gum waste.
The measured elastic torsional (S’) data in the rubber vulcanization processes provide indication of the cross-linking level achieved (i.e., the sum of the chemical bonds involved in the cross-links). Figure 1 shows the rheometric monitoring of the rubber vulcanization-like process of the chewing gum waste at 413 K in Mix A. The curve in Figure 1 has a similar behavior to that of reversion in rubber vulcanization (Bornstein and Pazur, 2020), where a steep linear drop of torque precedes the reaction stage during precursor formation, in a way analogue to the first stage occurring with uncured rubber, which will be discussed later in the ATR-FTIR analysis section. After said drop, the S' value shows a fast rise, which can be associated with a cross-linking density increase, just up to a maximum value of 17,46dNcm. Then, S' values show a slight drop, probably due to a reversion process, a term generally applied to the loss of network structures due to non-oxidative thermal aging, as a result of an overly long vulcanization (Coran, 1964; Bornstein and Pazur, 2020).
The Mix A formulation was characterized by the addition of 3,0phr of sulfur, a higher content of carbon black (60phr), and the use of CBS and TMTD as accelerators. Based on the monitoring of torque during the process (Figure 1), a scorch time TS2 (at which cross-links begin to occur) equal to 1, 96 min was determined, as well as the time at which the progress of the cross-linking in the vulcanization reached 90% of the maximum (T90), equal to 5,15 min (T90 = 3,74 minutes for natural rubber).
The final material obtained via the vulcanization-like process of chewing gum waste using CBS and TMTD in Mix A (Table 1) was characterized in terms of density and Shore A hardness (Table 2). The density (1 510 ± 5kg/m3) turned out to be approximately 1,18 times higher and the shore A hardness (97 ± 0,3) 77 units larger than those of the raw material (chewing gum waste). The Shore A hardness of the raw material (20 ± 0,3 units) classifies chewing gum waste as a soft material, while that of the material obtained with this treatment is characteristic of extra-hard materials.
All of these data evidence the feasibility of the rubber vulcanization-like process of chewing gum waste. Under conditions similar to those experienced by rubberin industrial vulcanization, this process is able to change the properties of chewing gum waste and transform it into a material with characteristics that could be adapted for convenient and cost-effective uses.
The Mix B formulation was characterized by lower contents of carbon black and sulfur. The vulcanization-like process of Mix B produced a material whose Shore A hardness (70 ± 0,3 units) classifies it as a medium-hardness material. The fact that this product has a lower hardness than the product obtained from Mix A can be attributed to the lower content of carbon black, which was used as a reinforcing material in Mix B (Sanchez et al., 2020), and, by analogy with rubber vulcanization, to the use of the CBS and TMTD accelerators, which have shown some influence on the final properties of vulcanized rubber (Sae-oui et al., 2007; Markovic et al., 2009; Formela et al., 2015). The increased concentration of carbon black in Mix A formulation had a positive effect on the hardness and density of the final product, possibly because, as in the case of rubber, it acts as a support material to form the bonds with the material that is being vulcanized (Bueche, 1961; Janacek, 1962).
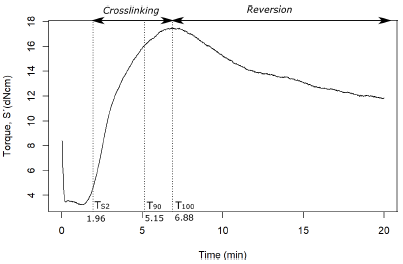
Source: Authors
Figure 1 Rheometer curve for the vulcanization of chewing gum waste in Mix A at 413 K
Monitoring the process via ATR-FTIR
Figure 2 presents the ATR-FTIR spectra series of the raw material, the reactives, the prevulcanized material, and the 25 aliquots isolated from the reaction medium every 10 minutes duringthe vulcanization-like process of Mix B (Table 1), which were acquired at 413 K.
Seeking to minimize any distortions due to overlapping signals in the components of the ATR-FTIR spectra of these mixtures, this study considered the intensity of the bands, which proved to be more accurate than its integral when analyzing the behavior of the set of signals in the spectra. Table 3 shows the location (wave numbers) and the average with the dispersion of the absorbance for each spectral band of interest in this analysis. The band at ῡ
: 2 953 cm-1 showed the least variation in the whole the set of spectra during the whole process; it had a dispersion of 0,04 units and a coefficient of variation of 13, 39% around its average value. Therefore, the band at
: 2 953 cm-1 was chosen as the reference to normalize the absorbance of all other bands of interest in each spectrum of the ATR-FTIR series of the samples (raw material, pre-vulcanized material, and aliquots). It is worth adding that the term to normalize means to represent each data from a sample of data (each ATR-FTIR spectrum of the series) by a ratio of proportion obtained as the quotient resulting from dividing it by another data belonging to the same sample, which is chosen as a common reference for all the data in the sample, in order to unify their scale and make them comparable to other equally normalized samples, i.e., with respect to the same reference (the average absorbance of the band at 2 953 cm-1).
In order to obtain information on changes in the chemical composition during the vulcanization-like process of the chewing gum waste, the corresponding 13 bands of each spectrum (Table 3) were selected along with their already normalized average absorbances, considering the magnitude of the coefficients of variation found for their average values of normalized absorbance in the whole set of spectra for the aliquots isolated from the reaction medium during the process. These bands were assigned as indicated in the literature (Anderson et al., 2004; Silverstein, 1991; Stuart, 2004), and their average absorbances were subjected to a statistical treatment, such as the one shown in Figure 3, looking for changes in the initial composition which could be interpreted as the occurrence of a vulcanization-like process of the chewing gum waste. Thus, changes in the carbonyl, methylene, and carboxylate groups were found and taken into consideration for the analysis.
It is very common to use the Pearson correlation coefficient to quantify a possible linear correlation between two sets of data (Zou et al., 2003). This coefficient was used to analyze linearity of the absorbances of all the spectral bands chosen for this study (Jampafuang et al., 2015; slouf et al., 2018).
For this analysis, the data from the ATR-FTIR spectra set were represented in a 2D matrix (1 line = absorbance of the first band; 1 column = absorbance of second band), and the correlation matrix was computed for the eight spectral bands with the highest coefficient of variation during the process: the bands at
: 1 700 cm-1 and
: 1 732 cm-1 for the stretching of carbonyls (v
C
=O); the band at v : 1412 cm-1 for the bending of methylene and/or the methyl groups (δH-C-H, δH-C-C, and δH-C-O); the bands at v: 1 559 cm-1 and
: 1 538 cm-1 for the bending of ester carboxylate groups
Table 3 Assignment of the bands and descriptive statistics of the average absorbance local maximum data prior to normalization for the bands of interest in the whole set of ATR-FTIR spectra of the aliquots isolated from the reaction medium during the vulcanization-like process of chewing gum waste
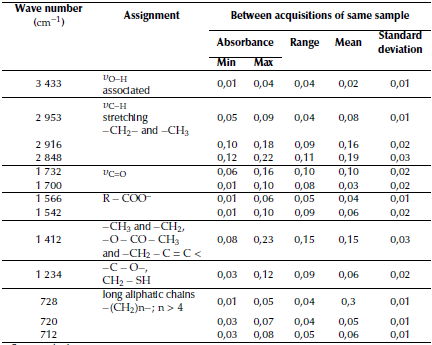
Source: Authors
(δo=C_O); the band at v: 1232 cm-1 for the stretching of C-O bonds in sp2 hybridized carbon (v=C-O) or the stretching of vC-S bonds (vC-S) in mercaptan-methylene fragments, -CH2-SH, which is most likely overlapped in this spectral region; and the bands at v: 728 cm-1 and v: 712 cm-1 for the rocking of CH2 in aliphatic chains, -(CH2)n- with n > 4.
Figure 3 depicts the 2D matrix considered for the statistical treatment when correlating the average maximum of absorbances for nine bands located at a given wave number in each ATR-FTIR spectrum of the series, which were finally chosen due to their proven relevance for interpreting the results. The form and the intensity of such spectral bands are shown by the curves appearing in the cells of the diagonal of the matrix in Figure 3. The bands at
: 1 732 cm-1,
: 1 700 cm-1 (vC=O), and the band at v: 1 234 cm-1 (v=C_O) frequently exhibited low absorbance values due to their asymmetric and variable forms (mostly with positive asymmetry). The other bands had a less pronounced yet similar tendency. For example, in Figure 3, an increasing linear relation is noticeable between the band at v: 1 732 cm-1 (vC=O) and the band at v: 1 234 cm-1 (v=C_O), supported by a linear correlation coefficient of 0,974, with a p-value of less than 5%. This means that the higher the absorbance values of the band at
: 1 732 cm-1 (vC=O), the greater the absorbance values for the band at
: 1 234 cm-1 (v=C_O).
Another possible increasing linear relation is that of the bands at v: 1 412 cm-1 (δH-C-H, δH-C-C, δH-C-O) and v: 712 cm-1 (δH-C-H), with a correlation coefficient of 0,684 and a lower p-value of 5%.
The absorbance values of the bands at v: 1 542 cm-1 and v: 1 700 cm-1 (vC=O) or those at v: 1 566 cm-1 (vO=C-O) and v: 1 700 cm-1 (vC=O) showed a linear decreasing relation supported by negative linear correlation coefficients of -0,665 and -0,631, respectively. Both had a lower p-value of 5%, indicating that these bands are attributable to independent phenomena occurring in functional groups without common atoms or bonds, as well as in different structural fragments of the sample.
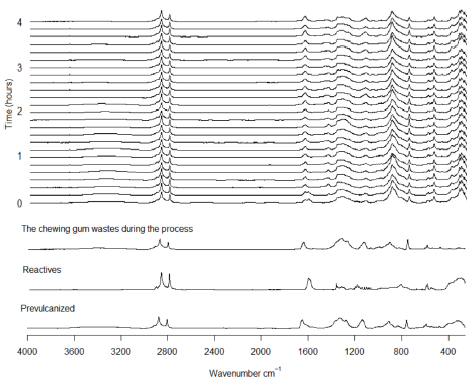
Source: Authors
Figure 2 ATR-FTIR spectra acquired during the vulcanization-like process of the discarded chewing gum normalized at peak intensity (2 953 cm-1) - Mix Bat 413 K
As mentioned above, these first results are only presented as a first approach, in order to collect evidence of the feasibility of the reaction of chewing gum waste under rubber vulcanization-like conditions, with the purpose of transforming these residues into materials with distinct properties, which could be suitable for profitable use. Therefore, these results do not pretend to clarify the mechanism involved in the chemical reactions occurring during the process; they aim to serve as a descriptive analysis of the changes observed, based on some similarities and differences with the processes recorded by the authors of previous studies on rubber vulcanization.
The most noticeable spectral features that were modified in the ATR-FTIR spectra of the materials isolated from the reaction medium during the treatment (Figure 2) can be thus described: the medium intensity of the band at v: 3 433 cm-1, attributable to the stretching vibration of associated O-H(vO_H), was present in the spectrum of the chewing gum waste and in those of the aliquots initially isolated from the reaction mixture, but this intensity decreased slightly in the spectra of aliquots, with some grade of cross-linking (vulcanized samples). Its appearance in the spectrum can be explained by residues of sweetener and acids as original components of the chewing gums, by the product of partial deacetylation of its major constituent (the polyvinyl acetate, PVAc) (Rimez et al., 2008), or by occluded water that was not removed by drying, as revealed by the band at : 1 640 cm-1, attributed to H-O-H bending (δH_O_H), in the spectrum of the chewing gum waste.
The bands at v: 2 953 cm-1,
: 2 916 cm-1, and
: 2 848 cm-1, due to C-H stretching vibrations on sp3 hybridized carbon atoms in aliphatic fragments (vC_H), correlate with the bands of bending in planes H_C_H of methylene groups (δH_C-H), or in planes H-C-C or H_C_O of methyl groups (δH_C_C, δH_C_O) that appear at
: 1 450 cm-1 and
: 1 433 cm-1, and their changes in the ATR-FTIR spectra have been previously associated with the progressive cross-linking occurring in rubber vulcanization with sulfur (Koenig, 1975), as a result of the decrease in a-methylene hydrogen contents caused by the vulcanization reaction, in which hydrogen, located in alpha, is abstracted to unsaturation as something concomitant to the formation of sulfur bridges (C_Sx_C). For the set of spectra acquired throughout this research, the variation coefficient of the average absorption in the band at
: 1412 cm-1 was 19,07%. At
: 1 475 - 1 365 cm-1, besides the bands of bending in planes of the _CH3 and -CH2- groups, those of bending in planes H-C-O of the -O-CO-CH3 fragment appear overlapped and interfere with the analysis of their behavior throughout the process.
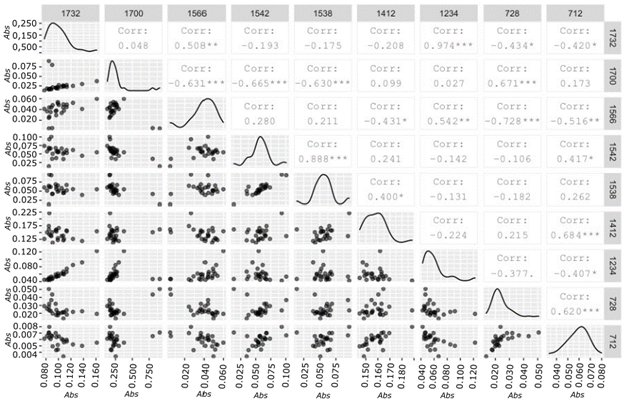
Statistical significance level: * 0,05, ** 0,01 and *** 0,001. The first line and the last column are wave numbers (cm-1).
Source: Authors
Figure 3 Linear correlation 2D matrix for the absorbance variation of the chosen bands of interest to analyze the set of ATR-FTIR standard reference spectra (normalized) of aliquots isolated from the reaction medium during the vulcanization-like process of chewing gum waste.
There are also three bands in the interval comprising v: 735 - 700 cm-1 which are attributable to the rocking or asymmetrical bending in planes H-C-H in aliphatic chains with four or more consecutive methylene groups. This set of bands, with maxima at v: 728 cm-1, at v: 720 cm-1, and at v: 712 cm-1, are complementary to the two sets of signals described before. Its appearance in the spectra can be associated with the gum base and the presence of stearic acid. This analysis shows the band at v: 712 cm-1 correlated to that at v: 1412 cm-1 (0,684 Pearson correlation coefficient, 5% significance) while the band at v: 728 cm-1 is correlated to that at v: 1 700 cm-1, which is attributable to stretching of carbonyl (with Pearson correlation coefficient of 0, 671 and a significance of 5%).
When it comes to studying rubber vulcanization and taking into account the IR spectra acquired before and after the process, different approaches have been proposed. Shipman and Golub (1962) reported that the most prominent change in the infrared spectrum of rubber after vulcanization with sulfur is the appearance of a new absorption at v: 961cm-1. This signal was associated with a carbon-carbon double bond formation (-CH=CH-) by elimination (Shipman and Golub, 1975), i.e., one from the various possible reactions considered in rubber cross-linking. Nevertheless, this signal did not appear in any spectrum of the ATR-FTIR monitoring of the vulcanizationlike process of chewnig gum waste under similar conditions, albeit without the use of accelerators.
Additionally, this set of ATR-FTIR spectra allowed detecting some modifications undergone by the zinc oxide and stearic acid present in the reaction medium since the beginning of the process as precursors of zinc-derived accelerators in the vulcanization (Ikeda et al., 2009).
A critical step to attaining efficient curing is the formation of zinc stearate via the reaction of stearic acid and zinc oxide (Figure 4). In the vulcanization-like process of chewing gum waste, this was monitored based on the band at v: 1 542 cm-1, attributable to the asymmetric stretching vibration of the carboxylate anion, which stands for the complete resonance in the C-O bonds of the carbonyl group due to coordination with the metal (Figure 4). During the first minutes of the process, this band showed an increase in absorbance (a sign of zinc stearate production), while the absorbance of the band at v: 1 700 cm-1, attributable to the stretching of carbonyl (vC=O) in the carboxyl group of the acid, had a proportional decrease, a sign of stearic acid consumption (Figure 3).
In other words, the carbonyl absorption decrease (at v: 1 700 cm-1) was replaced by the increase of that of the band at v: 1 542 cm-1 appearing in the spectrum (this was supported by a Pearson correlation coefficient of -0,665). The band at v: 1 542 cm-1 appeared as a unique band in the spectra of aliquots isolated until 90 minutes after heating began. From that time onward, a second band appeared at v: 1 566 cm-1, attributable to the anti-symmetric stretching of bands for the bidentate association with zinc ions, which has a Pearson correlation coefficient of -0,631 with the band at v: 1 700 cm-1. A similar behavior was reported for rubber vulcanization (Musto et al., 2013; Ikeda et al., 2015).
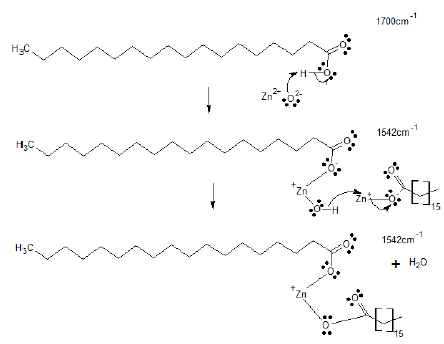
Source: Authors
Figure 4 Reaction scheme for the zinc stearate formation related to ATR-FTIR signals recorded during the vulcanization-like process of chewing gum waste
In the whole set of ATR-FTIR spectra for aliquots isolated from the reaction medium during the studied process, major variations were observed in the absorbance of the bands at v: 1 700 cm-1, at v: 1 566 cm-1, and at
: 1 542 cm-1 for the first monitoring intervals times (0-10 min); whereas the bands at
: 1 732 cm-1 and
: 1 234 cm-1 varied noticeably in the spectra of aliquots isolated at the end of the reaction (220-240 min). Both sets of bands are related to phenomena occurring in carboxylate or carboxyl functional groups, but the variations in the band at v: 1 732 cm-1 do not behave similarly to those of
: 1 700 cm-1. This suggests that the band at v: 1 732 cm-1 might be a result of the overlapping between bands from the -COOH group of stearic acid associated with hydrogen bridges (Musto et al., 2013) and those from carbonyl of the carboxylate groups of PVAc in the chewing gum waste, as evidenced by the appearance of said bands in the spectrum of the residues, as well as by their absence in the spectrum of the other reagents (Figure 2).
The absorbance of the band at v: 1 732 cm-1 (u=C_O) varied just as that at
: 1 234 cm-1 (u=C_O); it showed a significant drop in the spectra of aliquots isolated from the reaction medium during the monitoring time, as well as a Pearson correlation coefficient of 0, 974 with a 5% significance, suggesting a progressive PVAc degradation under the oxidative conditions inherent to the process applied to chewing gum waste, which was statistically supported by a Pearson correlation coefficient of 0,974 and a 5% significance. This indicates the feasibility of the reaction of such residues under rubber vulcanization-like conditions.
Conclusions
These findings are the first to show that, under conditions such as thoseof rubber vulcanization, chewing gum residues react to produce materials with different properties, thus evidencing the cross-linking of the lineal chains initially existing in their components, as it probably happens in the aforementioned process.
Monitoring via rheometric measurements and ATR-FTIR spectrometry of a vucanization-like process involving chewing gum waste on a laboratory scale allowed determining the occurrence of cross-linking in a reaction time of 5,15 minutes. A harder (Shore A hardness: 95 units) and denser (1510 kg/m3) material than chewing gum wastes was obtained (Shore A hardness: 20 units and density: 1280 kg/m3).
Monitoring via the ATR-FTIR spectra of the vulcanization-like process of chewing gum waste evidenced the occurrence of structural changes throughout the process, which was also proven by the distinct values of their corresponding rheometric properties, always indicating a progressive evolution in the reaction, similar to that of cross-linking in rubber vulcanization.
These initial findings suggest the possibility of reincorporating chewing gum waste into market value chains and promoting the search for profitable alternatives to treat this type of environmental contaminant. This work encourages readers to consider further research, the scaling of the process, and the characterization of the final product with more rheometric measurements such as the storage and loss modulus, together with viscosity parameters that could enrich the prospects of this research.