1. Introduction
Chitosan (poli-β-(1,4)-N-acetil-D-glucosamida) is a natural biopolymer which has received great attention due to its wide range of applications such as removal of metal ions in contaminated water, dyes removal from water [1-8] and its versatility to form different types of materials such as chitosan conjugated DNA nanoparticles, graft copolymerized chitosan, biomaterials and composite scaffolds among others [9-16]. However, the insolubility of this polysaccharide in regular organic solvents and water has encouraged researchers to change its structure to improve its potential use in different fields of interest [17-19]. One of the modifications carried out consists in the reaction between the amino groups of chitosan and aldehyde derivatives to obtain imines by condensation reactions. Such as reactions are interesting not only because they improve the solubility of chitosan by disrupting the intermolecular hydrogen bonds [19, 20], but also because a versatile group such as an imine provides this polymer with new potential applications [21]. Even though obtaining imine-chitosan derivatives could be challenging due to the acidic aqueous media necessary to dissolve the polymer (which also should not allow the equilibrium to be displaced towards the imine products), several simple and mild aqueous methodologies have been reported allowing this type of reactions to be classified into the green chemistry field [22-24].
Chitosan, in different presentations, has already been tested in metal sorption studies with the purpose of being subsequently used in wastewater treatment with remarkable results [25-29]. Many of these studies have reported that the main center of metal ion interaction are the -NH2 and -OH terminals of chitosan [30-32]; however, different functionalized chitosan species have shown to be very convenient since they stabilize the polymer in acid media making it insoluble and therefore allowing post sorption separation to be easier as they add new groups that can interact with different metals of interest [33-36]. It is worth mentioning that some of these modifications reduce the sorption efficiency because of the loss of -NH2 or -OH groups [37.38].
On the other hand, compounds such as hydrazones are of interest in supramolecular chemistry since appropriately substituted hydrazones are able of acting as ligands in coordination chemistry [39, 40], finding use as molecular switches [41-46], metallo-assemblies [47-49], among others [50, 51].
Herein we report a water-based synthesis of three novel imine-chitosan derivatives. Such compounds exhibited solubility in different range of pH depending on the type of functionalization. Our results represent a new class of chitosan derivatives that can be used for different applications, for instance, coordination with metal ions for wastewater treatment among others.
2. Experimental
2.1 Materials and equipment
Most reagents were purchased from Sigma Aldrich and used without any further treatment. Glacial acetic acid, methanol and chloroform were purchased from Baker and used without further purification. Mycelia derived from citric acid production were provided by SUCROAL S.A. (Cali, Colombia).
FT-IR spectra were recorded in a Thermo Nicolet 6700 spectrophotometer equipped with an ATR-FTIR accessory. 1H-NMR spectra (in deuterated chloroform and dimethyl sulfoxide) were carried out on a Bruker UltraShield 400 MHz spectrometer. Turbidimetry studies were performed on a UV-vis UV-1700 PharmaSpec spectrophotometer while elemental analysis for C, H and N were carried out with a Thermo FLASHEA 1112 series CHN analyzer. The TGA and DSC studies were performed on a simultaneous Mettler Toledo thermal analyzer, using 5-10 mg of sample of both pristine and functionalized chitosan, varying the temperature from 25°C to 600 °C at a 10ºC/min rate.
2.2 Extraction of chitosan
The extraction process was based on a previous study in our research group using the same source of chitosan [52]. Aspergillus niger mycelium (100 g) was washed three times with distilled water and dried at 60 ºC during 12 hours. Then it was subjected to basic hydrolysis during 4 hours with a NaOH solution (40% w/v) at 100 °C. The obtained beige solid was washed with distilled water until obtaining pH of 7-8. Then, three liters of distilled water were poured over the solid and glacial acetic acid was slowly added while stirring to reach a pH of 3.8. The obtained suspension was then passed through white cambric fabric filter and the filtrate was driven to a 9-10 pH value with addition of a 30% NaOH solution. The beige precipitate was purified twice by dissolution in acetic acid media (pH 3.8) and precipitating with NaOH. Yield: 5.5 %. Elemental analysis: C: 33.52%; N: 6.25%; H: 6.49%.
2.3 Characterization of chitosan
Measure of molecular weight
The molecular weight of chitosan was calculated by gel permeation chromatography (GPC) and intrinsic viscosity measurements. HPLC chromatography and Shodex SB-805 HQ, Shodex SB-806 HQ columns were used for GPC. The calibration curve was prepared with standard pullulan solutions with molecular weight between 1300-22000 g/mol in 0.15 M NaNO3 and 0.5 M HCOOH with a flow of 1 mL/min. Intrinsic viscosity measurements were performed in a Ubbelohde No OC-200 viscometer using the Huggins and Mark-Houwink-Sakurada equations. The viscometer constant, 3.14x10-7cm4/s2, was determined with a 0.2 M NaCl/0.1 M HCl solution.
Characterization of chitosan: Degree of deacetylation (DD) by potentiometric titration
Chitosan (100 mg) was dissolved in a 0.3M HCl solution and titrated with standardized NaOH solution. pH measurements were recorded using a Schottgerate CG832 pH-meter. A curve with two inflexion points was obtained by using the first derivative criteria over the original curve. The amount of acid consumed between these points is the one necessary to neutralize the amino groups in the polymer [53, 54].
1 H-NMR characterization
1H-NMR spectra of chitosan and its derivatives were obtained, using DMSO-d 6 /CF3COOD and D2O/CF3COOD (3:1). The NMR experiments were conducted at 45 °C. NMR signals were assigned according with reported data [55-57].The degree of deacetilation (DD) was calculated using 1H-NMR by following the indications on reference [44]. Likewise, the DD was determined by CHN elemental analysis as described by reference [58].
2.4 Synthesis of benzaldehyde derivatives
4-(bromomethyl)-2-hydroxybenzaldehyde, A 1
The salicylaldehyde derivative was synthesized by modification of a reported methodology [59]. Salicylaldehyde (610 mg, 5 mmol) was added stepwise during 30 minutes to a paraformaldehyde solution (500 mg) in concentrated HBr (2 mL). The solution was stirred at room temperature overnight. The obtained precipitate was washed with cold water (5 x 3 mL), filtered and dried to generate a white solid. Yield: 85%. 1H-NMR (400 MHz, CDCl3) δ (ppm): 11.10 (s, 1H, OH), 9.92 (s, 1H, CHO), 7.63 - 7.58 (mult. 2H, H-3 and H-4), 7.02 (d, 1H, H-6), 4.53 (s, 2H, CH2). 13C-NMR (100 MHz, CDCl3) δ (ppm): 196.09, 161.61, 137.74, 133.94, 129.53, 120.44, 118.46, and 32.35.
4-formyl-3-hydroxybenzoic acid, A 2
4-formyl-3-hydroxybenzoic acid was synthesized according to a reported methodology [60]. NaOH (20 g, 0.5 mol) were dissolved in 40 mL of water; 4-hydroxybenzoic acid (10 g, 72.4 mmol) in 10 mL of MeOH was added. Then, 20 mL of chloroform were added dropwise at 60 °C; this temperature was maintained during 10 hours with stirring. The resulting solution was cooled with ice and filtered to obtain a yellow solid. Then, the solid was dissolved in a concentrated ammonium hydroxide solution and precipitated with a 15% CuSO4 solution, yielding a green solid. This solid was dissolved in hot sulfuric acid and then cooled to obtain a yellow solid. Yield: 60%. 1H-NMR (400 MHz, DMSO-d 6) δ (ppm): 12.8 (s, 1H, COOH), 11.52 (s, 1H, OH), 10.31 (s, 1H, CHO), 8.24 (d, 1H, J = 2.3, H-6), 8.04 (dd, 1H, J = 8.7, H-2), 7.08 (d, 1H, J = 8.8, H-5). 13C-NMR (100 MHz, DMSO-d 6) δ (ppm): 191.08, 166.87, 164.52, 137.28, 131.21, 122.52, 122.42, and 117.99.
(E)-6-((2-(pyridin-2-yl)hydrazono)methyl)picolinaldehyde, A 3
This compound was prepared following a previous methodology reported by our group [49]. 2,6-pyridinedimethanol (200 mg, 1.43 mmol) was dissolved at 40 °C in CHCl3 and the minimum possible amount of MeOH. Then, activated MnO2 (625 mg, 7.19 mmol) was added to the reaction, which was followed by TLC and stopped when dialdehyde started to appear on top of it. The monoaldehyde obtained was purified by column with a 95:5 CHCl3/MeOH solution getting a 42% yield. The monoaldehyde was condensed with 2-hydrazinopyridine which was added in a 1:1 ratio in CHCl3 until a pale yellow precipitate was formed; this precipitate was washed with cold ethyl ether until no 2-hydrazinopyridine was observed in TLC revealed with iodide powder, giving the solid in 85% yield. This hydrazone was dissolved in CHCl3 to be finally oxidized with 4 equivalents of activated MnO2, purified by column using CHCl3/MeOH (9:1) as eluent, and obtained a yellow solid. Yield 51%. 1H-NMR (400 MHz, DMSO-d 6) δ (ppm): 11.36 (s, 1H, N-H), 9.97 (s, 1H, CHO), 8.24 (d, J = 8.0 Hz, 1H, H-5’), 8.16-8.14 (m, 2H, Himin and H-6), 8.03 (t, J = 7.8 Hz, 1H, H-4’), 7.82 (d, J = 7.5 Hz, 1H, H-3’), 7.69 (d, J = 8.7, 1H, H-4), 7.34 (d, J = 8.4 Hz, 1H, H-3), 6.86 - 6.81 (m, 1H, H-5). 13C-NMR (100 MHz, DMSO-d 6 ) δ ppm: 193.9, 156.9, 155.6, 152.5, 148.3, 138.6, 138.4, 138.3, 123.7, 121.3, 116.1, 107.2.
2.5 Synthesis of imine-chitosan derivatives, QA 1 -QA 3
The imine-chitosan derivatives were prepared by the solid-state methodology previously reported [61-63]. Chitosan (200 mg) was added to a 1 mL solution of 2.0% of acetic acid in methanol, then it was added dropwise an aqueous saturated solution of A 1 -A 3 . Then, the resulting mixture was heated at 70 °C. Reactions were monitored via ATR-FTIR by periodically evaporating the water of aliquots. After reaching equilibrium, the reaction mixture was cooled and the solvent was evaporated slowly. The resulting solids were washed with water, methanol, chloroform, hexanes, and ethyl ether until TLC did not show any non-reacting aldehyde derivatives. Finally, the solids were vacuum dried for 24 h.
2.6 Estimation of solubility at different pH values
Water solubility pH-dependence of extracted chitosan and imine-chitosan derivatives was estimated by turbidimetry following an already established methodology [56]. Therefore, solutions of the polymers were prepared in 1.0% acetic acid and a concentrated NaOH solution was added stepwise while measuring the transmittance at each previously determined pH. The λ used was 750 nm.
2.7 Heavy metals removing study. Pb(II) and Hg(II)
Standard solutions of Pb (100ppm) and Hg (1000 ppb) were prepared. These were diluted in order to obtain a calibration curve for Pb(II) (from 2-10 ppm) and for Hg(II) (from 6-30 ppb). Samples doped with Pb (10 ppm) were treated with 2-4 mg of the absorber (chitosan or imine-chitosane derivative) in aqueous media adjusting the pH to 4.0 with diluted acetic acid and other sample with diluted NaOH to adjust the pH to 10. The solutions were stirred during 4 hours, then filtered and the filtrate diluted to 25 mL. The same procedure was used to the samples doped with Hg. Pb and Hg were measured by atomic absorption spectroscopy with the flame technique for Pb and by hydrides generation for Hg. The amount of Pb(II) and Hg(II) absorbed in mg per mg of polymer (Qt) was determined by Equation 1 [64]:
C0 and Ct are the initial and final concentration (mg/L), respectively. W is the amount of imine-chitosan derivative, and V is the volume of the solution.
3. Results and discussion
3.1 Extraction and characterization of chitosan
The extracted chitosan showed a viscosity average molecular weight of 6.49x106 g/mol. This high molecular weight should make the polymer very insoluble, but the degree of deacetylation was high enough to help chitosan protonation in many sites thus improving its solubility in acidic media. The degree of deacetilation (DD) was determined by potentiometric titration and 1H NMR (see Table 1] getting similar results. The DD by 1H NMR was determined by the H1 (GluNAc)-H1 (GluNH2) integration set as previously proposed [56, 57]. In which, a DD of 84.74% was obtained (see supplementary information). Assignment of the signals was performed using previous reports (See Figure 1] [57, 65]. An acceptable agreement between data obtained by potentiometry and NMR was observed, selecting the DD determined by 1H-NMR as the most reliable [54]. In addition, the DD can be also calculated by CHN elemental analysis, based on the methodology reported in reference [58] we obtained a DD of 87.27 % which is in good agreement with the potentiometric titration and NMR determinations.
3.2 Synthesis and characterization of chitosan-imine derivatives
After characterization of chitosan, the imine-chitosan derivatives were synthesized in a green chemistry fashion by the reaction between extracted chitosan and aldehyde derivatives previously synthesized (See Figure 2 and supporting information). With this purpose, the imine derivatives were prepared without the use of organic solvents, just needing a very small amount of methanol. Noteworthy, aldehyde derivatives A 1 and A 2 possess a hydroxyl group in the orto position (with respect to the aldehyde moiety). This -OH group can allow the formation of an intramolecular hydrogen bond upon imine formation and therefore stabilizing the product.
Evidence for imine-bond formation (-C=N) is provided by the appearance of the imine vibration band between 1630 and 1640 cm-1 in the IR spectra for the chitosan derivatives, as well as by the decrease in intensity of the band around 1560 cm-1 which corresponds to the -NH2 group. There is also the disappearance of the aldehyde band around 1650 cm-1 which supports the notion of imine-bond formation [63] (See Figure 3]; however, it is necessary to be cautious with the assignment of the imine band since the amide band in chitosan is very close to this region and can be overlapped, making the assignment more difficult [61-66]. The 1H-NMR experiments showed new signals in the aromatic region attributed to the molecules attached to chitosan (see supplementary information).
Thermogravimetric analysis showed that imine-chitosan derivatives are decomposed in two main steps (See Figure 4 and Table 2]. The first step starts at 45 and 50 °C, usually attributed to the loss of residual or physically adsorbed water in the polymers [67]. Extracted chitosan showed two events at 80.6 °C and 281.6 °C due to the loss of water and several processes such as dehydration of the glycosidic rings, depolymerization and decomposition of the deacetylated and acetylated units, respectively [18, 68, 69]. Whereas the loss of water for the imine-derivatives occurs between 75°C and 90 °C, the temperature were extracted chitosan loses the most of its mass is higher than that for the derivatives QA 1 and QA 2 , but lower than the one for QA 3 . In the case of QA 1 and QA 2, both aldehyde derivatives have very polar groups, including carboxyl, amine, and alcohol terminals able to form hydrogen bonds and electrostatic interactions in the structure which can lead to more effective cross-linking, increasing the stability of the polymer. This effect has been previously achieved with other types of chitosan derivatives [70]. On the other hand, the lower stability for QA 3 may be due to the inserted substituents that should disorganize the crystalline structure of chitosan, achieved by hydrogen bonds formed between chains of polymer [71-74]. Additionally, the hydrazonyl group would not be able to generate enough electrostatic forces to stabilize the obtained polymer.
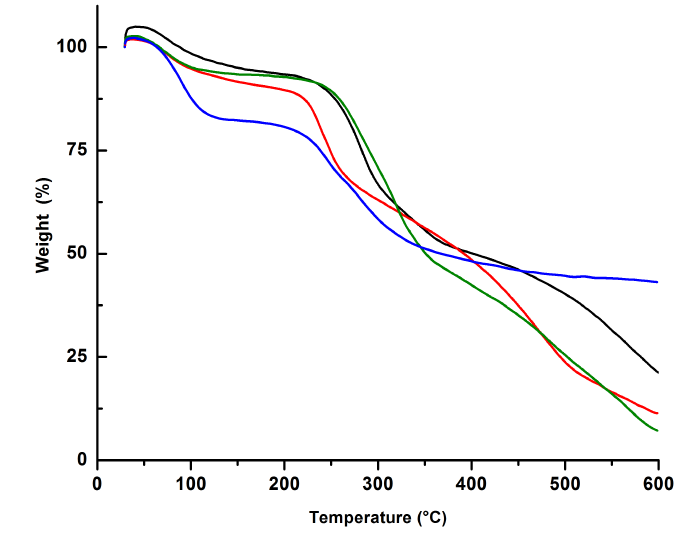
Figure 4 TGA curves of chitosan (black), QA 1 (red), QA 2 (blue), and QA 3 (green). and their corresponding first derivatives (dotted lines)
In the DSC curves, we found important differences when comparing endothermic peaks of chitosan and imine-derivatives (See Figure 5]. Previously it has been established that chitosan has two possible polar groups that can associate with water molecules: hydroxyl and amine groups all along the polysaccharide [75]. Since hydrogen bonds, formed with amine groups are weaker than those from with hydroxyl groups, it has also been established that desorption at temperatures lower than 100 °C corresponds to water interacting with -NH2 groups, while the imine-derivatives showed desorption at 118 - 124 °C assigned to water bonded to -OH groups [76]. Since the formation of chitosan derivatives occurs between the carbonyl and the -NH2 groups, there will be less free amino groups able to form hydrogen bonds with water. Decomposition signals after 250 °C agree with observations in the TG analysis, since all the chitosan derivatives showed a lower temperature of decomposition than that for the extracted chitosan due to the functionalization of chitosan with molecules possessing polar groups simultaneously increasing intermolecular forces and decreasing chain stiffness. Thus, the decomposition temperature is lower for the imine-chitosan derivatives than that of chitosan [77]. Moreover, the glass transition temperature (Tg) for all the imine-chitosan derivatives compared with the pristine chitosan suggested an increase in the flexibility due to the addition of those aldehyde derivatives to the chain of the polymer.
3.3 pH dependence studies of chitosan-imine derivatives
A pH-dependence study on water solubility was carried out since the presence of new functional groups should restrict or enhance solubility at different pH values. Figure 6 shows the solubility change tendencies when going from pristine chitosan to imine-derivatives. QA 1 dissolves well in most of the pH range, explained by the presence of both, non-functionalized -NH and hydroxyphenyl groups. For QA 2 and QA 3 , both of which have strong acidic groups, an increase in the solubility on basic media is evident. Since one of the interests of functionalizing chitosan for wastewater applications is allowing the polymer to stabilize (stay insoluble) in a wider range of pH values [28, 76, 78, 79], the result obtained for QA 3 is quite interesting, since only at pH values higher than 9 it starts to significantly dissolve. Noteworthy, functionalization of chitosan modulates its solubility in water; therefore, QA2 and QA3 are potential candidates for other applications in pH´s where chitosan lacks of solubility. For instance, if dealing with water waste treatment these derivatives can be used at different pH´s depending on the type of waste.
3.4 Metal Removal studies of chitosan-imine derivatives
The possible use of imine-chitosan derivatives for metal removal from contaminated water was evaluated by atomic absorption spectroscopy (see experimental section). As it is shown in Table 3, the solution pH controls the ability of metal removal. To pH 10 the imine-chitosan derivatives QA 1 and QA 2 are soluble and interact with the Pb(II) ions since the carboxyl and hydroxyl groups are deprotonated, however when the pH is acid the ability to absorb decreases due to the competition for the active sites between hydrogen ions in the media and Pb(II) ions [80]. On the other hand, the presence of hydrazonyl groups on imine-chitosan derivatives QA 3 allows coordination of metal ions showing interesting results. In the case of Hg(II) the results established the sensibility of the method is not high enough to determine concentration ca 10 ppb (See Table 2].
As it was mentioned before, pristine chitosan exhibits a larger Pb(II) removal in acid media while imine-chitosan derivatives a superior in basic pH´s. These results are of great importance since leads to the potential use of chitosan for treatment of contaminated water and therefore it deserves a further analysis as it is going on in our research group.
4. Conclusions
Functionalization of chitosan was achieved by the formation of Schiff bases between the free amine groups of the chitosan and three selected aldehydes. These compounds were characterized by 1H-NMR, elemental analysis, potentiometric methods, FT-IR, TGA, and DSC. Furthermore, the poor solubility of chitosan in basic media was overcame by functionalization, finding higher solubility for the compound bearing a carboxylic group. Likewise, the new compounds exhibit larger capacities for the removal of Pb (II) ions in solution at basic pH´s.