1. Introduction
Anaerobic digestion (AD) is a biological treatment, widely used worldwide, useful for energy recovery in the form of biogas and stabilization of organic matter (OM) [1,2]. A variety of organic waste from different agricultural, industrial, and urban activities is amenable to be treated by AD. One of the most commonly used substrates is manure, thus AD is considered a promising alternative to mitigate emissions of greenhouse gases (GHG) from this material [3].
However, in order to increase the methane yield of cattle manure (CM) researchers have conducted studies applying codigestion of CM with other organic waste. This approach considers that codigestion of organic waste offers clear benefits: it provides a balance of nutrients (C/N ratio and macro and micro nutrients content) [1,4]; contributes to dilute inhibitors from toxic compounds; generates greater buffering capacity due to increase of ammonia from organic waste; improves the maximum organic loading rates acceptable; increases the biogas yield obtained with respect to a single substrate; increases the quality of the digestate and improves the stability of the process[5]. These benefits, which are presented both at bench scale and pilot scale, have enabled the development of research on the subject in recent years [4,6]
Particularly, CM is one of the main organic waste generated in agricultural activities in Colombia, which in many cases is not used or appropriately disposed of and, consequently, causes negative impacts on the environment. According to the UPME [7], 83,497,181tons/year of cattle manure are generated in Colombia, which represent an energy potential of 71,771 TJ/year. Unfortunately, despite AD being a well-established technology in the rural sector of many countries that is not the case in Colombia, where AD is not very well developed.
A specific case is illustrated in the cold climate municipality of Cumbal (Nariño, Colombia), whose average temperature is 10°C all year long, and where one of the main productive activities is the exploitation of dairy cattle [8]. This economic activity generates a significant amount of cattle manure that is currently not being used or adequately disposed of. Additionally, there are other organic wastes, generated in complementary agricultural activities such as milk production (dairy wastewater (DW) and guinea pig breeding (guinea pig manure (GPM)), that, equally, are not being used.
In order to promote the implementation of AD, it is necessary to conduct research that reveals the technology’s operation and applicability with the available local organic waste. For its practicality and economy, the Biochemical Methane Potential (BMP) test is one of the most used when the feasibility of AD is to be evaluated. The test may also be applied to determine the best substrates or co-substrates in terms of maximum methane potential. The production of methane influences both the design and the economics of a biogas plant[9].
The aims of this research were to improve the methane production from CM through its co-digestion with GPM and/or DW, generated in the cold climate municipality of Cumbal (Nariño, Colombia), and to study the behavior of two real-scale biodigesters, operating them with the best conditions found in the lab and using bioclimatic strategies to increase the internal temperature of the biodigesters.
2. Materials and methods
2.1. Substrates and co-substrates
CM was used as main substrate and GPM and DW as co-substrates. CM and GPM were collected from a farm located in the municipality of Cumbal (Nariño, Colombia), a cold climate zone whose main activity is the raising of dairy cattle and guinea pigs. On the other hand, DW was collected from a milk storage facility belonging to a community association in the same locality. Samples were taken as required, characterized [Table 1], and kept at -4°C until the start of the experiment. GPM was size-reduced to a range of 4 mm to 6 mm.
2.2. Inoculum
The inoculum used was sludge from an anaerobic digester treating cattle manure, operating at ambient temperature (13ºC on average) in the municipality of Pasto (Nariño, Colombia). The inoculum selected met the quality criteria required by the literature [10] with the following characteristics: 9.5% Total solids (TS); 62.2% Volatile Solids (VS); 7.5 pH; 0.975 ± 0.036 g CH3COOH/L total Volatile Fatty Acids (VFAs); 0.252 g N-NH4/L ± 0.011 ammonia nitrogen; 3.520 g CaCO3/L ± 0.070 alkalinity. In addition, the positive control test demonstrated the capacity of the inoculum to degrade microcrystalline cellulose with a BMP of 0.36 ± 0.03 Nm3 CH4/kg VS, value within the range of 0.352 - 0.414 Nm3 CH4/kg VS accepted for standard cellulose [11]. Pre-exposure to the substrate is considered a good strategy to improve the biodegradation potential of an inoculum [12]; thus, a representative sample of the inoculum was taken to the laboratory, where it was acclimatized for one month at ambient temperature and fed with 1 g/L/day of the substrate and co-substrates.
2.3. Reactors and operation
The experiments were carried out following BMP protocols [9]. Glass bottles of 250 mL with an effective volume of 200 mL, hermetically sealed with stoppers and control gas opening valves, were used as experimental units. The tests were performed using a concentration of substrate of 2 g VS/L and an Inoculum/Substrate ratio of 2:1 based on VS; these are considered typical values for the BMP test [13]. The experiments were conducted within the mesophilic temperature range, at 37°C±1. The experimental units were shaken manually twice a day during all the experiment. The treatments were evaluated by triplicates and control bottles without substrate were also included to correct the methane production from the inoculum. The experiments were carried out for 60 days.
The biogas production was measured daily using the liquid displacement technique, and representative biogas samples were taken to determine the concentration of methane by gas chromatography. Furthermore, periodically liquid samples were taken in order to analyze control parameters such as pH, Volatile Fatty Acids (VFA), ammonia, and alkalinity. For some treatments, it was necessary to adjust the pH using a solution of NaOH (1 M).
2.4. Methane potential of individual co-substrates and effect of addition of nutrients
BMP test was carried out for each one of the co-substrates with and without nutrients with the purpose of determining the methane potential of co-substrates GPM and DW, individually, as well as the effect of the addition of nutrients. For the preparation of the nutrient solution, 0.5 g of Cysteine hydrochloride, 2.6 g of sodium bicarbonate, and solutions A, B, C, D and E presented in the "description of anaerobic basic medium" by [9] were added in quantities of 10, 2, 1, 1 and 1 ml respectively, and the volume was made up to 1 litter with distilled water. For the treatments with DW, in the preparation of the nutrient solution, distilled water was substituted for DW. For the treatments with GPM, the volume of each reactor was completed with the nutrient solution.
2.5. Effect of inoculum and co-substrates on methane potential of cattle manure
In order to determine the effect of inoculum and co-substrates on the methane potential of CM, 8 treatments were evaluated. The treatments were organized in a factorial structure (2*4) with a completely randomized design with three repetitions. The inoculum had two levels: with and without inoculum; and the co-substrate had four levels: without co-substrate, co-substrate GPM, co-substrate DW, and the co-substrate GPM + DW [Table 2]. In treatments 2, 4, 6 and 8, it was used a CM:GPM ratio of 7:3 in terms of VS, which was defined considering the average amount of recoverable CM and GPM generated in the farms in the study area but keeping the CM as the main substrate. In treatments 1, 2, 5 and 6, the effective volume was completed with distilled water. In treatments 3, 4, 7, and 8, the effective volume was completed with DW to maintain the substrate concentration of 2 g VS/L.
2.6. Full-scale implementation
At full scale, two biodigesters (B1 and B2) were implemented on a farm in the Cumbal municipality. Low-cost tubular digesters were selected due to their simplicity and easy operation. In the design and implementation of the biodigesters, the recommendations of the literature [14] were taken into account.
Each biodigester had a total and effective volume of 8 and 6 m3, respectively. Given the low temperature on site (10 °C on average), bioclimatic elements were implemented in order to increase the internal temperature of the biodigesters; thus, an expanded polystyrene insulation of 3 cm of thickness was installed in biodigester B1 and an insulation of bamboo in biodigester B2. In the case of the bamboo insulation, a shade screen was also installed to protect the system. Additionally, a greenhouse plastic covering was installed to protect the two biodigesters and to increase their internal temperature. Each biodigester was loaded up to 35% of effective volume, including 5% of inoculum in terms of VS; this inoculum was the same used on the lab scale. The daily feeding was 20 kg of manure (CM: GPM of 7:3 in weight) and 60 liters of DW, corresponding to an OLR of 0.4 g SV/L/day.
The experiment was conducted for a period of 4 months. Liquid samples were periodically taken to analyze control parameters. The temperature of the system was studied during the third month on 5 different days. Three daily measurements were recorded, the external temperature, the temperature inside the greenhouse, and the internal temperature of the biodigesters. The temperature inside the biodigesters was measured using a portable digital thermometer (HI 98103, Hanna Instrument). The estimation of biogas production was made through its combustion in a controlled-flow gas stove. In addition, using the volumetric method, comparisons were made to determine the percentage of methane in the biogas, and in the third month of operation, specific biogas samples were taken to analyze their methane content using chromatography.
2.7. Analytical methods
Characterization of substrate, co-substrates and inoculum, as well as the control parameters, were performed according to the standard methods [15], as follows: VFAs (5560 C. Distillation method), ammonia (4500-NH3 E. Titration method), alkalinity (2320B. Titration method), pH (pH meter), Chemical Oxygen Demand (COD) (5220 D. Closed reflux, colorimetric method), ST (2540 B. Total solids dried at 103-105 °C), SV (2540 E. Fixed and volatile solids incinerated at 550 °C), Total Kjeldahl Nitrogen (TKN) (4500-Norg.B. macro-Kjeldahl method). Cellulose, hemicellulose, and lignin were determined by the Van Soest method through the procedures of neutral detergent fiber, acid detergent fiber, and acid detergent lignin. For methane content, the volumetric method was carried out, according to the literature [16]. Chromatographic analyses of biogas were made in a SHIMADZU GC17A gas chromatograph, column: DB-WAX (Agilent Scientific, 30 m x 0.25 mm x 0.25 μm), Detector: FID at 280 °C, Injector: split/splitless at 250 °C. Injection split 1:10 and mobile phase: Helium AP at 1.0 ml/min. Before analyzing the results, biogas production data were converted to normal conditions (temperature of 0°C and 1 atmosphere of pressure). For CM and GPM, the theoretical BMP was calculated based on the elemental composition developed in [17] using Equations 1 and 2 [18]; for DW, it was calculated assuming that the consumption of 1 g of COD corresponds to 350 mL of methane at standard temperature and pressure using Equation 3 [18].
The factor 22.4 corresponds to the molar volume (L/mol) at 273.15 K and 1 atm.
3. Results and discussion
3.1 Methane potential of individual co-substrates and effect of addition of nutrients
The methane production started almost equally for GPM and DW, but after day 3, it increased very rapidly for GPM, while for DW, it seemed to stop [Figure 1]. This behavior coincided with a drop in pH, which was more severe for DW [Figure 2]. On day 8, it was observed that the pH for the treatments with GPM increased up to 6.8 while for the treatments with DW remained below 6.0; therefore, for DW the pH was adjusted to neutrality. However, in spite of pH adjustment for DW treatments, the methane production did not increase until day 18 of the experiment. From that point onwards, the methane production recovered to levels comparable to that of GPM.
At the end of the experiment, a maximum methane potential of 0.465 and 0.418 Nm3 CH4/kgVS for DW and GPM were obtained, respectively. No significant differences were found (p-value = 0.282) between treatments with or without nutrients, as well as between substrates GPM and DW. These results demonstrate that both GPM and DW contained the necessary nutrients for optimal bacterial growth. In the case of GPM, this statement is supported by [19], who mention that manure is rich in a wide variety of nutrients necessary for optimal bacterial growth, which is why some BMP tests are developed without the addition of supplementary nutrients [20]. The fact that there were no significant differences in the use or not of nutrients allowed us to verify that GPM and DW correspond to an appropriate selection of co-substrates for the AD of CM and; in turn, an appropriate selection of co-substrate allows operating AD processes without supplementary nutrients, offering operational and economic advantages to the AD process [21] since the use of nutrients, especially in a full-scale process, would mean additional costs [22]. Although the GPM treatments did not show greater potential, they showed greater stability; therefore, this residue could be treated in monodigestion; On the contrary, due to the low pH presented by DW treatments, it may not be advisable to treat this residue in monodigestion, since low pH values could inhibit the activity of methanogens [23].
The VFAs alkalinity ratio (buffer capacity) is considered a stability criterion of the AD process [24-26]. A VFAs alkalinity ratio below or around 0.4 represents a stable process, between 0.4 and 0.8, slightly unstable, and above 0.8 is considered significantly unstable [27]. The VFAs Alkalinity ratio in all the treatments evaluated was slightly above 0.4, but at the end of the experiment, values very close to 0.4 were observed, and even the DW-N and DW-WN treatments presented a VFAs alkalinity ratio below 0.4. However, it is important to note that the optimal ratio of these two parameters varies depending on the process conditions[28].
3.2 Effect of inoculum and co-substrates on methane potential of cattle manure
From the beginning of the experiment, the methane production was higher in treatments containing inoculum compared to those without inoculum, and the trend continued until the end of the trial [Figure 3], although after 40 days, the treatment CM + GPM + DW exceeded one of the treatments containing inoculum, CM + I treatment. After day 15, it was evident that the higher methane potential occurred in treatments containing both inoculum and DW.
The final methane potential of the treatments showed significant differences (p < 0.001). At 60 days, the treatments with DW and inoculum had the highest methane potentials 0.671 and 0.605 Nm3 CH4/kg VS for the treatments CM + GPM + DW + I and CM + DW + I, respectively; followed by treatments CM + GPM + I, CM + GPM + DW and CM + I; and finally, the treatments with the lowest methane potential were CM, CM + GPM and CM + DW. Comparing the experimental and theoretical BMP of treatment CM + GPM + DW + I, 69.07% of the theoretical BMP was produced experimentally, significantly higher than the percentage obtained individually for CM and DW, 43.81% and 34.49%, respectively. However, for individual GPM, the experimental BMP was 78.38% of the theoretical, higher than the theoretical BMP percentage obtained for the mixture of all substrates.
The significant increase of the methane potential when CM was co-digested with DW may be due to the balance of nutrients that DW provided to the digestion process. It is important to notice that DW contains milk which has vitamins and readily biodegradable carbohydrates, such as lactose [29], that dissolved in the medium may contribute to a rapid startup. On the other hand, thanks to the buffer capacity of CM, the acidification caused by DW was prevented, a problem observed when DW was digested as a single substrate [Figure 1]. Thus, the codigestion, as described in [5], improved the process stability.
In the same way, it could be noticed that the inoculum also had a positive influence on methane production. In this case, an inoculum with an important amount of well-adapted microorganisms increased the amount of active methanogens stimulating the methanogenic activity and, therefore, the methane production, as was described by [30] and [31] who mentioned that the quality and quantity of inoculum is a key factor for the implementation of the AD process. These authors concluded that an adequate amount of inoculum improves hydrolysis, accelerates biogas production, reduces time operation and helps prevent acidification.
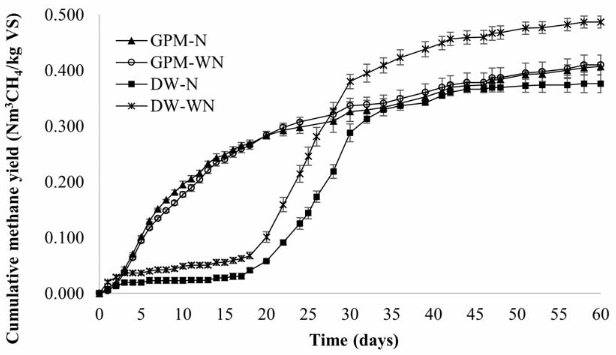
Figure 1 Methane potential of guinea pig manure (GPM) and dairy wastewater (DW) with (N) and without nutrients (WN)
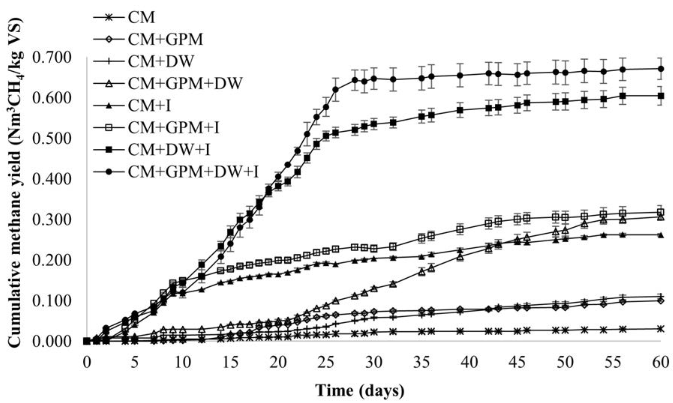
Figure 3 Methane potential of the codigestion of cattle manure (CM) with guinea pig manure (GPM) and dairy wastewater (DW), with (I) and without inoculum
These advantages were reflected in the rapid onset of methane production in treatments with inoculum since hydrolysis is the first step of AD, and its proper development facilitates the following stages to proceed until methane production [32]; in addition, the time in which 90% of methane was obtained was lower for all the treatments that contained inoculum, with differences in the range of 8 to 24 days for the treatments that contained CM and CM+GPM+DW, respectively; finally, none of the treatments that contained inoculum presented risk of acidification. Nonetheless, in many cases, the digestion of cattle manure is carried out without the addition of any inoculum, as reported in [33]. The reason for this is that the cattle manure contains anaerobic microorganisms; thus it could be used as substrate and inoculum. However, the disadvantage of not using inoculum is that a long lag phase can be experienced at the start of the process, as observed in the present experiment.
Table 3 shows the methane potential obtained for the 8 treatments. Treatments with CM, CM + GPM, and CM + DW presented yields of 0.031, 0.100, and 0.111 Nm3 CH4/kg VS, respectively; values well below the yields reported in [34], where a comparison of methane production from different substrates is presented, among which is cattle manure. The methane yields reported by these authors are in the range of 0.166 to 0.590 Nm3 CH4/kg VS, where the lowest value corresponds to cattle manure as a single substrate, and the maximum value to the codigestion of cattle manure with glycerin. The results of these treatments are also below those reported by [35], who obtained a potential between 0.125 to 0.166 Nm3 CH4/kg VS testing different compositions of cattle manure resulting from the variation of the diet of animals.
The methane yield in treatments CM + GPM + DW, CM + I y CM + GPM + I was 0.306, 0.262, and 0.317 Nm3 CH4/kg VS, respectively; these values are higher than those reported by [36], who obtained a methane yield of 0.204 Nm3 CH4/kg VS using dairy cattle manure and inoculum from a digester treating CM. The addition of GPM did not lead to a significant difference in the potential of methane; however, a slight increase was observed compared with CM alone. This is contrary to what was reported by [37], who found that the biogas potential decreased when CM was co-digest with GPM in a tubular biodigester, at an altitude of 2800m.
The highest methane potentials obtained for the treatments CM + GPM + DW + I and CM + DW + I are close to that reported in [34], where a value of 0.590 Nm3 CH4/kgVS for codigestion of cattle manure with glycerin was reported. These potentials are also close to the upper range reported in [6], from 0.18 to 0.6 Nm3 CH4/kgVS for CM with different substrates. However, the potentials obtained are lower than the methane potential observed in [38] for livestock manure from western and eastern Europe and North America, with values of 1.000 and 0.908 Nm3 CH4/kgVS.
No significant differences were found between the two treatments with the highest potentials. However, for the CM + GPM + DW + I treatment, a higher value was observed compared to CM + DW + I, perhaps because the mixture of the two co-substrates provided additional vitamins and a better balance of nutrients. In addition, there were differences in the time taken to reach 90% of the methane produced [Table 3]. The treatment with higher methane production (CM + GPM + DW + I) produced 90% of its potential in the shortest time, 26 days. Six days later, the CM + DW + I treatment reached this condition. By day 42, 90% of the methane potential of CM + I and CM + GPM + I treatments were obtained. Finally, the treatments that took the longest time to achieve 90% of their methane potential were CM, CM + GPM + DW, CM + DW, and CM + GPM at 50, 50, 52, and 54 days, respectively. The time to reach 90% of its methane potential in treatment CM + GPM + DW + I (26 days) is comparable to that obtained by [36], who obtained 90% of the biogas production of CM at 28 days of digestion, when they evaluated the BMP of five different types of manure. These differences in the days of digestion of the evaluated treatments can represent important advantages in a real-scale AD system.
A decrease in pH was observed in the early days coinciding with the hydrolysis process [Figure 4]. As the process advances, pH tends to stabilize towards neutrality as common in AD. It is noteworthy that the treatments with DW and without inoculum experienced the greatest drops of pH, in the same way as in the first experiment. Therefore, it was necessary to adjust the pH for these treatments on days 4 and 8. Likewise, pH was adjusted for treatments referred to as CM and CM + GPM on day 8. In contrast, none of the treatments with inoculum required pH adjustment since their pH values throughout the experiment were within the optimum range of 6.5-7.2 [39]. Thus, it can be said that methane production from DW, in addition to a suitable inoculum, requires a cosubstrate that provides a balance of nutrients. Given the low carbon content of DW, a possible cosubstrate could be agricultural waste since it is a good source of carbon [27].
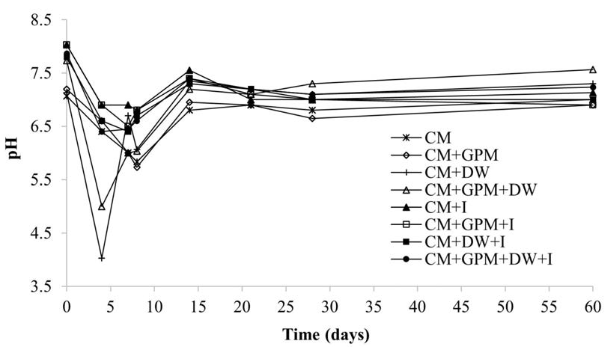
Figure 4 Behavior of pH in the anaerobic codigestion of CM with GPM and DW, with (I) and without inoculum
In the treatments with the highest methane production (CM + GPM + DW + I and CM + DW + I),VFAs increased until day 3; after this, they decreased gradually until the end of the experiment [Figure 5], following a normal batch AD process [30]. For treatments containing inoculum and DW, the production of VFAs during the first days of the process was significantly higher than the other treatments, meaning that DW, as co-substrate, contributed with components of easy degradation, while the inoculum provided necessary hydrolytic and acidogenic microorganisms to guarantee VFAs production. The results showed that the VFAs produced were effectively consumed during the digestion process in the inoculum and DW treatments.
If VFAs are not consumed during the AD process, it could lead to a decrease in pH causing inhibition or even the failure of the process [39]. This was probably what happened in treatments without inoculum and containing DW (CM + DW and CM + GPM + DW), since these treatments presented lower pH values during the first 5 days. No significant decrease of VFAs was observed until day 14 for treatment CM + GPM + DW and day 28 for CM + DW. However, it should be noted that in all treatments, the concentration of VFAs did not exceed 2 g/L, which is reported as the starting point of possible enzyme inhibition in batch AD processes [40].
In suitable concentrations, ammonia is used as a nutrient for bacterial growth, but if found in high concentrations, it can become toxic. In AD, high levels of ammonia affect the production of methane by inhibiting methanogenic microorganisms. For this reason, in the present research, this parameter was followed, founding differences in the treatments with and without inoculum Figure 6. Throughout the process, the ammonia values for the treatments without inoculum were maintained in the range of 22-110 mg/L, with concentrations considered to be beneficial for the AD process (below 200 mg/L). In contrast, for treatments with inoculum the ammonia concentration reached values above 200 mg/L, especially for the treatments containing DW (CM + DW + I and CM + GPM + DW+ I). In the treatments with inoculum and without DW (CM + I and CM + GPM + I), the concentrations of ammonia only exceeded 200 mg/L at the end of the process. Some authors suggest even lower values to guarantee the stability of the process, stating that although the concentration of ammonia that can cause inhibition depends on the type of substrate, type of inoculum, and environmental conditions such as temperature, pH and the acclimation periods [39], concentrations of ammonia higher than 150 mg/L are considered inhibitory [41]. However, ammonia contributes to buffering capacity, since it has the ability to react with VFAs, neutralizing them, avoiding inhibition by the accumulation of VFAs and favoring the production of biogas [39].
Treatments with the highest production of methane presented the highest COD removal: treatments CM + GPM + DW + I and CM + DW + I, with 92.24% and 88.04%, respectively. COD removal reflects high gasification efficiency, which is calculated as a percentage of COD in the feed converted to methane. For treatments CM, CM + I, CM + GPM, CM + GPM + I, CM + GPM + DW and CM + DW, a COD removal of 85.19%, 79.31%, 78.62%, 72.98%, 50.00% and 39.58%, was found, respectively. All COD removal percentages are higher than that reported by [36], 10.42% in batch AD of CM.
3.3 Full-scale implementation
Biogas production started faster for the B1 biodigester, about 20 days after start-up. For the B2 biodigester, biogas production was not observed until the third month. This difference in the start-up may be related to a more favorable internal temperature, as explained below (3.3.2.). After one month of follow-up, better performance in terms of biogas production for B1 was evident, but a lack of constancy in the feeding of the system by the owner family was observed. Therefore, in order to stabilize the system and motivate the family to take care of the biodigesters, a small amount of granular anaerobic sludge was added to each biodigester (500 ml, which corresponds to 0.008% of the volume of the biodigester). As expected, biogas production increased notoriously, filling the 2 m3 of biogas storage space after 3 days.
By the third month of operation, the biogas production of B1 [Figure 7] was sufficient to cook for 1 hour a day, in a stove designed to operate with 0.9 m3 of biogas per hour. While for B2, the production of biogas was still insufficient to be exploited. The biogas production of B1 continued to increase (>50%), eventually being enough to supply cooking requirements for a family of 5 people, especially during the three weeks of propane gas shortages that occurred in the region, at the end of the study. It is in line with the findings of [42], who found that the biogas produced by a low-cost digester could replace 80% of the traditional fuel (propane) in rural areas of Colombia. However, the sustainability of the system depends on the commitment of the owners to the required operation and maintenance.
The methane content was examined by the third month of operation of B1, and the percentage of CH4 in the biogas generated was between 61- 69%. This range coincides with the methane content in the biogas generated in domestic tubular digesters at a pilot scale in Latin America, reported by the literature between 40% and 70% [43] and, more specifically, with the percentage of methane showed by [42], who reports a 65.6% of methane content in biogas when monitoring a low cost digester in Colombia under realistic conditions and operated with CM. Besides, the values found are higher than the one reported for CM and whey mixture at a pilot scale, 51.4% of methane in biogas on day 56 [44].
Control parameters
The pH remained within the optimum range for the two biodigesters, only on day 74 the pH dropped to 6.34 in B2, recovering later without intervention. Ammonia values remained within ranges considered beneficial for AD. In B2, a significant increase in the ammonia concentration was observed on day 74, coinciding with the lowest pH value (6.34) but without exceeding the range of 200-1000 mg/L, in which no negative effects are expected. In addition, the ammonia values were well below the values considered as inhibitory (1500-3000 mg/L) [45].
In the two biodigesters, B1 and B2, alkalinity decreased until day 51, then increased, coinciding with the literature [44], which reported an increase in alkalinity from day 16 to 56. The alkalinity decrease up to day 51 was due to the degree of dilution of the material due to the amount of water added at the beginning of the process.
The COD removal was higher than 50% for the two biodigesters; even most of the time, it was higher than 85%. For B1 the removal was 92.69%, 90.97%, 89.54%, 56.90% and 86.09%; and for B2 it was 95.03%, 93.24%, 89.20%, 56.90% and 93.91% for days 35, 55, 65, 75 and 90, respectively. These removal percentages coincide with what was reported by [46], who informed reductions of over 90% in an AD process of nine months of operation.
Effect of bioclimatic strategies on biodigesters temperature
With the use of insulation and the greenhouse cover, the temperature inside the biodigesters was significantly increased with respect to the external temperature. The increase in the temperature inside biodigester B1 was 10°C and, in B2, it was 8°C, showing that the expanded polystyrene insulation was slightly more efficient than the bamboo insulation. During the 5 days of evaluation, the external temperature varied between 2.0 and 15.0°C with an average temperature of 9.3°C, the temperature inside the greenhouse varied between 10.0 and 58.0°C, with an average temperature of 24.6°C, and the temperature inside biodigesters varied between 11.0 and 22.0°C, for B1, with an average temperature of 19.1°C and between 10.1 and 20.0°C, for B2, with an average temperature of 17.1°C. These results are close to those obtained by [47] who observed a slurry temperature 8.4°C above the external temperature when monitoring a low-cost tubular digester, installed in cold weather with insulation and greenhouse environment. The higher internal temperature in B1 may be the reason for the faster and higher biogas production in this biodigester. Thus, the insulation and the greenhouse plastic covering improved the process conditions.
4. Conclusions
A significant increase in methane production from cattle manure was achieved with the addition of inoculum and co-substrates guinea pig manure and dairy wastewater. Moreover, the advantages of the addition of an adapted inoculum were reflected in the behavior of pH, since it prevented acidification. The dairy wastewater significantly increased the methane potential when digested with cattle manure or with guinea pig manure; otherwise, no significant increase was observed when guinea pig manure was digested with cattle manure. The best combination for methane production was the mixture of cattle manure with guinea pig manure and dairy wastewater, without the need of any addition of nutrients. The inclusion of bioclimatic strategies in the construction and implementation of biodigesters in a cold climate makes it viable by significantly increasing the internal temperature. The management and use of cattle manure in codigestion with guinea pig manure and milk wastewater, through the use of low-cost tubular biodigesters, reduce the environmental impacts caused by this waste and offers a renewable energy source for rural families in the municipality of Cumbal (Nariño-Colombia). However, the sustainability of the system depends on the commitment of the owners to carry out an adequate operation and maintenance of the system, with the technical support required.