1. Introduction
Municipal wastewater released into natural water sources currently represents a critical problem worldwide. This is primarily because high volumes of water are being polluted every day, leading to a decrease in the availability of this natural resource for human consumption, agricultural use, and domestic activities [1,2]. As a result, the current study attempted to search for new ways to treat and recover wastewater.
In the last decade, Advanced Oxidation Processes (AOPs) have been widely studied to achieve this objective [3-5]. The effectiveness of these technologies is based on the generation of strong and non-selective radicals, leading to the elimination of a wide variety of contaminants and enteropathogenic bacteria present in wastewater [6-9]. The most common AOPs used for the treatment of wastewater are solar photo-Fenton, solar UV-Vis/H2O2, UV/O3, and solar photocatalysis based on semiconductors such as ZnO or TiO2.
In general, heterogeneous photocatalysis based on semiconductors leads to a photochemical activation process that can be applied to a wide variety of reactions such as total oxidations, hydrogen transfer, dehydrogenation, metal deposition, water detoxification, and the removal of gaseous pollutants, among others[10]. The activation of the semiconductor enables the promotion of an electron from the valence band to the conduction band. This process simultaneously creates a hole with a positive charge density which is generated in the valence band, as shown in Equation 1.
These photogenerated charges have an important influence on the elimination of pollutants by AOPs. Even though the effectiveness of these treatments has been proven in different environmental recovery processes, heterogeneous photocatalysis presents a series of disadvantages. For instance: in this process, the 𝑒− + ℎ+ pair can be recombined in nanoseconds, thus leading to a significant decrease in the overall treatment effectiveness. In order to avoid this recombination, different methodologies such as the addition of fluorine [11] and the photodeposition of metal nanoparticles including Au, Ag, and Pt have been employed [4,12].
It has also been reported that the heterojunction formation between mixed oxides such as ZnO, TiO2, and Nb2O5 is an effective way to achieve a better separation of photogenerated charges. This improves the photocatalytic response of these materials [13], and therefore, the effectiveness of the composed materials in wastewater treatment by photocatalytic processes [13-15].
It is also widely known that Nb2O5 has basic acid properties, which can effectively contribute to the degradation of different organic pollutants like dyes, glycerol, cyanide, bacteria, and drugs 14,16-18. This effect can be associated with better adsorption of these pollutants on the surface of niobia, which can present positive and or negative active sites available for its adsorption, thus leading to a more effective way of breaking the bonds in the pollutant molecule.
From the perspective of the current study, it is possible to observe that a number of scientific studies have been devoted to the evaluation of the efficiency of mixed oxides in the degradation of organic pollutants; however, most of these studies are focused on the use of lab prepared solutions including model molecules as substrates. Currently, there is an important necessity in the evaluation of treatments as potential answers to solve environmental problems. As a result, it is very important to know the effectiveness of different treatments by using real wastewater.
Taking the above into account, the main objective of this study was to test the effectiveness of heterojunctions formed by ZnO/TiO2 and ZnO/Nb2O5 in the photocatalytic treatment of real wastewater samples. These polluted samples were obtained from textile fiber dyeing activities in handicraft factories and water samples from a polluted river. The photocatalytic performance of these heterojunctions for the elimination of organic pollutants such as dyes and the elimination of bacteria such as E. Coli was evaluated.
2. Materials and methods
2.1 Photocatalysts preparation
The prepared materials corresponding to heterojunctions were formed by coupling pairs of oxides. These materials were prepared following the wet impregnation method reported by Vaiano et al. [14]. The TiO2 (Sigma Aldrich, 99.7%) and Nb2O5 (Sigma Aldrich, 99.9%) with commercial ZnO (Sigma Aldrich, 99.0%) were mixed in a defined 1:1 ratio. This mixture was subjected to rotatory evaporation in the presence of an isopropanol solution (0.3M) for 1 hour at 82 ºC. Finally, the materials were recovered by centrifugation and characterized by different instrumental analysis techniques.
2.2 Photocatalyst characterization
The materials prepared were characterized by XRD, SBET, and UV-Vis DRS. The experimental conditions of these analyses and the equipment employed are described as follows. The XRD analyses were carried out by using a Siemens D-501 diffractometer, including a nickel filter and cupper Kα radiation. The scanning angle utilized was 2θ from 10° to 80° with passes of 0.05° and a total lecture time of 1 s. The photocatalysts were evaluated by UV-Vis diffuse reflectance spectrophotometry (UV-Vis DRS) by using a Varian Cary 100 spectrophotometer with an integrating sphere and BaSO4 as a reference. These measurements were evaluated in a wavelength range between 200 and 800 nm. The band gap values were calculated by using the Kubelka-Munk F(R∞) function, which is proportional to the absorption of radiation. These calculations utilized the graphical function (F(R∞) × hν)1/2 Vs. hν.
2.3 Wastewater sampling
The water samples to be treated by photocatalysis were collected from two different sources and are described as follows: (i) In Tunja, Colombia, from a highly polluted river due to municipal effluents which was sampled at the coordinates 5° 33' 13.5" N, 73° 21' 02.6" W; and (ii) at a textile factory located in Nobsa, Colombia, at the coordinates 5°46′11″ N, 72°56′24″ W. The samples were collected in batches of 30 L, transported and stored using the protocols described in the Standard Methods for Examination of Water and Wastewater (SMEWW) [19]. The samples were tested on the same day they were collected.
The water samples were characterized before and after photocatalytic treatment. Hardness, alkalinity, and chlorine concentration were determined according to the Standard Methods[19], as reported in Table 1. TOC analyzes were performed using an AJ multi N/C series TOC analyzer. The Total Coliforms and E. Coli colonies were determined using the standard plate count method with dilutions in a selective Chromocult (26.5 g/L Merck Agar Chromocult) medium. Colonies were counted after 24 hours of incubation at 37º C. The bacteria concentration in the wastewater samples analyzed is reported as CFU (Colony Forming Units)/ 100 mL. Bacterial regrowth studies were conducted and monitored every 24 hours for three days.
The pH values were measured by using an SI analytics Lab 850 pH meter. Other physicochemical parameters such as alkalinity, hardness, and chloride content were calculated by using the 2320 B-1995 APHA, 2340: C APHA, and 4500 Cl-B-1995 APHA methods, respectively[19]. All of the physicochemical analyzes were carried out twice.
The evaluation of the dye concentrations in the handicraft factories’ wastewater during the photocatalytic tests was analyzed by UV-Vis spectrophotometry using a Thermo Scientific Evolution 300 spectrophotometer.
2.4 Wastewater photocatalytic treatment
Photocatalytic tests were carried out in a batch reactor using a 200 mL sample of wastewater, 1 g/L of photocatalysts, an oxygen flow of (50 L/h), and a total treatment time of 240 minutes under continuous magnetic stirring. As a light source to induce the photocatalytic reaction, an Osram Ultravitalux lamp (300W) with radiation similar to sunlight and the main emission at 365 nm was used. In order to promote the adsorption-desorption equilibrium in the suspension (water sample - photocatalyst), some procedures were carried out. Thus, before switching on the light, it was maintained under stirring and air flow, in the dark, for 10 minutes.
The main conditions employed for the heterogeneous photocatalysis processes are enlisted as follows: (i) All reactions were carried out at room temperature (20 + 1 ºC), (ii) under atmospheric pressure, (iii) the photocatalysis dose was 1 g/L and (iv) total reaction time of 4 hours.
Finally, in order to obtain confident experiments, all photocatalytic experiments were carried out twice.
3. Results and discussion
3.1 Characterization results
The XRD spectra for the semiconductors analyzed in this study are shown in Figure 1. As can be seen, the diffraction pattern of ZnO indicates the wurtzite structure for this semiconductor, with an average crystal size of around 25 nm [9,18]. The main signal for TiO2 is observed around 2θ = 25.25°, which indicates the presence of only the Anatase crystalline phase for this material [4,18]. In the case of Nb2O5, the main signal can be observed around 2θ = 28.58° due to the plane (100), corresponding to the crystalline Nb2O5 in the hexagonal phase [13,18].
The diffraction patterns of mixed oxides show three maindiffraction signals located at 2θ = 31.7°, 34.5°, and 36.2°, corresponding to planes (100), (002), and (101) of ZnO in the Wurtzite crystalline phase [17]. In the ZnO/TiO2 material, a diffraction signal around 2θ = 25.25° can be observed, it is associated to the main of the Anatase crystalline phase of TiO2 [20,21]. In the ZnO/Nb2O5 diffraction pattern, a band around 2θ = 28°, characteristic of the pure hexagonal phase Nb2O5 [14] is seen. These results suggest that the synthesis method used for the preparation of mixed oxides did not modify the crystallinity of the pristine semiconductors.
The TiO2 photocatalyst presents a low surface area (SBET), with a value of 11 m2/g when compared with ZnO (30.20 m2/g). On the other hand, the UV-Vis DRS spectra of TiO2 and ZnO are presented in Figure 2. The absorbance spectra seen by the characteristic absorption edge of the ZnO, TiO2, and Nb2O5 photocatalysts are located around 400 nm. This band is associated with the intrinsic transition in these photocatalysts. The band gap energy calculated for the photocatalysts were 3.25 eV, 3.33 eV, 3.25 eV, 2.95 eV, and 3.02 for ZnO, Nb2O5, TiO2, ZnO/TiO2, and ZnO/ Nb2O5, respectively.
3.2 Photocatalytic wastewater treatment
Polluted river water
The results obtained in eliminating bacteria present in the river under study are summarized in Table 2. Municipal wastewater treatment using ZnO/TiO2 and ZnO/Nb2O5 photocatalysts was moderately effective in the elimination of bacteria. Using the unmodified oxides, 50% effectiveness of the treatment is not exceeded, while with the materials ZnO/TiO2 and ZnO/Nb2O5, and elimination percentages of 55.5% and 64%, respectively, were achieved after 240 reaction minutes which increased over time. This may be because the coupling of two semiconductor materials extends the half-life of the hollow electron pair. The electrons photogenerated in the surface of one of the oxides can jump to the conduction band of the others and involve reduction reactions to produce reactive radicals that react with the pollutant generating its degradation. Additionally, the free holes in the system can react with -OH or water, promoting the production of •OH, which is the main radical responsible for the oxidation of organic molecules until the formation of CO2 and H2O [21,22].
Figure 3 shows the TOC results, which demonstrate the coupling of semiconductors in order to increase the mineralization of the pollutant. When the reaction is carried out by using ZnO, TiO2, and Nb2O5, the TOC elimination percentage was 42.3%, 48.49%, and 57.25%, respectively. However, using ZnO/Nb2O5, an 83% elimination of organic material present in contaminated river water samples was achieved.
Likewise, the figure shows that the coupling of ZnO with TiO2, in this case, does not work as expected because the removal of TOC with this photocatalyst only reached 33%. This is due mainly to the band gap energy of the photocatalysts, especially since ZnO/Nb2O5 showed the lowest value (2.95 eV), which allows the electrons to be photogenerated with lower energy on the surface of the material as well as presenting Lewis acids sites on the surface. Niobia could act as an electron collector, which increases the half-life time of the photogenerated charges and this leads to better photocatalytic performance [22-24].
Table 3 Physicochemical average values of wastewater samples from textile handicrafts factories
Photocatalyst | Reaction time (min) | Hardness (mg/L CaCO3) | Alkalinity (mg/L CaCO3) | Chlorides (mg/L Cl-) | pH |
ZnO | 0 | 330 | 3202.78 | 5672.48 | 8.983 |
240 | 320 | 3122.71 | 5495.21 | 8.674 | |
TiO2 | 0 | 300 | 3202.78 | 5672.48 | 8.983 |
240 | 310 | 3202.78 | 5672.48 | 8.894 | |
Nb2O5 | 0 | 330 | 3202.78 | 5672.48 | 8.983 |
240 | 320 | 3122.71 | 5495.21 | 9.095 | |
ZnO/TiO2 | 0 | 330 | 3202.78 | 5672.48 | 8.983 |
240 | 300 | 3202.78 | 5495.21 | 9.074 | |
ZnO/ Nb2O5 | 0 | 330 | 3202.78 | 5672.48 | 8.983 |
240 | 320 | 2962.57 | 5495.21 | 9.063 |
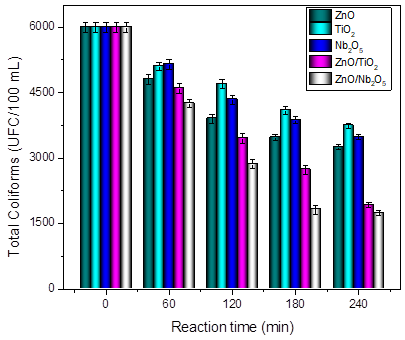
Figure 4 Total coliforms elimination after 240 reaction minutes using the photocatalysts ZnO/TiO2 and ZnO/Nb2O5
Wastewater from textile fiber staining activities in handicrafts factories
Figure 4 shows the results of the bacteria elimination in the wastewater samples from textile handicrafts factories. From the figure, the composite materials showed greater effectiveness for the elimination of bacteria than the unmodified materials (ZnO, Nb2O5, and TiO2), with ZnO/Nb2O5 being the best material for the elimination of microorganisms with an elimination percentage of 71% after 240 reaction minutes. Although it is a relatively high percentage for the elimination of bacteria, the large amount of CFU that persists in the sample after the reaction must be considered since microorganisms proliferate easily under normal conditions of temperature and pressure. This increased performance for the elimination of pathogenic bacteria in these samples is mainly due to the initial population in the sample (6000 CFU / 100 mL) in comparison with the water from the polluted river (37800 CFU / 100 mL). On the other hand, it is important to note that these wastewater samples present higher TOC values and physicochemical parameters such as alkalinity, hardness, and chlorides. These values were greater in comparison with the polluted river water samples, as reported in Table 3. Recent research has mentioned that the high concentration of salt species in the reaction medium decreases the possibility of finding photogenerated holes in the system that will interact with O2 or -OH species in order to produce •OH, which have been identified as being primarily responsible for the elimination of bacteria [4,25-27].
The results obtained in the elimination of dyes on unmodified materials were studied by UV-Vis Spectroscopy; these results are presented in Figure 5. These semiconductors show photocatalytic activity for the elimination of dyes in the wastewater samples; however, the elimination of color is only 33.3%, 81%, and 78% for Nb2O5. ZnO, and TiO2, respectively. This result is mainly due to the lower photogeneration of ROS on the surface of pristine oxides compared to the heterojunctions formed [14,22,24,28]. In the case of Nb2O5, as previously reported, it can have repulsive effects on dyes such as bromophenol blue (BPB), preventing this dye from being degraded [25]. The improved efficiency for the elimination of bacteria can be attributed to the efficiency of the separation of the charge carriers and the quantity of photogenerated ROS. Additionally, the presence of Zn2+ in the prepared materials could enter into the bacterial membrane and promote the proliferation of fibroblasts, thus providing a long-lasting antibacterial property [29], and, finally, the inactivation of the bacteria.
The mixed oxides make it possible to significantly increase the dyes elimination in solution. The elimination percentages obtained were 91% and 90% for ZnO/TiO2 and ZnO/Nb2O5, respectively. Although the percentages of dye removal are significant, it should be noted that the coupling of ZnO with Nb2O5 allowed the drawbacks regarding the affinity of the latter for dyes to be overcome, increasing the photocatalytic material response. These results are due to a decreased energy bandwidth, unchanged crystallinity of the materials, and improved light absorption properties, as evidenced in the characterization of the samples. It has been previously reported that the high efficiency of the materials can be due to the cascade effect of the photogenerated carriers during the separation of the electron-hole pair, the transfer to the other semiconductor, and the migration to the surface of heterojunction formed that permits the oxidation-reduction reactions to be carried out. These characteristics allow the elimination of the organic pollutants present in the wastewater samples to be effective [30].
4. Conclusions
The coupling of ZnO with oxides such as TiO2 and Nb2O5 proved to be a suitable methodology to increase their effectiveness in photocatalytic treatments. This is because these kinds of nanocomposites resulted in an effective separation of photogenerated charges. The ability for photogenerated electrons and holes, which can jump into the conduction or valence band of the other semiconductor, allows them to be more involved in oxidation-reduction reactions to encourage the production of ROS, thereby making photocatalytic treatments more effective.
The mixed oxide that exhibited the best behavior for the elimination of pathogenic microorganisms, degradation of dyes, and photo-reduction of metals was ZnO/Nb2O5. This is due to the physicochemical properties it showed during its characterization: i) Lower bandwidth energy (2.95 eV) with respect to starting materials, ii) high crystallinity in the material, and iii) homogeneity in the distribution and particle size of the material.