1. Introduction
Heavy metals are involved in all aspects of microbial growth and are mainly related to metabolic pathways [1]. There are some metals that are trace elements while others serve no essential function in microorganisms; however, when chemically similar elements may be replaced by some other [2]. Within cells, Cadmium (Cd) can damage the integrity of the cell membrane, block enzymes, inhibit transport systems and move items which are essential nutrients in cellular metabolism [3].
With the increase of industrial activities in recent decades, generation of waste polluted with heavy metals such as Cd has also increased. This contaminant has serious consequences in human health. Besides, heavy metals deteriorate aquatic ecosystems because they bioaccumulate easily inside the organisms affecting the food chain by a multiplier effect, a problem that is increased by their chemical stability towards biodegradation processes [4].
Cadmium is a heavy metal widely used in galvanoplasty industry, in semiconductor alloys for home appliances [5] and in the elaboration of pigments for textile industry and photography. At lower concentrations, Cd can come out harmless to humans and animals, but because of the bioaccumulation, it can damage the cell wall integrity, which affects organs such as kidneys, liver and lungs. It also causes disruption of mithocondria and endoplasmatic reticulum leading to the release of calcium within the cell, activating mechanisms of autophagy and apoptosis in some cells [6], and replacing elements which are essential nutrients in cell metabolism.
Bioremediation is a biological treatment which consists in the use of living organisms such as plants, microorganisms, fungus, and others, to degrade or transform pollutants. The use of biological treatments in some circumstances can turn out to be more convenient since it is not as expensive as other treatments and the resulting products are innocuous. Microalgae are organisms that can be used in this kind of process since they are not only food in aquatic environments, but they can also treat waste water from industrial activities [7]. Bioremediation with microalgae have been successful because these microorganisms are able to assimilate pollutants such as heavy metals and interact with them by the means of changes in the ion permeability, active exclusion, extracellular and intracellular chelation and cellular compartmentalization of the heavy metal [8, 9].
The present study aims to evaluate the potential of three native strains of microalgae from the genus Ankistrodesmus, Chlorella and Scenedesmus in the removal of cadmium in synthetic water looking to generate bioremediation processes using native microalgae.
2. Experimentation
2.1 Microorganisms and culture conditions
For the tests, three strains of native microalgae of Chlorella, Scenedesmus and Ankistrodesmus genus isolated from different sources of urban and rural water in Antioquia department were donated by the research team GRINBIO at University of Medellin. For maintenance and tests of tolerance, microalgae were grown in 250 mL Erlenmeyer containing 150 mL medium 3N + V BBM which comprises NaNO3 (0.75 g.L-1), CaCl2.2H2O (0.025 g.L-1), MgSO4.7H2O (0.075 g.L-1), K2HPO4 (0.075 gl.L-1), KH2PO4 (0.175 g.L-1), NaCl (0.025 g.L-1), Na2EDTA4 (5 mg.L-1), FeCl3.6H2O (0.5874 mg.L-1), NaMoO4 (0.024 mg.L-1), ZnCl2.6H2O (0.03mg.L-1), MnCl2.4H2O (0.246mg.L-1), CoCl2 6H2O (0.012 mg.L-1), Tiamina HCl (0.0012 mg.L-1) y Cianocobalamin (0.00001mg.L-1) [10]. For Cd tolerance tests, cultures were prepared using a metal stock 1000 mg L-1 (brand HACH®) added to the growth medium at different concentrations as shown below, except for the control group which did not have any trace of the heavy metal. Microalgae were cultured for 12 days under controlled laboratory conditions at 22 ° C and constant light (4,100K 17-Watt Cool White Fluorescent Tube Light Bulb) and for each sample concentration there were set two other flasks in order to ensure repeatability.
2.2 Lethal concentration (LC 50 ) test
The median lethal concentration (LC50), which is a measure of the lethal effect of a substance in 50% of a population was used as the toxicological endpoint to find the acute effects of Cd in microalgae from an Probit analysis [11].
To determine LC50, culture media were contaminated with 0, 2.0, 4.0, 8.0, 12.0, 16.0, 25.0 and 40.0 mg L-1 of Cd to the species of the genus Chlorella sp. and Scenedesmus sp. Lower doses of 0, 2.0, 4.0, 6.0, 8.0, 12.0 and 20.0 mg L-1 were used Cd for Ankistrodesmus sp, as previous studies (unpublished data) showed its sensitivity to metal. Assays were performed in total volumes of 5 mL of isotonic solution (0.9% NaCl) inoculated with 10% microalgae culture, and contaminated with metal at different concentrations. The LC50 was evaluated after 96 hours of culture [12]. To determine the effect of time of exposure to Cd on the feasibility of microalgae, sampling after 0, 48 and 96 hours of culture were performed. Viability was quantified using the method of Evans blue staining [13]. Assays were performed in triplicate. During counting, the samples were kept in dark in order to avoid factors that promote the growth of microalgae.
2.3 Growth curves
To determine the effect of Cd concentration on the kinetics of growth of microalgae culture at concentrations of 0.0 (control group) 0.5, 1.0, 3.5, 5.0, 7.0, 10.0 and 15.0 mg L-1 of Cd were used. The cell density was determined by microscopic observation and cell counting using a Neubauer chamber. The dry biomass was found using gravimetric techniques proposed by Arredondo Vega & Voltolina (2007) [14].
To determine the effect of Cd on the production of pigments (chlorophylls and carotenoids) 4.0 mL samples were taken every 3 days. The quantification of the pigments was performed spectrophotometrically following the methodology proposed by Parsons Strickland [15].
The specific growth rate is determined using equation (1) Where x1 and x2 are cellular concentrations at times t1 and t2:
2.4 Determination of Cadmium removal percentage
After evaluating the tolerance of the strains of microalgae to Cd, the most resistant strains were cultivated in concentrations of 0.0 (control group) 1.0 and 7.0 mg L-1 Cd in order to determine their ability to remove Cd. Assays were performed in triplicate.
Cadmium analysis was made after 7 days to ensure that microalgae were in the final phase of growth near the stationary phase. The process of preservation and digesting for analysis of Cd was performed using the methods proposed in the Standard Methods of the examination of water and wastewater for dissolved metals [16] for which the samples were preserved at 4 ° C, acidified with nitric acid, and then an acid digestion was performed. Finally, Cadmium determination was performed by mass spectrometry.
3. Results and discussion
3.1 Median lethal concentration (LC 50 )
After 96 hours of culture, the analysis of LC50 showed values of 20.89 mg L-1, 8.00 mg L-1 and 5.43 mg L-1 for Scenedesmus sp., Chlorella sp. and Ankistrodesmus sp. respectively (P-value<0.05) [Figure 1]. Ankistrodesmus sp. was the least tolerant microalgae to this particular heavy metal and in contrast Scenedesmus sp. presented the highest tolerance of the three genus studied. When comparing our results with those reported by other authors who found that cadmium, in concentration of 7.6 g, decreases cell growth by 50%, it is possible to evidence the tolerance of this microalgae [17]. In the case of Chlorella vulgaris, it has been reported that at concentrations varying from 5.1 to 15.72 mg L-1, this heavy metal causes acute toxicity evidenced in an inhibitory effect on the growth of this species [18], and comparing these results with the ones found in the present work, it can be said that Chlorella sp. had a response to the exposure of Cd that is within the range mentioned above. However, the disparities on LC50 values found on the literature can be explained by the environmental conditions such as temperature, light, initial cellular density, and culture medium that were used in each of those experiments, which can alter the algae response towards Cd even if the same species and heavy metal are used [19]. Results confirm the importance of assessing toxicity in terms of Median Lethal Concentration (LC50), because when exposing microalgae to a heavy metal such as Cd, it is possible to generate interactions between these organisms and the heavy metal, which can cause different responses such as growth rate inhibition or cell death that are reflexed in the value of LC50 [20].
3.2 Cadmium effect on the growth curves
Regarding the toxicity of Cd for the three microalgae species in the case of Ankistrodesmus sp. it is possible to identify a reduction on the growth with the increase of the Cd concentration in the medium [Figure 2]. It can be observed from the graphic that there are three groups of kinetics: The first group is represented by cultures with absence or lower Cd concentration (0.0, 0.5, 1.0 mg L-1). Control group (without heavy metal), reached the maximum biomass density of 4.00 x 105 cell mL-1 after 12 days of culture, when the death phase started. Cultures exposed to concentration of 0.5 and 1.0 mg L-1 of Cd have maximum densities of 3.75 x 105 cell mL-1 and 2.75 x 105 cell mL-1 after 9 days of culture, respectively. For these cultures a little affectation was manifested in an extension of the initial adaptation phase.
A second group of kinetics is represented by cultures with mean concentrations of Cd (3.5 y 5.0 mg L-1). They reached the maximum biomass density of 2.00 x 105 cell mL-1 and 1.50 x 105 cell mL-1, respectively, after 3 days of culture, when cell growth is suspended. It is possible to infer that cells begin to die at concentrations of 5.0 mg L-1 of Cd because their growth is poor and they remain in the adaptation phase for ten days.
The third group of kinetics is represented by cultures with highest Cd concentration (7.0, 10.0, 15.0 mg L-1). These cultures have important affectations that are manifested with an initial cell death, an extension of the adaptation phase, and lower peaks of biomass densities around 1.00 x 105 cell mL-1 reached after 3 days. Although from the Lesser Significant Difference Test (LSD) of the one-way ANOVA it was ratified that the distinct Cd concentrations in the mediums have a significant influence on the population density (P-value <0.05) and growth rate of Ankistrodesmus sp. (P-value <0.05) [Table 1], the test clearly differentiates two groups of kinetics, in which cells at 0.0, 0.5 and 1.0 mg L-1 behave significantly different in terms of growth than the ones from the cultures of 3.5 mgL-1 of Cd and higher.
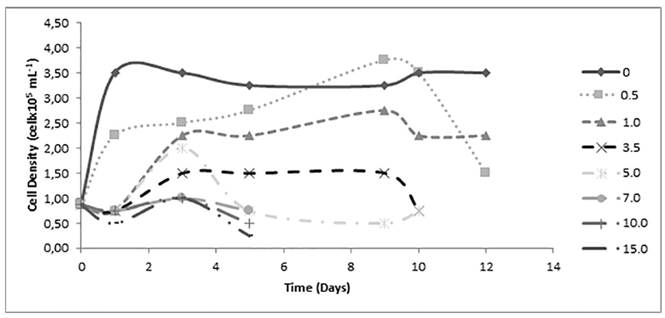
Figure 2 Effect of the concentration of Cd on the cellular density of Ankistrodesmus sp. during 12 days of culture
Table 1 LSD test for maximum cell density achieved by the microalgae (One-way ANOVA)

*Same letter represent homogeneous groups within the specific genus. All values are in cells x 106 mL-1.
Generally speaking, growth of Scenedesmus sp. is more prosperous than Ankistrodesmus sp. reaching a maximum value of 1.9 x106 Cells mL-1 after ten days of culture. In relation to toxicity, the Cd effects are lower for Scenedesmus sp. than Ankistrodesmus sp., evidenced in the analysis of the effects caused by the Cd on the growth of the cultures of Scenedesmus sp. performed by Fisher’s LSD test where it did not show differentiation among treatments [Table 1]. For this microalgae, the highest values of cellular density are observed at 0.5 mg L-1 of Cd. From Figure 3, it is possible to identify three groups of kinetics for Scenedesmus sp. too. The first group is represented by cultures with absence or lower Cd concentration (0.0, 0.5, 1.0 mg L-1) which reached the maximum biomass density of 1.92 x 106, 1.97 x 106 and 1.80 x 106 cell mL-1 after 12 days of culture, respectively, when the death face began. These three cultures have short initial phase of adaptation, evidencing its tolerance to low doses of the metal, where the highest values of population density are observed at 0.5 mg L-1 of Cd. A second group of kinetics is represented by cultures with mean concentrations of Cd (3.5 y 5.0 mg L-1), they reached the maximum biomass density of 1.62 x 106 and 1.30 x 106 cell mL-1 after 5 and 7 days of culture respectively. The third group of kinetics is represented by cultures with highest Cd concentration of 7.0, 10.0, 15.0 mg L-1 with the toxic effects evidenced by decreasing of the cellular density with values of 8.75 x 105, 9.00 x 105 and 1.00 x 106 cell mL-1 at the end of the experiments.
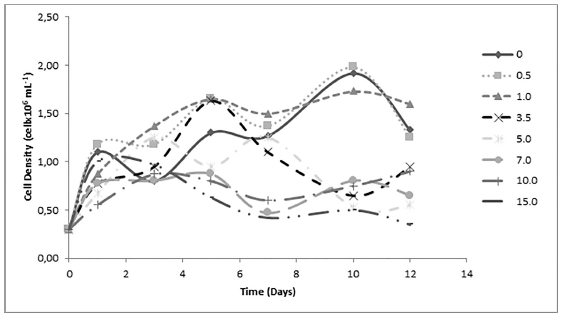
Figure 3 Effect of cadmium concentration on Scenedesmus sp. cell kinetics growing during 12 days of culture
The general growth of Chlorella sp. is higher than the growth of the other two strains of microalgae evaluated in this study reaching a maximum value of 3.8 x 106 cell mL-1 after ten days of culture under control conditions without Cd [Figure 4]. Regarding toxicity of Cd, Chlorella sp. at low concentrations of 0.5 and 1.0 mg L-1 of Cd, has a growth-enhancing effect that raises growth peaks to values of 2.52 x 107 and 7.65 x 106 cell mL-1, respectively. According to Fisher’s LSD test made for Chlorella sp. differences between the means of population density for each concentration of the heavy metal are not significant with the exception of the treatment at Cd concentration of 0.5 mg L-1, which reported a higher value indicating that Cd at this concentration has an important promotion of cellular growth [Table 1]. Besides, all kinetics, except that obtained using 0.5 mg L-1 of Cd, have similar tendencies to the control, and the cultures with 10.0 and 15.0 mg L-1 Cd entered death phase and were suspended on day 9 evidencing a toxic effect of this heavy metal.
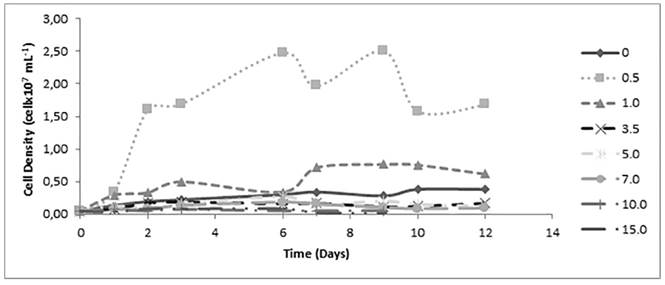
Figure 4 Effect of cadmium concentration on Chlorella sp. cell kinetics growing during 12 days of culture
In general, growth curves of the cultures indicate that the biomass increases through time, and because of the conditions of the environment in which cells developed, the speed of that growth varies; different phases are exhibited describing the changes of cell concentration. In the present study, the phase of adaptation of the microorganisms to the environment is not shown (lag phase) due to its brief duration of less than 24 hours. Exponential phase, where there are no growth-limiting factors, and stationary phase, in which growth-rate and death-rate are equal, varied according to the Cd concentration in the medium and how fast the death phase was achieved, in which death-rate surpassed the growth-rate. Cultures used in the present work did not manifest inhibition of the biomass increase in mediums with Cd at 0.5 mg L-1. Even though for Scenedesmus sp. and Ankistrodesmus sp. kinetics behavior at the mentioned concentration were not very different from the control group, in the case of Chlorella sp., this amount of Cd in the medium stimulated its growth and reported the highest growth-rate and maximum population density than at any other concentration. This can be explained since there are some metals like Cd that can replace other elements such as Ca and Fe, going through their specific transporters and carriers, getting into the cell. On the other hand, there is also evidence that suggests that in presence of cadmium, algae are able to produce polysaccharides on the cell wall as a protection mechanism against the heavy metal toxicity, which stimulates metabolic activity within the cell [9, 18, 21]. As a result, at low concentrations the heavy metal is not harmful for the microalgae and it does not affect negatively the cell growth.
Based on the dry weight data of Anikistrodesmus sp., from the one-way ANOVA, it was found that different concentrations of Cd have a significant influence on the values of total dry weight (P-value <0.05). The highest value of the parameter reached by the microalgae was at a Cd concentration of 1.0 mg L-1 [Figure 5] and from this point, the inhibition of the culture growth starts to increase as the concentration of the heavy metal becomes greater.
For higher concentrations of the heavy metal Scenedesmus sp. is the microalgae with the highest biomass dry weight value, reaching its maximum (36.15 µg mL-1) at a Cd concentration of 3.5 mg L-1. This indicates that the mentioned genus is more tolerant to higher heavy metal concentrations, which coincide with the values found in LC50 tests. Besides, it is consistent with the results found for Scenedesmus abundans where the response of this species at a Cd concentration range of 0.0 - 20.0 mg L-1 was analyzed, and negative effects were not observed on the viability of the cultures exposed to the heavy metal concentration up to 10.0 mg L-1 [22].
Based on the results obtained from the dry weight data of Chlorella sp., the highest biomass was achieved at a Cd concentration of 0.5 mg L-1. From Fisher’s LSD test, it is shown that the culture at this particular concentration is statistically different from the other groups of Cd concentration (data not shown). From concentrations of 3.5 mg L-1 and higher Chlorella sp. is less tolerant to the heavy metal, that behavior is reflected on the biomass growth and in the low values of total dry weight.
3.3 Cadmium effect on pigments
Pigment analysis evidenced that chlorophyll concentration for the three strains was significantly affected by cadmium concentration in the medium (P-value <0.05) and three homogenous groups were detected [Table 2]. In this case, Cd concentration of 0.5 mg L-1 gave the highest concentration of chlorophylls reported in the present study, being the mean concentration of this pigment 1.15 µg mL-1.
The effect of Cd toxicity over the carotenoids and chlorophylls production is associated with the Cd concentration and genus of microalgae. Chlorophylls production at different Cd concentration have a similar behavior with the control not contaminated for Scenedesmus sp. (near 0.3µg mL-1) (Data not shown). In general, pigments (chlorophylls and carotenoids) production is reduced for Scenedesmus sp. and Ankistrodesmus sp. at low concentrations of Cd. However, Chlorella sp. reports higher chlorophylls production at 0.5 mg L-1 Cd when compared with the control group. This behavior is related with the growth, indicating that low concentrations of Cd promote growth and pigment production in Chlorella sp.
The low cadmium tolerance presented by Ankistrodesmus sp. could be related to inhibition in the enzyme production, responsible for the catalysis that takes place in the biochemical reactions within cells [23], inhibition of protein synthesis and degradation of cell pigments, which interferes with photosynthesis and eventually causes death [24]. According to other researchers, Ankistrodesmus sp. is more likely to be found in waste water polluted with organic compounds, rather than environments with inorganic pollutants such as heavy metals. Nevertheless, some species of this genus, for instance A. braunii, have been studied for their capability to accumulate cadmium through the cell wall bonds for short periods of incubation [25, 26] although there is also important to consider the fact that the obtained response of this genus might be related as well to the genetic predisposition of the strain used for this study, and not only restrained to environmental conditions.
Chlorophylls and carotenoids participate in the capture of solar energy which is use for the synthesis of organic compounds necessary in cellular processes [27] and when expose to heavy metals, it is known that Cd is capable of being incorporated in green pigments forming heavy metal substituted chlorophylls, replacing Mg +2, which diminishes photosynthetic processes, behavior that has been observed in Scenedesmus quadricauda [28]. This is why, when analyzing the concentrations of these pigments in the study, it can be said that microalgae cellular processes were not much affected by lower Cd concentrations in the medium and just Scenedesmus sp. reported a better photosynthetic response at higher concentrations of the pollutant.
3.4 Cadmium removal from the medium
The highest removal percentage at low initial concentration in the medium (1.0 mg L-1) was achieved by Chlorella sp. (8.07% compared with 5.13% removed by Scenedesmus sp), however when the Cd concentration was increased to 7.0 mg L-1 Scenedesmus sp. reach a 32.74% of Cd removal on the culture media [Table 3].
It is known that Scenedesmus incrassatulus was able to remove the 24.1% of cadmium in water contaminated with 7.36 mgL-1 of the metal [17], Scenedesmus abundans removed the 97.4 % of cadmium in media with initial concentrations of 10 mgL-1 metal, reaching a final concentration of 0.26 mg L-1 [22] for Chlorella vulgaris exposed to 3 mg L-1 Cd, removed between 51.4 and 96.5% [29] and for this same species, it was found that for very short periods of time it could remove 90% of the heavy metal in 30 minutes when suspended in alginate of sodium, and up to 90% of Cd when varying pH from 1 to 6, reaching its maximum removal at 4.5 for 2 h [30, 31]. The percentages of removal reported in literature are higher than those found in this work, this is probably because they used strains previously exposed to some metal, different carbon sources, time of exposure, pH and other conditions which enhanced the microalgae tolerance to cadmium and the ability to remove it from the medium.
Regarding the maximum biomass density, total dry weight and pigment concentrations, Chlorella sp. reported a better response at lower Cd concentrations while Scenedesmus sp. behaves better at higher concentrations of the heavy metal which reflects higher tolerance of Cd.
Microalgae Cd removal capacity obtained at high Cd concentration and LC50 values was related. It was observed that Chlorella sp. (with LC50 8.0 mg L-1 of Cd) had a lower removal percentage (equivalent to 8.6%) than Scenedesmus sp. (with LC 50 20.0 mg L-1 Cd) which get a removal percentage of 32.74%. This microalgal response supports that the native Scenedesmus sp. isolated could be a microalgae useful for Cd water bioremediation. Heavy metal tolerance of microalgae is a consequence of different cellular strategies including volatilization, accumulation or formation of chemical complex with extracellular compounds [32].
Chlorophytes cell wall is composed of polysaccharides, mainly cellulose [33]. Scenedesmus sp. has a cell wall composed of multiple layers and excretes extracellular polymeric substances with anionic properties; some heavy metals such as Cd can be accumulated in this cell wall [30], which acts as a cation exchanger bounding metallic cations; this behavior can be an advantage to limit the toxicity when the microalgae are exposed to great concentrations of the metal in the nature. In the case of Chlorella sp., the variation of the cell wall of a single species under different conditions can be very large, which makes it difficult to know exactly what kind of compounds are found in it [34], and the polysaccharides located in the cell wall might have different mechanism of reaction than the ones in Scenedesmus sp. cell wall and may not give a protectant effect that allows growth at greater Cd concentrations. Tolerance in some cases can also be due to transporter proteins which mediate heavy metal movements through membranes, and some of them are classified as cation diffusion facilitator (CDF) that are involved in the heavy metal homeostasis [35]. Furthermore, this group of proteins can expulse from the cell cations such as Cd through ion exchange in a chemiosmotic process [36].
Colombia has a wide diversity of microalgae [37] with great potential for the treatment of water polluted with Cd or other heavy metals. Besides, the results found in the present study show important percentage of removal which can lead to more studies with other native microalgae species that can possibly generate a similar response towards Cd as it was found in this research.
4. Conclusions
The experimental results of this research show that Cd tolerance depends on the genus of microalgae as well as on the cadmium concentration in the culture medium. Ankistrodesmus sp. was the microalgae with the lowest resistance to Cd toxicity measured as LC50, while Chlorella sp. and Scenedesmus sp. had higher tolerance. Furthermore, growth biomass measured as total dry weight and growth curves show that at higher metal concentration Scenedesmus sp. is the one with the best response. In general, low Cd concentrations did not affect negatively the growth of microalgae and there was found positive response in Chlorella sp. Regarding the Cd removal, it was found that Scenedesmus sp. removed 32.74% of the heavy metal at 7.0 mg L-1 in the medium. Chlorella sp. had a removal percentage of 8.07%, at an initial Cd concentration of 1.0 mg L-1 although it is relevant to perform removal kinetics in further studies in order to identify the accumulation processes that may occur in these microalgae.
With the strains of microalgae used in this study, it is recommended to make more comparative assays in mediums with different carbon sources in order to reach higher cell densities, it is also recommended to vary light and dark periods of exposition of the cultures, pH and temperature, expose microalgae to gradual concentration of Cd and compare the percentage removal with the results obtained in this study, and use microalgae previously exposed to Cd or another heavy metal since these are strains that possibly develop higher tolerance to greater heavy metal concentrations and might have major removal percentage.