1. Introduction
The dumping of heavy metal-contaminated effluents into water bodies is one of the most severe environmental problems in recent years[1]. Heavy metals such as cadmium, chromium, lead, zinc, and nickel discharged into water sources can accumulate in flora and fauna, other organisms and eventually reach the human food chain causing various health problems[2]. Nickel is used in several applications, such as electroplating, dyeing, battery manufacturing, tanneries, paint formulation, ceramic and porcelain glazing, among others[3].
Nickel is not biodegradable and is particularly harmful to humans and other organisms, even in minimal concentrations; it can cause dermatitis, long-term exposure to high doses of nickel can lead to kidney and lung damage, pulmonary fibrosis, gastrointestinal failure, renal oedema, gastrointestinal disorder, cancer and teratogenicity[4]. Besides, nickel carbonyl that is easily absorbed into the skin has been reported to be lethal in humans at atmospheric exposure of 30 mg/L per 30 min[5]. The US-EPA established a maximum concentration of 0.5 mg/L of nickel in drinking water[6]; therefore, the treatment of effluents contaminated with this metal is a priority.
The development of technologies for removing heavy metals is a challenge because conventional techniques such as electrochemistry, membrane treatments, oxidation, precipitation, and heterogeneous photocatalysis are expensive due to the operating costs and reagents required[7]. Thus, bio-adsorption is presented as an economical and environmentally friendly alternative, which allows the recovery of waste as contaminant adsorbents due to the presence of active centres in its lignocellulosic structure[8]. Different biomasses have been used to remove heavy metals such as lychee[9], Orange[10], Pisum sativum[11], yam[12], lime[13], cranberry seed peel, rosehip seed peel, and banana peel[14], rambutan peels[15], macaúba[16], sweet lime and lemon peel poder[17], which reported good yields and adsorption capacity.
On the other hand, more than 102 million plantains are produced annually, with 35% of each fruit producing approximately 36 million tons of waste after its exploitation[18]. In Colombia, a large number of Musa paradisiaca plantain crops in the department of Bolívar have generated a significant increase in post-harvest waste because their production is focused on marketing or as a household food option. After selecting the fruit with the best conditions, those that do not meet market demands in terms of size and plantain maturity are used as fertilizer for the harvest through decomposition, representing 10% of the total plantain crop produced. Therefore, in this research, the residual biomass of the plantain starch production process is proposed as a nickel Ni (II) bio-adsorbent in solution in a batch system, evaluating the effect of temperature, adsorbent dose, and concentration of the material in the adsorption.
This work provides the process operating conditions for implementing the Ni (II) removal method by biosorption, as an efficient technology, easy to implement, and friendly to the environment, simultaneously solving the disposal and use of large volumes of agro-industrial residues from plantain. This topic contributes to the state of the art of the subject of clean technologies because it presents an efficient alternative, with low energy consumption and without chemical reagents; therefore, it can be considered a low cost and easy to implement solution. These previous results contribute to the applied engineering literature for the implementation of the technology on a pilot scale with real wastewater. It also highlighted a contribution to studying the agro-industrial waste from the process of obtaining plantain starch, which is part of a series of studies for the implementation of a biorefinery for processing various agricultural products of the Caribbean region, such as yam, yucca, and plantain. It is projected to process and obtain starch from these three raw materials, to establish a bio adsorbent production line taking advantage of the waste from this process.
2. Methodology
2.1 Experimental design
An experimental design following a continuous linear factor on central compound response surface, star, was developed in the Statgraphics Centurion XVI.II. Software. In Table 1, the experimental ranges and levels of variation of the variables are shown. In Table 1, the experimental ranges and levels of variation of the variables are shown.
2.2 Preparation of the bio-sorbent and the synthetic solution
Post-harvest plantain waste was obtained in the municipality of Santa Catalina de Alejandría in the department of Bolívar (Colombia). The residual biomass of plantain starch was obtained as proposed by Maniglia and Tapia-Blácido[19]. Initially, the fruit was peeled, cut, washed with distilled water, and dipped in a 0.25% NaOH solution; the mixture was refrigerated for 18 h, reduced in size, filtered. Subsequently, the residual bagasse was adjusted to 7 and dried at 40 ºC to a constant mass. The residual biomass was sieved in an Edibon Orto Alresa Vibro sieve shaker.
A Ni (II) base solution was prepared at 1000 mg/L using Merk Millipore analytical grade nickel sulphate (NiSO4); 4.479 g of reagent was added to 1 L of deionized water. The solution was then diluted to reach the concentrations in Table 1.
2.3 Adsorption tests
The Ni (II) adsorption tests were performed individually in a Gemmy Industrial brand IN-666 shaker incubator. For this purpose, 100 mL of solution were taken at pH 6 and 200 rpm for 24 h, following the design of experiments in Table 1[20]. The remaining metal concentration in the solution was determined by atomic adsorption at 232 nm. Adsorption efficiency and capacity were determined according to Equations (1) and (2):
Where qt (mg/g) is the adsorption capacity, %E is the adsorption efficiency, Ci (mg/L) is the initial concentration, Cf (mg/L) is the final concentration, m (g) is the mass of the adsorbent, and V (L) is the volume of the solution.
2.4 Kinetics and adsorption isotherms
Kinetics studies were performed at the best experimental conditions of temperature, adsorbent dose, and initial concentration using 100 mL of solution, at pH (6) and 200 rpm. The samples were placed in the orbital shaker, and aliquots were taken at each time interval (5, 10, 15, 20, 30 min, 1, 2, 4, 8 and 22 h) to determine the behaviour of the contaminant over time[21]. The experimental data were fitted to the pseudo-first-order, pseudo-second-order, and Elovich models. Adsorption isotherms were performed by varying the initial concentration of the contaminant (50, 100, 200, and 300 mg/L) to determine its influence on the process as it reached equilibrium. The experimental data were adjusted to the Langmuir and Freundlich models. The kinetic and isothermal fitting models are shown in Table 2.
2.4 Thermodynamic study
The graphical method based on the Van't Hoff equation was used to determine the spontaneity, type of adsorption, and to predict the magnitude of changes on the surface of the adsorbent, respectively. Therefore, the change in Gibbs' standard free energy (∆G), enthalpy (∆H), and entropy (∆S) were estimated by testing the percentage removal of the samples at 33.8, 40, 55, 70, and 76.2 ºC. Then the final concentration of the metal after adsorption was determined, and the amount of metal removed was estimated. Subsequently, an Ln (Kc) vs. T-1 graph was made, and the thermodynamic parameters were determined[23]. The thermodynamic parameters mentioned were calculated according to Equations (3), (4), and (5).
3. Results
3.1 Characterization of the biomaterial
The elemental characterization showed that carbon with 47.74 % was the element with the highest content in the residual starch biomass, followed by oxygen and hydrogen. The results of the elemental analysis are shown in Table 3.
The chemical analysis presented in Table 4 shows that the high presence of carbons, oxygen and hydrogen in the biomass indicated in Table 3 is due to the 41.80% content of cellulose. Lignin and hemicellulose are present to a lesser degree. The high content of cellulose and lignin in the bio-sorbent under study corresponds to the presence of carbon and oxygen from the elemental analysis. The existence of these compounds in bio-sorbents with lignocellulosic origin offers active centres for the union of metallic ions because of the appearance of the hydroxyl group in the material's structure. Consequently, the material under study is massively useful when applied in the process of adsorption of metallic ions due to the cationic nature of these contaminants[24, 25].
The Fourier Transform Infrared Spectroscopy (FTIR) technique was used before and after Ni (II) adsorption [Figure 1], in order to determine the functional groups present in the biomaterial that could be involved in the ion exchange between the adsorbent and the metal. The IR spectrum of the residual plantain biomass before the adsorption process registers vibrations at wavelengths between 3000 and 3500 cm-1, indicating the presence of hydroxyl (-OH) functional groups; between 3200 and 3500 cm-1 is observed the appearance of amines and small carbonyl overtone signals due to the stretching vibrations of the -OH link[25]. The possible disturbances of C-H methyl, methylene, and methoxy groups are given by the peak of 2927.94 cm-1[26]. Wavelengths between 2000 and 2500 cm-1 show slight signals of alkynes and carboxylic acids[27].
Additionally, the FTIR spectrum has bands close to 1650 cm-1 that indicate the existence of functional groups of cellulose, hemicellulose, and lignin due to the stretching of C=O and C-O by vibrations of carboxyl groups of hemicellulose and lignin[28, 29]. This fact indicates the strong presence of aromatic rings produced by hydrogen vibrations representing the stretching of C=C. Between 1000 and 1200 cm-1, it is evident the presence of primary, secondary, and tertiary alcohols, a product of the stretching vibrations of the C-OH bond, and the flexions between the plane (1225-950 cm-1) are only complementary signals because of the C-C, C-N, and C-O stretch fall in the same region. Also, several signals appear depending on the hydrogen content, which could affect the interactions that rule the adsorption phenomena when the adsorbate interacted with the carbonyl groups or surface alkenes of the bio-sorbent[29]. The presence of the above-mentioned functional groups in the 400-4000 cm-1 spectrum is attributed to the high content of lignin, cellulose, and hemicellulose in the structure of the bio-sorbent extracted from the residual biomass from plantain starch[30].
The infrared spectrum after ion removal shows a remarkable increase in the width of the spectral bands after the Ni (II) adsorption process. Spectrum changes were observed in the 1000 cm-1 band due to bond N-H bending and a shift towards a lower band. Therefore, it is concluded that the nitrogen atom should be the leading adsorption site for Ni (II) binding in the biomass. Furthermore, changes in the FTIR spectrum at wavelengths 3500-3408.0 cm-1 and 1100.3-1090.4 cm-1 can also be assigned to the stretching vibration in the OH groups and the C-O stretching vibration, respectively[31]. Another significant change in transmittance is observed in the wavenumber 2904.6-2835.2 cm-1 after the adsorption. This is attributed to the C-H methyl, methylene, and methoxy groups vibrations present in the biomass. The above is consistent with the results obtained by different authors[32].
Figure 2 shows the SEM micrographs at a magnification of 200x of the residual plantain starch biomasses before [Figure 2a) and after Ni (II) adsorption [Figure 2b). A smooth surface structure is shown, with small beads that are attributed to the presence of lignin, hemicellulose, and cellulose[33]. In contrast, the plantain starch biomass micrographs after Ni (II) adsorption are shown in Figure 2. They exhibit a clearly soft and porous structure. The presence of pores and cavities on the surface is equivalent to the increased surface area accessible for adsorption. It can be observed that there is no precipitation of the metal on the surface of the adsorbent. So, it can be said that chelate formation does not appear, and the adsorption occurs by the interaction of electrostatic forces. The adsorption of the ions occurs inside the pores and cavities of the material[34]. These results are consistent with those found when using Citrus limetta for adsorption of Ni (II)[35], Citrus limettioides[36], coconut sawdust, and bagasse[37]. Previously, we reported for the residual biomass of plantain starch a surface area of 3.859 m2/g, a pore volume of 4x10-7 m3/g, and pore size of 4.89x10-9 m; thus, it can be said that the bio-adsorbent has the presence of mesoporous, with a small volume and surface area[38].
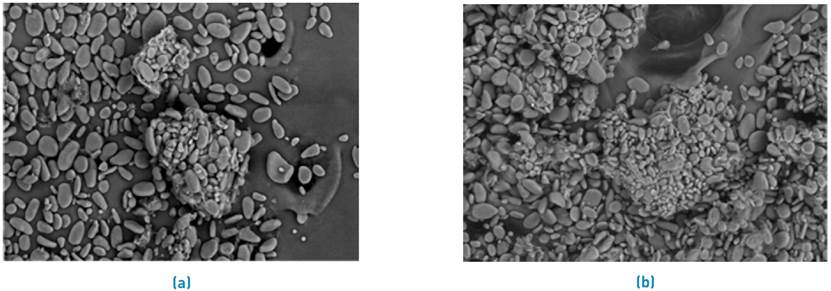
Figure 2 SEM microphotographs of residual plantain biomass (a) before and (b) after Ni adsorption (II)
Figure 3 shows the EDS spectra of the residual plantain biomass before [Figure 3a) and after the removal of NI (II) [Figure 3b). The EDS showed a high content of oxygen, carbon, and nitrogen, which is consistent with the elemental analysis presented in Table 2, characteristic of biomaterials of lignocellulosic origin. After the adsorption process, the appearance of Ni (II) in the spectrum is observed, confirming the adsorption on the surface of the adsorbent and the disappearance of chlorine, sodium, and potassium, which would be the active centres to which the ion adhered[37, 39].
3.2 Adsorption tests
After carrying out the Ni (II) adsorption tests using the residual plantain biomass, the effect of the amount of biomass, the temperature, and the initial concentration of the metal was evaluated by calculating the adsorption capacity of the metal ion. In Table 5, the results in terms of adsorption capacity (Equation (1)] and removal efficiency (Equation (2)] are shown at the set conditions experimental design.
It was established that it is at 55 °C, 368.2 mg/L and 0.6775 g of biomaterial, where a higher percentage of removal of 87.53 % is obtained.
T: Temperature, Ci: Initial concentration, Cf; final concentration, and qt: adsorption capacity.
The temperature effect on the adsorption process is useful in the actual application of the adsorption system, as the effluents are discharged at different temperatures[39]. The effect of temperature on the adsorption capacity is shown in Figure 4.
It is observed that a temperature increase from 40 to 70 °C favours the adsorption of Ni (II) ions, suggesting that the system is endothermic and needs energy supply to increase the electrostatic interaction forces between the active centres of the material and the ions[40]. The favourable effect of temperature on Ni (II) adsorption may be due to the increased ionization of the lignocellulosic components[41]. They act as adsorption sites, thus promoting the increased rate of diffusion of Ni (II) through the outer boundary layer into the pores[42, 43].
The effect of the dose on the adsorption capacity is shown in Figure 5.
A decrease in the adsorption capacity of the metal was observed as the amount of adsorbent increases, with the maximum capacity and percentage of adsorption being reached at an adsorbent dose of 0.6775 g. At a high dose of adsorbent, reduction of adsorption capacity may be due to the binding of ions to the active sites and establishment of the balance between ions bound to sorbent and ions in the solution. Therefore, there may be a competition of solute ions for limited available binding sites, electrostatic interactions, overlapping or aggregation of adsorption sites, low surface area, interference between binding sites, and contact of ions below higher adsorbent densities[41].
Figure 6 shows the effect of the initial concentration of Ni (II) on the adsorption capacity of the metal on the bio-sorbent. An increase in adsorption capacity is observed as the concentration of contaminant in solution increases. This event could be due to the higher ionization potential of the metal at higher concentrations and the increase in the concentration gradient as the driving force promoting mass transfer, thus decreasing the resistance to mass transfer between the bio-sorbent and the bio-sorbent medium[43].
3.3 Statistical analysis
Figure 7 shows the standardized Pareto diagram for Ni (II) adsorption capacity, which shows in a standardized way that the most positively influential variable is the initial concentration and the initial concentration-initial concentration interaction. At the same time, the adsorbent dose has a significant negative influence on the process[44].
Likewise, the statistical significance of the second-order equation revealed that the regression is statistically significant, and based on the results of ANOVA, the regression model of Equation (6), reports a high value of R2 of 99.39%, which implies a high correlation between observed and predicted values[45, 46].
Where qt (m/g) is the adsorption capacity of the adsorbent, C (mg/L) is the initial concentration of the contaminant, T (ºC) the temperature and D (g), and the adsorbent dose. The positive and negative signs before the terms indicate the synergistic and antagonistic effect of the respective variables[47, 48].
To evaluate the individual interaction and the quadratic effects of the variables that influence the Ni (II) removal capacity on the plantain residues, an analysis of variance (ANOVA) was performed. Table 6 shows the results obtained from the analysis of variance; this table shows the partitions of the adsorption variability for each variable evaluated with a p-value < 0.05. It is observed that the factors with greater relevance in the design are adsorbent dose (g), initial concentration (mg/L), and initial concentration-initial concentration interactions [Figure 7]. The ANOVA validated the importance and adequacy of the model obtained by optimizing the response.
Regarding the percentage of Ni (II) removal, from Table 6, it can be seen that the F-value of the model is 32.13 and the P-value of 0.042, which means that the model is statistically significant (Equation (6). The model's significant terms were C, D, CC, and DD, with only T as a non-significant factor for the response. From the statistical results obtained, it can be seen that the model was adequate to predict the removal of Ni (II) within the range of the variables studied. A correlation coefficient between the real data and those predicted by the model of 99.26% was obtained, portraying that the developed models successfully captured the relationship between the variables of the adsorption process evaluated and the response [Figure 8].
It is observed that the most influential variable on the process is the initial concentration of the contaminant. This variable is a driving force for the mass transfer of the metal ion from the solution to the surface of the adsorbent, whereby as the concentration in the solution increases, the process is more efficient. The adsorption capacity will be higher because this is the result of the increasing concentration gradient that acts as a driving force to overcome the resistance to mass transfer of the metal ions between the adsorbate and the adsorbing species[48]. Also, the variation in the percentage of elimination and adsorption capacity of Ni (II) on residual biomass of plantain starch does not have a proportional variation profile, considering that this variable is one of the most significantly influential in the process.
3.4 Adsorption kinetics
The kinetic study was carried out to analyse the behaviour of the adsorption process in time. In Figure 9, the kinetics of Ni (II) adsorption and the fit to the pseudo-first-order, pseudo-second-order, Elovich, and intraparticle diffusion adsorption models are shown. It is observed that the rate of adsorption is fast adsorption in the first 60 min, reaching a maximum metal adsorption capacity of 19.1 mg/g, which is high compared to 9.8 mg/g when using tea residues[49] and 9.13 mg/g on pisum[11]. This rapid rate of biosorption at the beginning of the process can be attributed to the high availability of sorption sites during this period, which demonstrates the effectiveness of residual plantain starch biomass as a bio-sorbent in the short contact time with Ni (II)[4].
The fit parameters of the models used were found by non-linear fit and are shown in Table 7. It was found that the pseudo-second-order model describes better the adsorption kinetics of Ni (II). Figure 8 suggests that two active sites of the biomass can adsorb the metal ion; from the value of the constant k2, it is shown that the initial sorption rate for Ni (II) is low, thus also achieving a low adsorption efficiency. Likewise, Elovich's model also presented a good fit according to the correlation coefficient of 0.9299 and the parameter α of 6806.2044 mg/g min. According to this, it can be concluded that Ni (II) adsorption takes place inside the pores of the particles of the bio-adsorbent materials considering the heterogeneity of the active sites of the adsorbent found in the FTIR spectrum of Figure 1, so they exhibit different activation energies along the adsorption process[29, 50].
3.5 Adsorption isotherms
In order to describe the equilibrium of the separated solute between the solid and liquid phases, the evaluation of the data obtained in the Ni (II) adsorption process on the residual biomass of plantain starch was carried out, using models such as Langmuir and Freundlich. The adjustment of the equilibrium data by non-linear regression is shown in Figure 10, and the adjustment parameters are shown in Table 8.
It was found that the two models evaluated to fit the Ni (II) adsorption data, which indicates that both mechanisms have an incidence. However, physical forces prevail over them, and the formation of a monolayer limits the process; no desorption reaction is considered. The number of species adsorbed does not exceed the number of active sites[51].
The value of Freundlich's constant n is in the range 1-10, so the electrostatic interactions and links formed between the ions and the active centres are strong, indicating that the process is favourable with high adsorption intensity[44, 52].
3.5 Thermodynamic parameters
The thermodynamic study of adsorption allows establishing the mechanisms of the process in terms of reversibility and spontaneity, and its exothermic or endothermic nature, by calculating the change in Gibbs' standard free energy (ΔG°), standard enthalpy (ΔH°) and standard entropy (ΔS°). The thermodynamic parameters mentioned above were calculated by applying Van't Hoff's graphical method and Equations (3) to (5); the results are shown in Table 9.
The positive value of ΔH suggests that the process is endothermic and needs energy supply to be able to happen, which confirms the results shown in Figure 4[53]. The negative values of ΔS suggest that the biomass has an affinity for the ion and strong bonds are formed on the surface, making the process irreversible; these results confirm what is shown by the FTIR and SEM-EDS analysis, as well as the fit of the equilibrium data to Freundlich's model[54]. The positive values of Gibbs' free energy indicate that the adsorption process is not spontaneous, favourable and that reactions are not feasible at moderate temperatures[52, 55].
4. Conclusions
The characterization of the residual plantain biomass shows the presence of hydroxyl, carbonyl, and carboxyl groups belonging to cellulose and lignin, which are attributed to participating in the adsorption process. After the adsorption process, the decrease of the adsorption bands was observed, so the metal removal was proved. The SEM and EDS analysis show that the process occurs due to electrostatic interactions between Ni (II) and the active centres inside the pores of the biomaterial. It was found that the best conditions for the removal of nickel were 368 mg/L, 0.6775 g of adsorbent, and 55 ºC, with a yield of 87% and a maximum adsorption capacity of 47.57mg/g. The adsorption kinetics were adjusted to the pseudo-second-order model and the isotherm to the Langmuir and Freundlich models, which suggests that the process is controlled by chemical reaction and occurs in multilayers. The adsorbent under study is presented as a viable and sustainable alternative for the removal of Ni (II) present in aqueous solutions.