1. Introduction
The soil is defined as a complex and dynamic system that constitutes a fundamental component of the environment. It aids in the generation of several ecosystem functions and services, acting as the foundation for biodiversity habitat, regulation of the hydrological and nutrient cycle, food production, among others. Soils are formed from the decomposition of rocks and organic matter over the years, caused by factors such as weather, climate, and microorganisms [1]. In soils, heavy metals can originate from natural sources such as parent material, pedogenesis, and weathering conditions that maintain an equilibrium in the concentration of these elements, contributing to the biological cycles. On the other hand, the anthropogenic origin of heavy metals in soils can be traced to industrial and agricultural activities (use of fertilizers and/or pesticides), sewage and debris, or mining operations [2].
Soil contamination, caused by the presence of heavy metals, such as lead (Pb), copper (Cu), manganese (Mn), zinc (Zn), cadmium (Cd), chromium (Cr), and iron (Fe), can generate negative impacts on hydric resources. For example, when these elements are transported at groundwater levels in soil solution, they affect the quality of groundwater [3] and human health, leading to an impact on the soil, which could, in turn, trigger a process of contamination in the entire ecosystem. To face this problem, in the industrial city of Shaanxi-China, a potential ecological risk index (RI) was developed to assess ecosystem risks caused by high levels of heavy metals (Pb, Cu, Ni and Zn), in order to provide inputs for the implementation of regulatory and risk management measures [4]. Similarly, in Indian soils, non-sustainable intensive agriculture, industry, and urban development have significantly contributed to the presence of metals that can present a great risk to the ecosystems when evaluated with the RI method [5].
In Colombia, the problem of metal contamination has been studied for the last forty years, and its impact has been observed in every hydrographic basin of the country, mainly due to the high persistence of these metals in the environment, their toxicity, biogeochemical recycling, and ecological risk. In some areas of the Colombian Caribbean coast, where gold-mining activities have been traditionally carried out, higher concentrations of metals, mainly mercury, are reported in soils, sediments and, through biomagnification mechanisms, in fish and humans [6].
The environmental effects of heavy metals in agricultural soils are related to their non-biodegradability and their interaction with different soil properties that determine their accumulation, mobility and bioavailability. This allows for their inadvertent accumulation in the soil profile, reaching toxic concentrations, which can later interact with other components of the ecosystem [7]. Another study analyzed their impact on soils and the Paramo ecosystem in the Colombian Andean region, demonstrating that soils contribute to the mitigation of metal pollution in rivers, and emphasizing the need of taking into account heavy metal concentrations in Colombian environmental quality legislation [8].
The Guasca municipality engages in agricultural and cattle raising activities, which produce intermediate and capital goods near Bogota, Colombia’s capital. This region is characterized by agroecosystems that have historically changed land use, oscillating between agriculture, livestock, and milk production, which have generated pressure regarding the quality of resources such as water and soil [9]. In 2015, the Borderless Engineers Project study group developed a quality analysis of water sources, reporting that its low quality was caused by the presence of total coliforms, low levels of pH, as well as high concentrations of Pb close to the ceiling limit provided in National Resolution 2115 of 2017. Similarly, other studies measured Pb concentrations in soils used for grazing activities, in order to examine a possible relation between Pb concentrations in the soil and concentrations of the same metal in the water. These findings determined that the groundwater is polluted by heavy metals, which could lead to health problems in the municipality of Guasca.
This research aims to determine the Potential Ecological Risk Index in a rural grazing area located in Colombia used for cattle raising, by determining metal concentrations, evaluating soil quality, and analyzing its spatio-temporal behavior.
2. Materials and methods
The study area is located at La Trinidad village in the municipality of Guasca, Cundinamarca: a total area of 2,292 m2 [Figure 1]. Based on the dimensions selected and the underground water reservoir located at the center of the north side of the study area, a slope of 9.47% was measured, which recharges from rainwater, a channel of approximately 30 cm. wide and 20 m. long. This channel diagonally crosses the study area ( Figure 2].
This area is used for grazing dairy cattle. Likewise, its underground water source has been demarcated for its protection, as it supplies the families living in the area. Historically, and verified through field visits, the area was used for potato cultivation, which used organic fertilizers, chemical fertilizers, and pesticides in its production.
2.1 Sample collection
The area was divided into seven blocks and each block into four equal parts, in order to cover areas adjacent to the water source [Figure 1]. Two composite samplings were carried out following the USDA methodology (2001), during the dry climatic season in March-2017 and during the rainy season in June-2017, according to rainfall records [10].
Soil samples were collected to analyze their physicochemical properties, including P, K, percentage of organic carbon, Cation Exchange Capacity (CEC), Nitrogen in Nitrate state (N-NO3), bulk density and pH. Additionally, 100 gr of soil sample were collected to determine heavy metal concentrations (i.e. Pb, Cu, Mn and Zn). For this specific study, Cr and Cd were not taken into account. Duplicated subsamples of soil were processed by acid digestion (HNO3) treatment, using protocols for metal determination in environmental samples (U.S. Environmental Protection Agency [EPA], 1991).
Furthermore, two certified labs conducted the analysis of these samples, in order to obtain the necessary information for this study. In relation to physicochemical properties such as P, K, percentage of organic carbon, Cation Exchange Capacity (CEC), Nitrogen in Nitrate state (N-NO3), and pH, the results were provided by Dr. Calderón Labs in the city of Bogota, a certified Lab, through a complete soil analysis. Additionally, the analysis for heavy metals in soil (Pb, Cu, Mn, Fe and Zn) was performed by the Chemical and Biology Lab from the ECCI University, also located in the city of Bogota. This analysis was made through the procedure of atomic absorption spectrophotometry (AAS) with direct air-acetylene flame, Standard Method 3111B. The instrument’s limits of detection (LOD) was 0.97 mg/g, and the limit of quantification of 0.153 mg/g for Pb, Cu, Mn, and Zn.
2.2 Soil quality
On the one hand, the chemical properties analyzed to establish the quality of the soil were Phosphorus (P), Potassium (K), percentage of organic carbon (% OC), cation exchange capacity (CEC), Nitrogen (N-NO3) and pH. On the other hand, regarding physical properties, texture and bulk density were analyzed. Furthermore, soil quality was assessed using Equation (1) [11]:
The scale categorizes the soil quality from one to ten with a linear or nonlinear valuation. The lower limit of the range is the “least favorable” value, while the upper limit is the “most favorable” value [12]. For this study, classification ranges of soil quality were considered intermediate if there were values between 3.1-5, acceptable with values from 5.1 to 8, and good with values from 8.1 to 10. This methodology was adapted from Arrieche, R., a study performed in 2012 [11]. Additionally, variables determined with Principal Components Analysis (PCA) on a temporal scale were analyzed, in order to identify relations between them.
2.3 Potential Ecological Risk Index (RI)
To estimate and quantify the risk that an adverse effect can generate on the integrity of ecosystems, the Potential Ecological Risk Index was determined using formulas 2-4 [13]. Equation (2) is the contamination factor of a single element. Equation (3) represents the potential ecological risk factor of a single element, and Equation (4) is the comprehensive potential ecological risk index.
Where
is the metal concentration extracted from the samples, and
is a reference concentration value gathered from studies carried out in the Bogotá Savannah. In this study, the reference values for each metal were: 10 mg/kg for Pb, 60 mg/kg for Zn [14], 198 mg/kg for Mn and 16 mg/kg for Cu [15]. On the other hand,
is the biological toxicity factor of a single element; the value used for Pb was five [16], for Mn it was two, for Cu it was five, and for Zn it was one [17]. The ecological risk factor
and potential ecological risk index (RI) outcomes were analyzed to determine the risk degree according to the categorization of Table 1. Iron (Fe) was not taken into account for this calculation because the secondary information, and initial methodology presented by Hakanson (1980), do not include a toxicity factor for this metal.
2.4 Kriging interpolation methodology
Considering the lead (Pb) concentrations found in the study area, their outcomes in relation to the ecological risk factor
, and influenced by the potential ecological risk index (RI), the Kriging Bayesian interpolation method (Equation (5)][18] was conducted, using ArcGis 10.3®. This technique was implemented to identify the behavior of Pb concentrations in both sampling seasons. The outcomes related to Pb were analyzed temporarily, digitizing the area and considering underground water reservoirs, inclination, distribution of blocks and their respective sampling points (28 in total). Additionally, a digital elevation model (DEM) was implemented, extracted from the NASA database with a resolution of 1.7x1.7 km, which allowed for identifying contour lines and the determination of their influence on the pollutant displacement.
S i is the value measured at a specific location, λi the unknown weighting for the value measured at location i. is the location of the estimation. Lastly, N is the number of measured values.
3. Results
3.1 Physicochemical indicators and soil quality
Every physicochemical result in the soil analysis displayed in Table 2 show that during the rainy season, the concentration values of K, P and N-NO3 increased (29.1, 3,105 and 34,800 cmol/kg, respectively) compared to values during the dry season (1.2, 31.0 and 40.0 cmol/kg, respectively). Similarly, the percentage of OC decreased in the rainy season r (4.9%-6.1%), in comparison to the dry season (11.5%-12.9%). Furthermore, the pH values decreased from a maximum range of 5.5 in the dry season to 4.6 during the rainy season. CEC showed an opposite behavior compared to that of OC and pH: it increased its values during the rainy season. Thus, it became a determining factor for the adsorption of contaminant elements in the soil (e.g., waste from agrochemicals and heavy metals) [11]. The change in values of CEC may be related to OC concentration, due to it being one of the composites that provides such features to soil aggregates.
The physical properties of the soil, texture, and density remained stable at both sampling seasons since no type of mechanization was carried out in the study area. Soil texture is silty, i.e., it is more impermeable than sand or clay; hence, it can generate greater retention of elements in soil aggregates and their water properties [19]. The apparent density results are low, as it has an OC-rich soil, which indicates that the porosity of the soil is adequate.
On the other hand, the behavior of physical and chemical properties in the sampling area showed how P and N-NO3 concentrations increased during the rainy season, specifically in the block five area, which is located to the left side of the underground water well and has less inclination. Compared to the higher concentrations of both elements in the dry season, which were distributed in the areas blocks three and five [Figure 2], they were located at the north end of the study area and of less inclination than the others. In addition, K displaced its highest concentrations from blocks one and five during the dry season, the first one located at a more significant distance from the well and with a greater elevation, to blocks two and three during the rainy season. The aforementioned was caused by the slope present in the field with a direction towards blocks two and three, which, with the interference of the runoff, can mobilize this element. On the other hand, during the dry season, K is accumulated in an elevated ground of the area. Similarly, CEC may be influenced by runoff processes, registering greater ranges during the rainy season in blocks three and five.
Table 1 Standards for classification of ecological risk factor (Eri) and the potential ecological risk index (RI)

There is also an increase in the concentrations of metals and chemical elements during the same sampling season. Thus, it is likely that these spatial behaviors are influenced both by the inclination of the ground and by the runoff and soil washing processes.
Average soil quality per sampling block indicates a good quality both for the dry season (5.9-6.5) and for the rainy season (6.4-6.9) displayed in Table 2. It was observed that soil quality increases and is maintained in closer ranges in each block during the rainy season. This behavior may be influenced mainly by the CO and pH values, as well as soil nutrients, such as K and P. When observing the results, it is evident that block one maintains a higher range and appreciation of quality during both sampling seasons compared to other blocks.
3.2 Metals in soil
The outcomes showed an increase in concentrations of Cu, Zn, Mn and Fe during the rainy season. From these results, we can infer that soil availability is influenced by characteristics such as pH, OC and CEC. On the other hand, Pb concentrations significantly decreased in each block with the exception of block three (790 mg/kg), located at the north end of the field and that had less inclination. This phenomenon is probably related to runoff, soil washing, and land inclination, as shown in Table 3.
When conducting a PCA exploratory analysis in relation to soil physicochemical properties and metal concentrations, a cumulative variability was obtained in factors one and two, corresponding to 61.51% for the dry season [Figure 3], and 68.33% for the rainy season [Figure 4]. These percentages can statistically explain the variation of metals in relation to their properties.
During the dry season, PCA shows a correlation between metals like Pb, Zn and Fe, and chemical properties like P and CEC. On the other hand, there is a low correlation between other chemical properties such as OC, pH and nutrients such as N-NO3 and K [Figure 3]. During the rainy season, the CEC is the property that mostly relates to metal concentrations, including Cu. Furthermore, the soil has K as a nutrient related to these elements [Figure 4].
3. 3 Potential Ecological Risk Index
From the reference concentration values and biological toxicity factor for each metal, the metal contamination factor, potential ecological risk factor, and potential ecological risk index were determined based on the concentration average [Table 4]. The results indicate that Pb is the key element that contributes to the contamination risk, with an
of 75.07 for the dry season and a value of 122 for the rainy season [Table 4], showing high levels of contamination in the two sampling seasons and a higher incidence during the rainy season, mainly due to its high toxicity. In contrast, the essential metals in the soil (i.e., Mn, Zn and Cu) have a low degree of contamination since they can only generate risk in extreme cases where their concentrations exceed the permissible levels. The aforementioned explains why the risk factors do not exceed 10.33 in relation to Mn for the rainy season. Furthermore, the ecological factor of the metals for each season impacted the behavior of the RI, which decreased during the dry season, in comparison to the rainy season [Table 4].
However, Pb is the main element closely linked to the RI, as identified by the values determined by the index, generated by the overall increase of its concentrations in the soil under study, as well as concentrations of the other metals that were present.
3.4 Implementation of the Kriging method
The Kriging method allowed for the interpolation of the distribution of Pb concentrations obtained for both sampling seasons [Figure 5]. During the dry season, we observed that the southern side of the field had the highest Pb concentration because the degree of elevation in the area is greater, specifically, in blocks one and six [Figure 5]. However, during the rainy season, the highest range concentrations of Pb move northward, where there is a steep inclination of the area, between blocks two, three, and five, which had the greatest contact with the underground water well [Figure 6].
4. Discussion
Several outcomes of this research showed that the behavior of soil nutrients is possibly affected by processes such as washing and the contribution of elements from surrounding crops which, alongside runoff and the inclination level, increase their concentrations in the soil surface layer, mobilized throughout the different seasons of the year (i.e., registering higher concentrations during the rainy season). Similarly, the sporadic fertilization generated by the excrement of cattle that usually graze the study area, according to[15], may explain the increase in K concentrations during the rainy season. In addition, it is possible to observe that runoff contributes with fertilization chemicals from surrounding crops during the rainy season. Likewise, this chemical element boosted the soil quality as it represents a greater nutritional contribution to soil and vegetable matter when the rainfall increases in the area.
Table 4 Values to determine the Potential Ecological Risk Index and its respective results
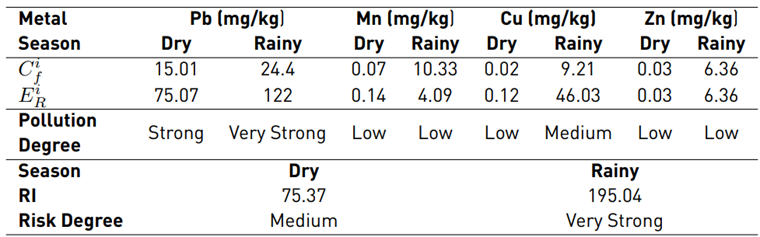
Note: Ci f is the pollution factor of the element and E i R is the potential ecological risk factor of a single element.
P availability, a fundamental element in the nutrition of vegetal matter, which allows for an increase in the maturation and quality of crops and which, in turn, does not have much mobility in comparison to nitrogen (N) [20], is easily affected by soil properties such as moisture, texture, organic matter and pH. In particular, the amount of available P is greater when the soil texture is fine. Given that the texture present in the study area is silty, we can infer that concentrations of this nutrient increase due to the retention generated in available spaces in the soil [20]. This is because silty-textured soils significantly impact water retention in soils, while retaining nutrition elements such as P, and contaminants as heavy metals. However, this could also be closely related to the pH levels found in the study area, since these acid values fix the element in a greater proportion to those generated at higher alkalinity levels [15]. Variations in soil concentrations of N-NO3 may be caused by the influence of temperature and humidity, which explains why concentrations during the rainy season are higher than during the dry season. This is possibly due to an increase in soil moisture, influenced by precipitation, which manages to raise levels of N available in the soil [21].
The variation in the OC percentage could be directly related to values obtained from pH in the soil because concentrations of OC increase in the same proportion as pH values. Soil organic matter tends to increase when the pH of the soil is acidic and to decrease in alkaline soils. Considering the pH and OC results, the importance of CEC is represented in the control it exerts on the availability of nutrients such as K, Mg, and Ca, as it is able to form structures and stability of aggregates, and retain contaminant elements in soil [22]. Finally, from its chemical properties, the soil is categorized as very acidic during dry and rainy seasons, which generally relates to leached soils and areas with high levels of precipitation [23], as those present in the region. Thus, it is evident that the current use of the soil in this study area is appropriate (grazing and conservation). However, its conditions are not suitable for implementing agricultural activities.
As already discussed, pH is a parameter that directly affects the availability of nutrients in the soil, since hydrogens and aluminum abound in a complex change of soil, which prevents other essential elements such as Ca, Mg, Na or K from remaining in it, as they are easily eliminated by runoff or assimilated by vegetable matter [15]. In the case study, we can infer that pH conditions for two sampling seasons are a limitation with respect to the growth and development of crops, as they hinder the retention of the aforementioned nutrients.
As for soil physical properties, first, we can infer that the silty texture does not greatly contribute to its quality since, according to [7], loamy textures (silt and sand) favor vegetable material production, and although it is a soil used for grazing with a water source limitation, its mechanization makes its structure more compact than that of soils with a productive use. In summary, it is possible to infer that in each sampling season, soil quality is mainly influenced by the values of pH, OC, and soil nutritional elements (K and P), the latter being the main parameters that can improve its quality, in the case of a possible productive use different than grazing.
By seasonally analyzing concentrations of each metal and factors that directly affected them, a PCA was developed where a decrease in Zn concentrations during the dry season was observed, influenced by CEC and by the high presence of P, due to soil porosity [20]. However, there was a significant increase in these Zn concentrations during the rainy season due to the high organic matter mineralization that remains throughout the season, which allows for the formation of mobile organic complexes absorbable by plants [24]. Copper behavior could be affected by factors such as pH with values higher than 7, which can interfere with its availability. In contrast, its availability increases with values below six. Likewise, this phenomenon can also be influenced by the micronutrient N-NO3 and the percentages of OC, since a highly organic soil can feature a deficiency of metallic micronutrients [20].
Taking into account the previous results, during the dry season, the concentrations of N-NO3 in each block are high due to excess of fertilizers implemented in the surroundings of the area, as well as a higher OC percentage than during the rainy season. Thus, we can deduce that the soil has a Cu deficiency during the dry season. However, during the rainy season, due to a decrease in the same nutritional element of the soil, the percentages of OC and pH, the availability of metals increases.
When it comes to iron, its soil availability is mainly affected by pH level of the soils. For this case study, the amount of this metal is limited by the type of silty texture shown in the laboratory analyses. This is because its moisture saturation is high, while its aeration is low and thus, the accumulation of CO2 increases, decreasing its availability [25]. Finally, and taking into account that the behavior of Pb was completely different from other metals, i.e., its concentrations decreased during the rainy season, we can attribute its mobility to the influence exerted by physical adsorption, which allows for the conservation of its chemical nature. It is worth noting that its dynamics in soil may also be influenced by OC, CEC and the low pH range[26].
Once the concentrations of soil metals are determined for both seasons, we can conclude that Pb has the highest degree of incidence on results of Potential Ecological Risk Index (RI), not only due to its high concentrations, but also to the impact that such element can have on the ecosystem stability, e.g., cause harm in relation to chlorophyll and photosynthetic processes, negatively impacting the overall growth of the plants [27]. Likewise, high concentrations of this element can also affect human health, including the central nervous system and the gastrointestinal tract [28] Therefore, RI shows how the influence of a single metal with a high ecological risk factor can involve a large degree of pollution risk for the human population. Considering that Pb was the metal that entails the greatest risk for RI, it was the only metal to which the Kriging spatial distribution method was applied, reaffirming the previously stated hypothesis, and confirming that the slope of the land has an impact on the distribution of this metal at different sampling points and seasons. Similarly, and taking into account the results of the analyses, an increase in the precipitation level and, therefore, in the runoff, causes Pb concentrations accumulated in the upper part of the study area to move to the bottom, that is, to blocks three, five and the northern part of block seven. It is worth highlighting that the channel that crosses in diagonally facilitates Pb dragging towards groundwater well, which will later be used for human consumption. Considering the aforementioned results, and the fact that the soil is used for grazing dairy cattle and the cultivation of vegetables and potatoes, we identified that this risk of heavy metal pollution, especially Pb, could create a direct risk for the population supplied by this soil resource (food and water bodies, surface or underground).
5. Conclusions
The analyses of physical and chemical properties in the study area show that the soil has an acceptable environmental quality (range 5.8 to 6.9) for the current use of grazing and the demarcation of the conservation area of the groundwater well. However, if the use of the soil changes, e.g., to agricultural use, a soil treatment process (mechanization, addition of amendments, bio-recovery, among others) must be conducted, in order to improve its quality and the performance of its resources. On the other hand, Guasca belongs to the Guavio region, where significant mining activity with an impact on the environment occurs, which causes contamination of the bodies of water and soil and makes this region susceptible to permanent risk monitoring concerning metals in this study area [29].
From the analysis of metal concentrations on a spatio-temporal scale, we can observe that, with higher levels of precipitation, these concentrations increase in the resource, influenced both by the change in the soil property values and by the runoff phenomenon, driven by the inclination of the area. This last factor not only provides chemical elements (nutrients and metals) from nearby crops, but also manages to transfer their concentrations to blocks near the well, which implies that, during seasons with high rainfall, the risk of pollution of water resources for human consumption, caused by heavy metals presence, increases.
As a result of the aforementioned analyses, this grazing soil showed a RI, due to metal concentrations, with a medium degree of risk (75.37) during the dry season, and very high (195.04) during the rainy season, which constitutes a risk alarm for the soil under study, the surrounding ecosystem and the population supplied by the crops and groundwater that emerges from the area. Such degree of risk was mainly influenced by Pb concentrations, which presented a potential risk factor of 75.07 for the dry season, and 122 for the rainy season. This metal is possibly introduced to the soil not only by human development activities carried out in the area, but also by contributions by parent material upon reaching surface layers of the soil, which, through natural weathering processes, can increase the release of this element and thus, its concentrations.
From the results of this research, at the time of conducting this study, we can conclude that the quality status of the soil does not amount for an alarm for the ecosystem, the surrounding population, or for the current use (grazing). On the contrary, the risk is directly related to heavy metal concentrations and their degree of incidence on the Ecological Risk Index, which may negatively impact the ecosystem or the health of the population, if the soil starts to be used for food extraction, which, through biomagnification, would increase the amount of direct contact and exposure to contaminants present in the soil.