1. Introducción
The main purpose of this work was to identify fabrics that were produced locally and with the potential to be used as a reinforcement in polymeric matrix composites. The use of bio-fibers is considered by some authors as the next generation of structural materials [1, 2] and its use for several applications is a global trend in growth worldwide by aspects as the reduction of environmental impact due to biodegradability of vegetal tissues and their lower production costs, high availability and reduction of dangers associated to occupational hazards due to its production (as the inhalation of fiber glass particles), development of sustainable policies by the governments, generation of new incomes for the producing regions, among others. Flax, hemp, jute, sisal and bamboo fibers are commonly used for the manufacturing of polymer composites due to their low density and high specific properties [3, 4].
Vegetal fibers have a complex structure which consists of cellulose, hemicellulose, pectins, lignin and some other components [5, 6]. Properties of the composite depend on the adhesion force between fiber and matrix, on the woven fabric type and on any previous tension of the fibers. Some aspects that must be considered when working with vegetal fibers are their hydrophilic character (humidity absorption), the variation of their physical and mechanical properties due to growth conditions of the plant (as climate and location), the fiber processing technique and the variations on their transverse cross section and length.
The alkali treatment of vegetal fibers is a possibility to improve mechanical properties of composites by increasing the adhesion force between the fibers and the matrix through modification of the fiber surface with Sodium Hydroxide. NaOH is widely used for this purpose due to its ease of handling and low cost [7]. Some papers describe chemical treatments for flax, bamboo, pineapple leaf, kenaf, sisal, jute, hemp, agave, coconut and alpha fibers with 5, 6 and 7 % wt. of Sodium hydroxide NaOH [7-15]. Other works have studied a larger group of NaOH concentrations, such as 4-10% [16], 5, 10, 15% [17], 2-10% [18] and 0.8-30% [19]. The last work treated hemp, jute, sisal and kapok fibers and concluded that 6% NaOH is the optimum concentration in terms of cleaning the surface of the fibers while keeping a high crystallinity index. Some effects of alkali treatment on vegetal fibers include the removal of impurities and natural waxes and oils, the production of a rough surface topography, fiber fibrillation (axial split of filaments or microfibrils), increase of aspect ratio and effective area for wetting, increase of fiber density by the collapse of cellular structure, increase of free hydroxyl groups on the surface (increase in adhesion with polyester and vinylester resins), increase in crystallinity and the removal of lignin and hemicellulose, which are elements that play a cementation role in the fibers by transferring the stresses to the microfibrils [13].
The use of commercial vegetal woven fabrics allows the control of some variables, such as the orientation of the threads in the fabric and its dimensions and properties, the presence of dyeing in the fabrics, the thread count, the cost, among others. Commercial vegetal fabrics allow the reproducibility of the composite materials and a high productivity of the processes in aeronautic, marine and automotive applications [20].
Mechanical characterization of the studied vegetal fiber reinforced composites at the percentage of reinforcement allowed by the hand lay-up manufacturing technique resulted in higher specific properties for the untreated flax fabric composite.
2. Experimentation
2.1 Vegetal fabrics
The flax, cotton and jute fiber woven fabrics were found through suppliers located inside the Metropolitan Area of Valle de Aburrá (Antioquia, Colombia). The appearance, surface density, and the thread count in the warp (ends per centimeter - e.p.c) and weft (picks per centimeter - p.p.c) directions are shown in Table 1.
2.2 Chemical treatments
Samples of the three vegetal fabrics were chemically treated to study the effect of surface modification of the fibers on the mechanical behavior of the composites. Rectangular cuts of the fabrics were soaked in a Sodium hydroxide NaOH solution at 6% w/v (pH close to 14) for 48 hours [8,19]. After that, fabrics were submerged in Acetic acid at 1% v/v for 1 hour [21] and washed with deionized water until pH was as neutral as possible [22]. Drying of the fabrics was done in an oven at 60°C for 24 hours. The fabrics, cooled to room temperature inside the oven, were then stored in sealed zipper bags (Ziploc™) [23] at an average temperature of 14.2°C.
2.3 Fabrication of test specimens
Specimens for mechanical tests were manufactured using the hand lay-up molding technique. Each sample was molded individually to avoid stress concentration over the cutting edges of the test specimens. Matrix material is an Altek H834-R pre-accelerated and low styrene content polyester resin. It was catalyzed using Akpa A50-PF Methyl Ethyl Ketone Peroxide (MEKP) at 1.5% v/v. Additionally, BYK A-555 antifoam agent at 0.1% v/v was added to avoid the occurrence of air bubbles. Polyester resin was chosen as the matrix material for all the composites due to its extensive use in the local industry for the manufacturing of all kinds of products made of fiber reinforced composite materials, such as bathtubs, waterslides and swimming pools, bodyworks for cars and trucks, water storage tanks, tubes, profiles and more. The test specimens were kept at room temperature for 1 hour inside the molds. Then the samples were extracted and let to cure for other 7 days at room temperature. The cure schedule was performed at room temperature in order to achieve composite materials with mechanical properties that were as close as the properties developed by the composite materials manufactured by the local workshops and businesses that use hand lay-up technique to make parts made of fiber reinforced composite materials, most of which do not make use of curing ovens. Every group of layers used for the manufacturing of each test specimen was weighed prior to impregnation. Then, the test specimen was cured and weighed and the fiber weight to composite weight ratio (i.e. the fiber weight fraction) was calculated.
The specimens for the out-of-plane shear tests were manufactured by placing fabric strips one by one perpendicular to the horizontal plane (i.e. the 1-2 local plane. See Figure 9 of Ref. [24]) in a mold with the shape recommended by the standard. The resin was applied on one side of the mold after all the layers were placed. Then the mold was turned around and the resin was applied to the other side. The percentage of reinforcement for the specimens were determined as the highest fractions that could be achieved by using the hand lay-up molding process to assure a homogeneous resin impregnation of the reinforcement fabrics. A summary of specimen dimensions and testing parameters for each type of test is shown in Table 2. At least three specimens were manufactured for every system tested.
2.4 Tensile test
All tests were performed in an Instron 3345 testing machine and using an Instron 2519-107 force transducer with a 5 kN maximum load capacity. The fixing of the specimen to the grips was done directly over the surface of the specimen. The key factor in the selection of the gripping method is the prevention of premature failure as a result of a significant stress discontinuity. Test results presented in section 3.1 belong only to those tests were successful failure modes and failure locations were achieved (i.e. a lateral failure type, a gauge failure area and a middle failure position), so that a uniform stress field was present at the failure location. See section 8.2.1.2, 8.2.2.2 and Figure 4 of reference [25]. For measuring longitudinal strains, the extensometer that belonged to the machine was used, and for the transverse strains an Instron I3575-250M-ST extensometer was used. The yield point was calculated according to ASTM D3039-14 [25] for those specimens with bilinear stress-strain curves. Poisson coefficients were determined according to section 9 of ASTM E132-04 standard. The strain values that were used in the calculation of elastic modulus and Poisson ratios were determined as close as those suggested by ASTM D-3039 standard (0.001 and 0.003).
2.5 Compression test
Longitudinal strains were calculated based on displacements of the upper fixture relative to the lower fixture according to ASTM D3410-03 standard [26]. Transverse strains were measured using the Instron I3575-250M-ST extensometer by using a couple of extensions fabricated in aluminum to be placed on the opposite borders of the specimen [Figure 1]. Specific modulus and specific ultimate strengths for the tensile and compressive tests were calculated as Es= E/(A and S S =S U /(A , where E is the Young’s modulus in tension or compression, Su is the ultimate strength in tension or compression, and (A the density of the fabric in weight per unit of area [27, 28].
2.6 In-plane shear test
This uniaxial test was performed using the same fixture of the tensile test as recommended by the ASTM-D 3518-01 standard [29]. The layers for the specimens were all placed to get an orientation angle for the threads of ±45°. The formulas for the calculation of strains γ 12i , stresses τ 12i and modulus G12 can be found in the standard. If ultimate failure does not occur within 5% shear strain, the data shall be truncated to the 5% shear strain mark, which will be considered the maximum shear strain.
2.7 Out-of-plane shear test
This test measures the properties related to interlaminar shear strain and stress components. The test fixture is shown in Figure 2a. The test specimen has a rectangular shape with two opposite notches with an angle of 90°, which penetrate 12.7 mm towards the specimen center to get a uniform distribution of the shear strains in the central zone (see Table 2]. The formulas for the calculation of shear strains, stresses and modulus can be found in ASTM-D 7078-05 standard [24]. Strain measures were performed using Tokyo Sokki Kenkyujo TML BFLA 5-5-3LT and FLA 3-11-3LT strain gauges by placing them on one side of the specimen [Figure 2b).
2.8 Scanning electron microscopy
Top views of untreated and alkali treated fibers and transverse cross section surfaces of the composites were investigated by scanning electron microscopy (SEM), using a JEOL JSM-6490LV instrument. Samples were first covered by a fine layer of gold using a Denton Vacuum Desk IV vacuum deposition system.
3. Results and discussion
The nomenclature to differentiate a specific composite from another one in the Figures and Tables is as follows: the first letter indicates the type of treatment, where T is used for NaOH treated fibers and U for fibers that were used as delivered by the supplier. The second letter indicates the type of material, where F, C and J stand for Flax, Cotton and Jute, respectively. The following number is a consecutive, while the last number, which is separated from the letters by a hyphen, belongs to the percentage of fiber reinforcement by weight in the composite.
3.1 Tensile tests
Stress strain curves for treated and untreated flax fabric composites are shown in Figures 3a and 3b. The percentage of reinforcement varied from 17 to 19%. The ultimate strength of the composites reinforced with treated and untreated flax are very close to each other. However, the best results for the elastic modulus, yield stress and ultimate strength were those of the untreated flax composite. The flax reinforced composite admits high fracture tensile strains in the weft direction compared to the warp direction, which could have a pre-tension. This could explain its higher yield point and lower fracture strain compared to the same properties measured when the applied force is parallel to the weft direction of the composite. Also, the area under the stress-strain curve is bigger for the test in the weft direction than for the test in the warp direction which suggests a possible higher impact strength when forces are applied parallel to the weft direction, which means a better ability to absorb energy and plastically deform without fracturing [Figure 3b).
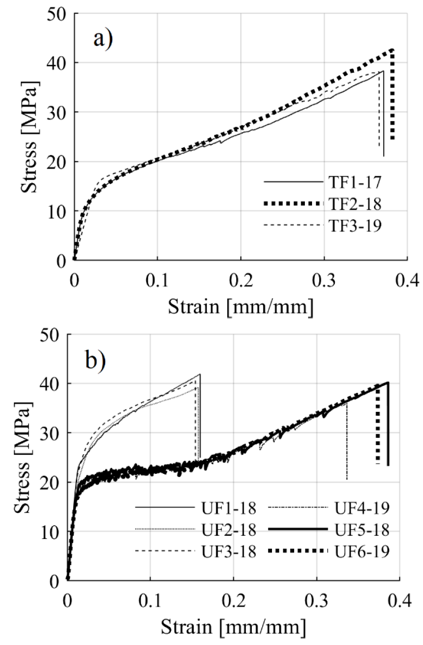
Figure 3 Tensile stress-strain curves for flax-polyester composite. a) Treated flax composite - Weft direction. b) Untreated flax composite - Warp and weft directions
Tensile tests over the cotton composite were done by applying the tensile force in the weft direction which has a bigger number of threads that the warp in this fabric (see Table 1]. Composites were reinforced with treated and untreated cotton fabrics in the range from 33 to 37% by weight. Results show that flax and cotton reinforced composites have a bilinear response [Figures 3 and 4). Composites reinforced with untreated cotton developed higher values of elastic modulus, yield strength and ultimate strength values than those reinforced with treated cotton.
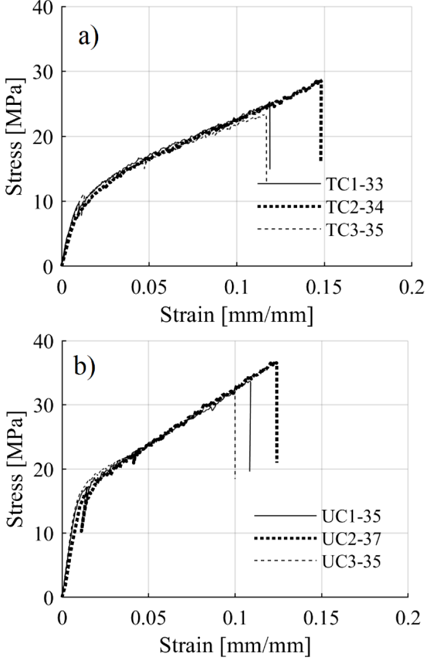
Figure 4 Tensile stress-strain curves for cotton-polyester composite. a) Treated cotton - Weft direction. b) Untreated cotton - Weft direction
In contrast to composites reinforced with flax or cotton, the stress-strain curves for jute reinforced composites [Figure 5] do not show yield points that can be clearly differentiated. Figure 5a shows that for treated jute composites the elastic modulus and ultimate strength decrease as fiber content increases, which could be explained by a poor adhesion between fibers and matrix or by a severe decrease in properties of the jute fibers caused by the alcali treatment. Evidence that supports this statement can be found in Figure 5b which, for untreated jute composites and similar fiber content (37-41%), shows higher ultimate tensile strengths and less ultimate tensile strain values. Even in the best case, shown in Figure 5b, the composite material does not seem to develop better properties than the polyester resin without any reinforcement, as can be evidenced by the modulus (E) and the ultimate tensile strength (SU) data shown in Table 3 for the polyester resin and the untreated jute.
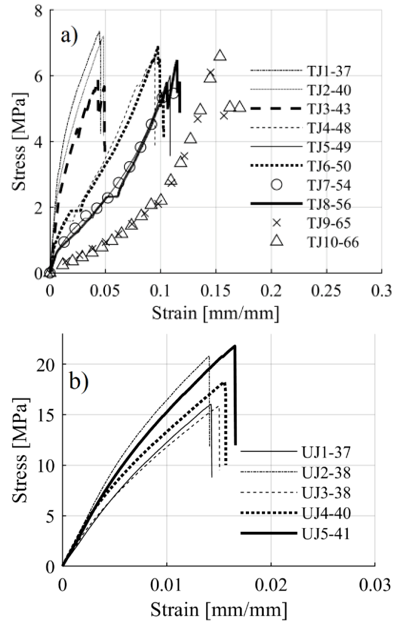
Figure 5 Tensile stress-strain curves for jute polyester composite. a) Treated jute - Weft direction. b) Untreated jute - Weft direction
Fracture strains presented in Figure 4b and Figure 5b show the low stretching of the jute and cotton composites when compared to the flax composite [Figure 3] where the higher strains were achieved for tests performed along the weft direction, probably due to the waving of the yarns and their stretching up to a straight configuration.
The difference in elastic modulus for an approximate percentage of reinforcement for the jute composites can be better seen in Table 3. This table summarizes the mean values of the mechanical properties measured by tensile test for the unreinforced polyester resin and the three types of reinforced composites. The stress-strain curve for the polyester resin (not shown) is linear up to the point of fracture. The abbreviations STD and CV found in the tables mean Standard Deviation and Coefficient of variation (see the ASTM standards).
Figure 6 allows comparison between the ultimate tensile strength and elastic modulus extracted from Table 3. The composites that develop higher ultimate strengths and elastic modulus under traction loads are those reinforced with untreated flax (UF18 and TF18), in contrast with those reinforced with treated jute (UJ39 and TJ40), which exhibit the lowest mechanical properties, which are even below the properties of the unreinforced polyester (P). After a review of Table 1 it can be found that flax fabric has a more compact configuration formed by a higher amount of yarns and with little separation between them, when compared to cotton and jute fabrics. As a result of this configuration, flax yarns can be impregnated by the resin in a more efficient way, which improves the adhesion, while in the cotton and jute fabric the resin wets the fibers of the surface of the yarn, leaving the innermost fibers dry; this and the separation between yarns produce a reduction of the reinforcing capacity of the fabrics.
3.2 Compression tests
Figure 7 shows the stress-strain curves for the treated flax composite. A reduction of elastic modulus, ultimate strength and ultimate strain is observed when increasing the fiber weight fraction in the composite. 100% polyester resin specimen has the higher ultimate strength and young’s modulus for the compression tests (see Table 4], which is explained by the fact that the resin is a reticulated rigid and solid polymer [30] that becomes weaker when the fiber fraction through the cross section of the specimen increases. This happens due to the nature of the vegetal fibers whose structure is composed of microfibrils of cellulose with tubular structures [31] that tend to collapse by flexure or crushing. The decrease of these properties caused by the increment of the fiber weight fraction can also be seen in Figures 8 and 9.
For the untreated flax composite with fiber weight fraction between 19 and 20 % wt. the stress-strain curves (not shown) were linear from the beginning up to the fracture point. The elastic modulus and compressive strength for the untreated and treated flax composite presented in Table 4 for similar fiber weight fractions (around 19% wt.) show that NaOH treatment drastically deteriorated these two properties in the composite. The stress-strain curves for composites reinforced with treated cotton at an interval between 33 to 43 % wt. is shown in Figure 8. A comparison between the treated and untreated cotton reinforced composites for similar fiber weight fractions (around 42% wt.) shows that compressive strength of the untreated composite is around 7.5 MPa while for the treated composite is around 16.5 MPa. This result may indicate that, for the cotton composite, alcali treatment seems to improve compressive strength performance.
The compressive stress-strain curves for composites reinforced with jute treated at an interval between 39 to 64 % wt. are shown in Figure 9. A comparison between the treated cotton composite and the treated jute composite for similar fiber weight fractions (around 39% wt.) shows that the compressive strength of the treated cotton composite is around 24 MPa, while the same property for the treated jute composite is around 8.5 MPa, which suggests that treated cotton reinforced composite could be more suitable to withstand axial compressive loads. Also, the analysis of results suggests that the effect of increasing the percentage of reinforcement is more important than the effects of the alkalization treatment on the decreasing of compressive mechanical properties, which suggests that an improvement in the manufacturing process could represent a higher increase of mechanical properties that improving the chemical treatment of the fibers.
Table 4 summarizes the mean values of the mechanical properties measured by compressive test in the three types of reinforced composites. Compressive stress-strain curve for the polyester resin without any reinforcement (not shown) shows a linear behavior until it reaches the fracture point at (0.2159 mm/mm, 77.61 MPa).
3.3 In-plane shear tests
The in-plane and out-of-plane shear tests were only performed on composites reinforced with untreated flax fabrics because they showed the best performance in tensile and compression tests. Stress vs. strain curves for the in-plane shear test are shown in Figure 10. A change from elastic to plastic strains can be seen at around 9 MPa; then above 13 MPa the material seems to show creep behavior. Ultimate strength was determined at 5% of shear strain due to the large deformation exhibited by the specimens [29]. The results for the in-plane shear modulus, ultimate strength and ultimate strain are summarized in Table 5.
For the in-plane shear tests, the specimens presented extreme fiber scissoring due to the large deformation obtained during the test. According to the standard, this behavior can be associated to ductile matrices or weak fiber/matrix interfaces. Fabrication of the specimens at relatively cold temperatures (around 14.2°C) could be one of the causes of this behavior because curing at temperatures below 15°C should be avoided as it can result in undercure [32].
3.4 Out-of-plane shear test
G ij shear modulus is measured by applying a shear force on the plane perpendicular to the i local axis in the direction of the j local axis. For this study, local direction 1 is parallel to the weft direction, while local direction 2 is parallel to the warp direction. Reinforcement orientation for these tests can be clearly seen by studying Figure 9 of Ref. [24].
The 1-3 shear stress vs. 1-3 shear strain curves can be observed in Figure 11a, while the 3-1 shear stress vs. 3-1 shear strain curves can be found in Figure 11b. Results for tests in these two directions are summarized in Table 6. More compaction between fabric layers was achieved due to the fabrication method used for the interlaminar shear test specimens, which explains the difference of the percentage of reinforcement of these specimens when compared to the same variable in the previous specimens tested.
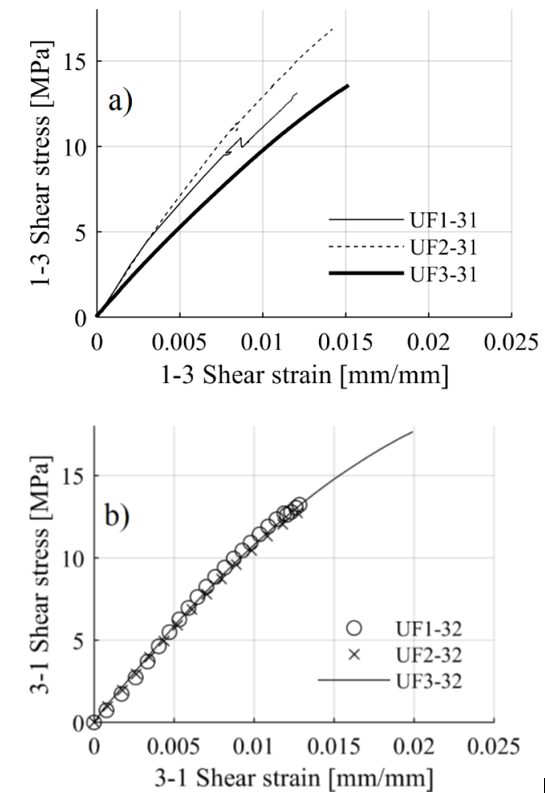
Figure 11 Interlaminar shear strain vs. Interlaminar shear stress curves for untreated flax fabric composite. a) 31% of reinforcement. b) 32% of reinforcement
The analysis of Figure 11 and Table 6 shows that the measured properties are quite close in the tested specimens (31% and 32% wt. of untreated flax). This result suggests that these shear properties are independent of the tested reinforcement orientations, so the mechanical performance of the composite under out of plane shear loads tends to depend on the matrix material for a fixed percentage of reinforcement.
Table 7 shows the results for the highest specific modulus and specific ultimate strength in tension and compression for the three composites in units of stress per unit of surface density of the fabrics. The performance of the untreated flax composite is the best among all the tested composites.
Table 7 Results for specific modulus and specific ultimate strength for tensile and compressive tests
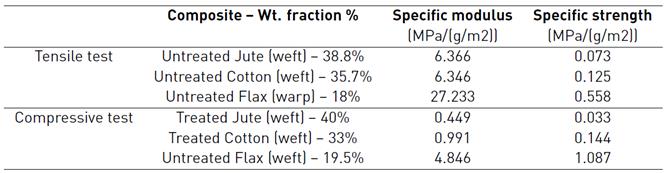
Some investigations about thermoset polymers reinforced with flax, bamboo, pineapple leaf, jute and kenaf, previously treated with alkaline NaOH solutions at concentrations between 5 and 20 % v/w, during 25, 30, 60 minutes and 24 hours, resulted in an increment of the mechanical properties of the fabricated composites. These results are different than the ones obtained at the present study, where elastic modulus and strengths measured in tension and compression are lower for the chemically treated vegetal fiber composites than those elaborated with similar proportions of reinforcement fabrics but without alkali treatment. An inconsistency can be encountered in the result for the strength and elastic modulus measured in compression for the cotton composite, which can be explained by the fact that the alkali treated cotton composite (TC33) contains a higher proportion of resin than the untreated cotton composite (UC42) [6, 7, 10, 11, 15].
The results of the present study agree with the results reported by other authors about the mechanical behavior of vinyl-ester, epoxy, polypropylene and polyester resin composites reinforced with jute, hemp, agave, coir and alpha fibers that were previously treated with 2 to 25% v/w of NaOH solution during 20, 60 minutes, 24, 48 hours, and 10 days. These studies present a decrease in the mechanical properties of the composites, mainly for elastic modulus and ultimate tensile strength [12-14, 16-18, 33, 34]. These papers suggest that the composite properties worsen with alkali (Sodium hydroxide NaOH) treatment at concentrations that are higher than optimal due to a reduction of the elastic modulus, diameter and bonding of the vegetal fibers related to an excessive extraction of lignin (bleaching and delignification), hemicellulose and OH group (damage on the cell wall). The effect of alkali treatment over the surface of jute fibers can be seen on Figure 12.
The difference between the results presented by the studies that describe an increase and those that describe a decrease in the mechanical properties of the composite could have been caused at some extent by the fabrication method of the composites. A study conducted by Gassan and Bledzky on a jute/epoxy composite (25% v/w by 25 min.) [33] concludes that, during alkali treatment, fibers suffer from shrinking, which reduces the elastic modulus of the composites. On the other hand, when the materials were fabricated in isometric conditions (i.e. restricting the contraction of the treated fibers) an increase up to 60% in the elastic modulus was obtained.
The results of this study suggest that polymeric matrix composite materials could improve their mechanical properties in tension by reinforcing them with thin vegetal fabric layers whose weft and warp are knitted with a high linear density of thin yarns. This configuration, like the one that is found in the flax fabric, allows for a better impregnation and penetration of the polyester resin between and towards the interior of the yarns, producing a better transfer of load produced by the stronger matrix-reinforcement bonding interface.
It is preferable to use the vegetal fabrics without alkali treatment but, in case it is used as a cleaning surface method, it is suggested to use low concentration alkali solutions and a lower immersion time to avoid deterioration of its mechanical properties. Regarding the behavior of vegetal composites under axial compressive loads, it was evident that, in general, for this kind of load, vegetal fabrics do not play a role as a reinforcement in the composites.
Mechanical behavior of thermoset and thermoplastic matrix composites reinforced with different vegetal fiber volume fractions and with a high linear density of yarns along its warp and weft directions will be studied next. Tests will allow to understand the influence of the pre-tensioning of the vegetal fibers when fabricating the composite using Vacuum Assisted Resin Transfer Molding (VARTM). This process will increase the compaction of the vegetal fabrics and will reduce air bubbles inside the composite material as usually happens when using the Hand Lay-up method (see Figure 13].
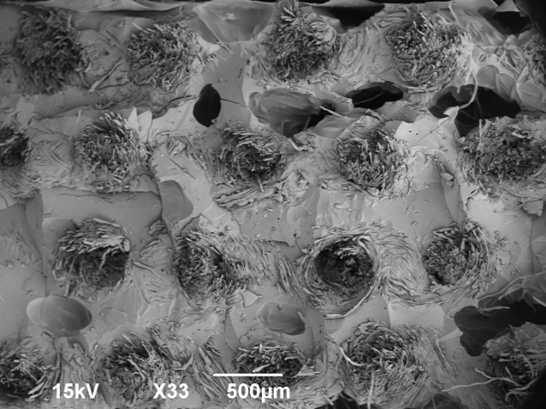
Figure 13 SEM image of air inclusions in cotton composite specimen fabricated using hand lay-up process
Related to the mechanical characterization of the materials, it must be seen that this study focused on the macro-mechanical behavior of the composites, i.e., the behavior when only averaged apparent mechanical properties are considered, so the properties were determined for specific percentages of reinforcement. On the other hand, the micromechanical approach could have included the individual properties of matrix and reinforcement and the fiber volume fraction of reinforcement as variables, so the properties would have not been limited to a specific fraction of reinforcement [35, 36]. This advantage from the micromechanics approach will be explored in future mechanical characterization studies.
4. Conclusions
The untreated flax fabric reinforced composite has the highest potential to be used for the manufacture of laminated composites between the three vegetal composites that were studied. Its specific tensile properties are higher than the same specific properties measured for the cotton and jute reinforced composites.
The excessive lignin and hemicellulose extraction due to alkali treatment for 48 hours could have affected the cementation role of these elements between the fibers, reducing their modulus and strength. The shrinking of the fibers due to the same treatment can also be an explanation for the reduction of mechanical properties of the composites that used treated fibers as the reinforcement. A reduction of the immersion time in NaOH solution and the manufacture of the composites in isometric conditions (without shrinking) seem to be a possible solution for this problem.
It is necessary to increase the fiber weight fraction of the composites to improve the load transfer to the fibers and optimize resin wetting, which can be achieved by improving the manufacturing process. Vacuum Assisted Resin Transfer Molding -VARTM seems to be an alternative to the hand lay-up molding process that could fulfill the before mentioned requirements and improve specific properties of the vegetal fabric reinforced laminated composites.