Services on Demand
Journal
Article
Indicators
-
Cited by SciELO
-
Access statistics
Related links
-
Cited by Google
-
Similars in SciELO
-
Similars in Google
Share
Agronomía Colombiana
Print version ISSN 0120-9965
Agron. colomb. vol.32 no.2 Bogotá May/Aug. 2014
https://doi.org/10.15446/agron.colomb.v32n2.43731
http://dx.doi.org/10.15446/agron.colomb.v32n2.43731
POSTHARVEST PHYSIOLOGY AND TECHNOLOGY
1 Department of Chemistry, Faculty of Sciences, Universidad Nacional de Colombia. Bogota (Colombia). cenarvaezc@unal.edu.co
Received for publication: 29 October, 2013. Accepted for publication: 19 March, 2014.
ABSTRACT
Because the use of drying at high temperatures might negatively affect the functional properties of fruits, the effect of air-drying at 60°C on the total phenolic content (TPC) and antioxidant capacity (AOC) of cape gooseberry fruit was evaluated at three ripeness stages. The AOC was evaluated with 2,2'-azino-bis(3- ethylbenzothiazoline-6-sulfonic acid) (ABTS ), ferric reducing ability of plasma (FRAP), 1,1-diphenyl-2-picrylhydrazyl (DPPH), and b-carotene-linoleate assays. The TPC and AOC increased in the fresh fruit as the ripeness stage increased. The TPC increased from 401.8±19.8 to 569.3±22.3 mg GA E/100 g dry weight (DW). The AOC values obtained with ABTS in the fresh fruit (ranging from 79.4±4.5 to 132.7±12.9 mmol trolox/g fruit DW) were comparable to those obtained with FRAP (ranging from 82.9±16.3 to 153.9±31.7 mmol trolox/g fruit DW). The values assessed with DPPH ranged from 21.0±3.2 to 34.1±5.1 mmol trolox/g fruit DW. The b-carotene-linoleate assay gave values ranging from 5.8±1.1 to 12.7±2.0 mmol a-tocoferol/g fruit DW. Air-drying the cape gooseberry fruit had a small influence on the TPC. The air-dried fruit had AOC values ranging from 27 to 164% of the value of the fresh fruit. While the ABTS assay produced higher values in the air-dried fruit than in the fresh fruit, the FRAP, DPPH, and b-carotene-linoleate assays resulted in lower values in the air-dried fruit.
Key words: tropical fruit, functional food, polyphenols.
RESUMEN
Debido a que el secado a altas temperaturas puede afectar negativamente las propiedades funcionales de las frutas, se evaluó el efecto del secado con aire a 60°C sobre el contenido total de fenoles (CTF) y la capacidad antioxidante (CAO) de frutos de uchuva en tres estados de madurez. CAO se evaluó por los métodos ácido 2,2'-azino-bis(3-etilbenzotiazolin-6-sulfonico) (ABTS), habilidad reductora de hierro en plasma (FRAP), 1,1-difenil-2-picrilhidrazil (DPPH) y b-caroteno-linoleato. En la fruta fresca los valores de CTF y CAO se incrementaron al aumentar el estado de madurez. CTF se incrementó desde 401,8±19,8 hasta 569,3±22,3 mg GA E/100 g peso seco, PS. Los valores de CAO obtenidos por ABTS en la fruta fresca (desde 79,4±4,5 hasta 132,7±12,9 mmol trolox/g fruta PS) fueron similares a los obtenidos por FRAP (desde 82,9±16,3 hasta 153,9±31,7 mmol trolox/g fruta PS). Los valores obtenidos por DPPH variaron entre 21,0±3,2 a 34,1±5,1 mmol trolox/g fruta PS. El ensayo de b-caroteno-linoleato arrojó valores entre 5,8±1,1 a 12,7±2,0 mmol a-tocoferol/g fruta PS. El secado con aire tuvo una influencia pequeña en los valores de CTF. La fruta seca tuvo valores de CAO que representaron entre 27 a 164% de los valores obtenidos en la fruta fresca, con valores mayores en la fruta seca que en la fresca para ABTS y valores menores para FRAP, DPPH y b-caroteno-linoleato.
Palabras clave: fruta tropical, alimento funcional, polifenoles.
Introduction
The cape gooseberry (Physalis peruviana L.), native to the Andean region, is a berry with an ovoid shape and 1.2-2.5 cm diameter, containing 100-300 seeds in a calyxcovered fruit (Fischer et al., 2011). The cape gooseberry is a climacteric fruit grown in countries such as Colombia, Peru, Venezuela, Egypt, South Africa, and Australia, among others (Ramadan, 2011). The cape gooseberry is the second most exported fruit from Colombia, after the banana, with European countries as the main customers: 3,225 t being exported to The Netherlands, 1,610 t to Germany, 966 t to Belgium, 115 t to Luxemburg, 214 t to Sweden, and 130 t to France (Fischer et al., 2011). In Colombia, an area of 1,000 ha is estimated to be dedicated to cape gooseberry plantations, with fresh fruit production ranging from 15 to 28 t ha-1 (Fischer et al., 2011).
The cape gooseberry is commercialized in the domestic and international Colombian markets as fresh fruit. In Colombia, the cape gooseberry fruit is sold at three different ripeness stages according to the color of the peel (light orange, stage 4; orange, stage 5; and deep orange, stage 6) (Icontec, 1999). Commercialization of the air-dried fruit is a is a relatively inexpensive technique that extends shelf-life due to the reduction of water activity. As a negative effect, air-drying fruits and vegetables can negatively affect their nutritional and sensorial characteristics (Santos and Silva, 2008). A recent study showed that both oven drying and drum drying of frozen-stored whole fruit and frozen-stored slurry of the cape gooseberry fruit reduce the total phenolic content and the antioxidant activity as measured with a DPPH assay (Valdenegro et al., 2013). Air-drying fresh cape gooseberry at temperatures ranging from 50 to 100°C changed the total phenolic content and the antioxidant capacity as measured with DPPH and FRAP assays (Izli et al., 2014; López et al., 2013). No attempts on gauging the effect of air-drying fresh cape gooseberry fruit at different ripeness stages on the antioxidant activity have been made. The objective of this research was, therefore, to evaluate the effect of air-drying cape gooseberry fresh fruit in three different ripeness stages (light orange, stage 4; orange, stage 5; and deep orange, stage 6) on the total phenolic content and antioxidant capacity.
Materials and methods
Reagents
All trans-b-carotene; a-tocopherol; 6-hydroxy-2,5,7,8- tetramethylchroman-2-carboxylic acid (Trolox); 2,2â²-azinobis-(3-3-ethylbenzothiazoline-6-sulfonic acid) (ABTS); dithiothreitol; 2,2-diphenyl-1-picrylhydrazyl (DPPH); 2,4,6-tripyridyl-S-triazine (TPTZ); and Folin Ciocalteauphenol reagents were supplied by Sigma (St. Louis, MO) and were used as they were received. Other chemicals came from Panreac Quimica (Barcelona, Spain).
Plant material
The cape gooseberry fruits were randomly collected from a commercial plantation in the municipality of Ramiriqui (Boyaca department), located in the central part of Colombia, 148 km northeast of Bogota, at 2,313 m a.s.l. with an average temperature of 16°C. The cape gooseberry fruits were visually selected at three degrees of ripeness, according to the color of the peel. This selection was based on the color specifications given in the Colombian Technical Standard NT C 4580 (Instituto Colombiano de Normas Técnicas y Certificación, Icontec, 1999). The fruits were allowed to mature on the plants and harvested at the ripeness stages: 4, light orange; 5, orange; and 6, deep orange. The samples were immediately transported to Bogota and processed.
Preparation of samples and processing
The calyxes were carefully removed from the fruit by hand. The fruit samples were washed with tap water and gently wiped with paper tissue. Fresh samples (without cutting them, 500 g fruit) were placed on 40 x 30 cm stainless steel food trays and loaded into a tangential air-flow cabinet. Natural gas-heated air at 60°C, at a flow of 1,200-1,500 m3 h-1, with a relative humidity ranging from 40 to 60%, was used. The samples were removed from the air-flow cabinet after 16 h, allowed to cool to room temperature, packed in polyethylene bags, and stored at -20°C until the analyses. The fruits were also analyzed before air-drying.
Compositional indexes
Water content, total titratable acidity (TTA ), and total soluble solids (TSS , °Brix) were measured in the fresh fruit. Water content was determined using the official AOAC (2000) method 934.06.27. TTA was measured by titrating the samples (2 g of homogenate plus 25 mL of CO2-free distilled water) with standardized 0.1 M NaOH to pH 8.2 using an Orion Advanced pH meter, model Orion 2-Star (Thermo Scientific, Boston, MA ). Because citric acid is the main non-volatile carboxylic acid in the cape gooseberry (Fischer et al., 2000), the TTA was expressed as mg of citric acid/100 g of fruit fresh weight (FW). The TSS was assessed using a digital refractometer Abbe II (Reichert-Jung, Leica Inc., Buffalo, NY ). The TSS /TTA ratio was calculated.
Total phenolic content and antioxidant capacity
The extraction was performed according to previous studies (Restrepo-Sánchez et al., 2009). A 1 g fruit sample was placed in a flask, equipped with a reflux condenser, and extracted with 20 mL of 50% (v/v) aqueous methanol by stirring on a magnetic stir plate for 30 min at 50°C. After cooling to room temperature, the mixture was centrifuged (4,000 x g, 10 min, 4°C) and the supernatant was kept at 4°C. The pellet was extracted four times with 5 mL of 70% (v/v) aqueous acetone for 30 min at 50°C. The five supernatants were mixed, the volume was graduated to 50 mL in a volumetric flask with distilled water, and used for the analysis of the total phenolic content (TPC) and antioxidant capacity (AOC).
The TPC was determined using the Folin-Ciocalteu (FC) method as described by Velioglu et al. (1998). A 100 mL extract was mixed with 750 mL of 10% (v/v) FC reagent. After 5 min, 750 mL of a 6% (w/v) Na2CO3 solution were added. After 90 min, the absorbance of the reaction mixture was measured at 725 nm. The results were expressed as gallic acid equivalents (mg GAE per 100 g of dry weight (DW) of fruit). Calibration curves for gallic acid were performed in concentrations ranging from 0.01 to 0.30 mg/mL (five data points, r2 = 0.996).
The AOC of the extracts was measured by the ABTS , FRAP, DPPH, and b-carotene-linoleate bleaching assays. The ABTS assay was based on Re et al. (1999). A mixture of 7.0 mM ABTS and 2.45 mM potassium persulphate was prepared and stored in dark conditions for 16 h. The resulting ABTS+• solution was diluted with ethanol to reach an absorbance of 0.70 at 734 nm. Aliquots of 10 mL of extracts were incubated with 1,000 mL of the ABTS+• solution at 30°C and the absorbance was monitored at 734 nm every 5 s over a period of 60 min.
The FRAP assay was carried out according to the method described by Benzie and Strain (1996). The FRAP reagent was prepared by mixing 25.0 mL of a 300 mM sodium acetate buffer (pH 3.6) with 2.5 mL of 10 mM TPTZ in 40 mM HCl, and 2.5 mL of 20 mM ferric chloride. The FRAP reagent (900 mL), distilled water (90 mL), and extract (30 mL) were mixed. The mixture was incubated at 37°C and the absorbance was monitored at 593 nm every 5 s over a period of 45 min.
The DPPH assay was performed according to Sánchez- Moreno et al. (1998). A methanolic stock solution of 50 mM DPPH was diluted with methanol to obtain a working DPPH solution with absorbance of 1.10 at 515 nm. The extract (25 mL) was mixed with 975 mL of working DPPH solution. The mixture was incubated at 20°C and its absorbance was measured every 5 s for 1 h at 515 nm. The scavenging effect (in percentage) was calculated as (A1-A2/A0)*100, where A0 was the absorbance of the control (without extract), A1 was the absorbance in the presence of the extract once the steady state plateau was reached, and A2 was the absorbance without DPPH. In the ABTS , FRAP, and DPPH assays, standard curves were generated with trolox in concentrations ranging from 100 to 1,500 mM (five data points, r2 = 0.999, 0.989, 0.975, respectively). In all cases, the absorbance reached the steady state within the given incubation time. Results from the ABTS , FRAP, and DPPH assays were expressed as mmol of trolox/g of DW fruit.
The b-carotene-linoleate bleaching assay was performed according to Velioglu et al. (1998). First, 500 mL of a 0.4 mg/mL b-carotene solution in chloroform was placed in a 100 mL plastic container and dried under N2. Next, 20 mL of linoleic acid, 200 mL of Tween® 20, 200 mL of the extract, and 50.0 mL of water saturated with air were added. The mixture was vigorously shaken, sonicated at 36 kHz for 10 min (Ultrasonic LC60H, Elma GmbH & Co., Singen, Germany), and incubated at 50°C for 2 h. The absorbance of the mixture was measured at 470 nm every 10 min during the given incubation time. The absorbance was plotted versus incubation time and the slope was calculated. A zero order kinetics was observed, which indicates that the depletion on the absorbance was caused by b-carotene bleaching and not by b-carotene precipitation (first order kinetics). The bleaching assay was also tested with a 50 mM a-tocoferol solution. The antioxidant activity of the extracts measured by the b-carotene-linoleate bleaching assay was expressed as mmol a-tocoferol/g of DW fruit.
Statistical analyses
The statistical analyses were performed using Statgraphics Plus® 5.1 for Windows (Manugistics, Rockville, MD). The mean values and their standard deviations were reported. One-way analyses of variance were performed for the compositional index results. Two-way analyses of variance were performed for the TPC and AOC. Comparisons among means were performed by Tukey's-test (P≤0.05).
Results and discussion
Compositional indexes
As shown in Tab. 1, as the ripeness stage of the cape gooseberry increased (evaluated by its color), the TSS increased and the TTA decreased. The TSS /TTA ratio of each stage was in agreement with the specifications given in NT C 4580 (Icontec, 1999). The TSS values of the cape gooseberry fruit were comparable to those of the tropical fruits mango (Mangifera indica) (14.2-21.7°Brix, depending on cultivar and storage temperature) and papaya (Carica papaya) (8.8-14.3 °Brix, depending on cultivar and geographical location), but the TTA values were higher than those of the mentioned fruits (0.03-0.42 g citric acid/100 g FW in mango and 0.09-0.19 g citric acid/100 g FW in papaya) (Vazquez-Salinas and Lakshminarayana, 1985; Wall, 2006; Schweiggert et al., 2012). The relatively high TSS /TTA ratio of the cape gooseberry agrees with the high-sour/low-sweet taste of the fruit.
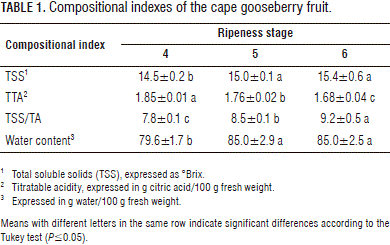
Total phenolic content (TPC)
As shown in Fig. 1, the TPC (in mg GAE/100 g DW) increased in the fresh fruit as the ripeness stage increased, from 401.8±19.8 (stage 4) to 569.3±22.3 (stage 6). When expressed in fresh weight (FW), the TPC (in mg GA/100 g FW) was 82.0±4.0 (stage 4), 81.0±2.8 (stage 5), and 85.4±3.3 (stage 6). The TPC values obtained in the current research were lower than those previously reported in cape gooseberry grown in Peru (154±3 mg GAE/100 g FW) (Repo and Encina, 2008), comparable to that grown in Ecuador (87±19 mg GAE/100 g FW) (Vasco et al., 2008), and greater than previous reports for Colombia (39±5 mg GAE/100 g FW) (Restrepo, 2008) and Chile (321.0±31.4 mg GAE/100 g DW) (López et al., 2013). The higher values reported here might be related, in addition to differences in geographic origin, to the extraction method. Besides a methanolic extraction, a more lipophilic extraction solvent, 70% (v/v) aqueous acetone, was also used. The increase in the TPC as the ripeness stage increased was in agreement with previous results for some mango cultivars (Sulaiman and Ooi, 2012).
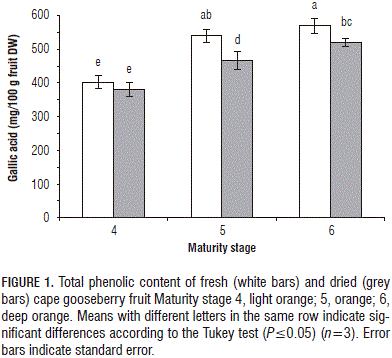
Air-drying the cape gooseberry fruit had a small influence, but statistically significant (P≤0.05), on the TPC. These values were reduced to 95, 86, and 92% of the original values after air-drying of the fruit at stages 4, 5, and 6, respectively. The moderate reduction in the TPC after air-drying the cape gooseberry was in contrast to the strong reduction in the TPC after air-drying in previous results on the type of fruit grown in Turkey (ripeness stage not reported) (Izli et al., 2014) and Chile (López et al., 2013), red pepper (Capsicum annuum) (Vega-Gálvez et al., 2009), saskatoon fruit (Amelanchier alnifolia) (Kwok et al., 2004), and apricots (Prunus armeniaca) (Madrau et al., 2009). Interestingly, López et al. (2013) reported that the modification of the TPC of air-dried cape gooseberry is temperature-dependant in a way that its content can be decreased but also increased. Furthermore, the stability in the air-drying of phenolic compounds observed in the cape gooseberry does not agree with the expected heat-degradation of the flavonols rutin (quercetin-3-O-rutinoside), myricetin, and kaempferol present in cape gooseberry fruit (Licodiedoff et al., 2013). It has been shown that rutin and other phenolics (e.g. chlorogenic acid and catechin) are susceptible to high temperatures (55°C) during the air-drying of apricots (Madrau et al., 2009). Likewise, the air-drying of mulberry (Morus alba) leaves (Katsube et al., 2009) at 70°C induces degradation of rutin, a kaempferol glycoside, and other phenolics. We hypothesize that the low reduction in the TPC of the air-dried fruit might be explained by the presence of other compounds, different from the aforementioned phenolics, that are stable under heat treatments and rencting to the FC reagent or that degradation products of the native phenolic compounds after air-drying have similar reactivity towards the FC reagent.
Antioxidant capacity (AOC)
Figure 2 shows the AOC of the cape gooseberry fruit as measured by the ABTS , FRAP, DPPH, and b-carotenelinoleate assays. The AOC values (expressed as mmol trolox/g fruit DW) obtained by ABTS in the fresh fruits (ranging from 79.4±4.5 in ripeness stage 4 to 132.7±12.9 in ripeness stage 6) were comparable to those obtained by FRAP (ranging from 82.9±16.3 in ripeness stage 4 to 153.9±31.7 in ripeness stage 6). In contrast, those values assessed by DPPH (ranging from 21.0±3.2 in ripeness stage 4 to 34.1±5.1 in ripeness stage 6) were much lower. When the AOC was evaluated by the b-carotene-linoleate assay, values ranging from 5.8±1.1 (ripeness stage 4) to 12.7±2.0 mmol a-tocoferol/g fruit DW (ripeness stage 6) were obtained. Furthermore, the AOC measured by the ABTS , FRAP, DPPH, and b-carotene-linoleate assays increased as the ripeness stage of the fruit increased. The increase in the AOC observed as the ripeness stage of the cape gooseberry increased agrees with results of AOCFRAP measured in papaya fruits during ripening when stored at 25°C (Ong et al., 2013). The greater antioxidant activity as the ripeness stage advanced observed in the cape gooseberry fruit was opposite to the trend described in guava and durian fruits (Arancibia-Avila et al., 2008; Gull et al., 2012).
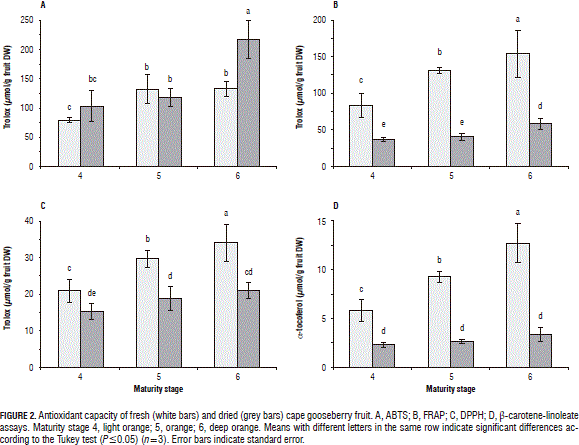
As a result of air-drying, the AOC measured by the FRAP, DPPH, and b-carotene-linoleate assays decreased, contrasting with the increase observed when the AOC was measured by the ABTS assay at ripeness stages 4 and 6. A decrease of the TPC, together with an increase of the AOC has been described when the AOC was evaluated by the DPPH assay during the air-drying of apricots (Madrau et al., 2009), mulberry leaves (Katsube et al., 2009), and red pepper (Vega-Gálvez et al., 2009). It would be possible that reaction products of the Maillard type or degradation products of phenolic compounds were generated during the air-drying and that those compounds, resulting in a low reaction to the FC reagent, contributed to the controlling power of the ABTS+•. It is unknown, nevertheless, why these compounds enhanced the AOC-ABTS , but decreased the AOC-FRAP, considering the fact that, in both methods, the redox potential involved was similar (Prior et al., 2005). It might be that the compounds responsible for the divergent behavior in these two assays have a differential behavior at the different pH values that each assay involves (Prior et al., 2005). The decrease in the AOC as measured by the DPPH and FRAP methods after air-drying was in agreement with previous results from cape gooseberry fruit (López et al., 2013; Izli et al., 2014).
Conclusions
The total phenolic content (TPC) as well as the antioxidant capacity (AOC), as measured by the ABTS , FRAP, DPPH, and b-carotene-linoleate assays, increased as the ripeness stage of the cape gooseberry increased. While the TPC in the air-dried fruits represented values ranging from 86 to 95% of the fresh fruit, the AOC in the air-dried fruits produced values ranging from 27 to 164%, with ABTS giving higher values in the air-dried than in the fresh fruits and the FRAP, DPPH, and b-carotene-linoleate assays giving lower values in the air-dried fruits. The differential behavior of the AOC (large variation after air-drying) as compared to the TPC (small variation after air-drying) might indicate a large transformation of phenolic compounds, yielding reaction products with different behaviors towards the chemicals used to access the AOC, but with similar reactivity towards the FC reagent.
Literature cited
AOAC, Association of Offcial Analytical Chemists International. 2000. Moisture in dried fruits. Method 934.06.27. In: Horowitz, W. (ed.). Official Methods of Analysis of AOAC International. 17th ed. Gaithersburg, MD. [ Links ]
Arancibia-Avila, P., F. Toledo, Y.-S. Park, S.-T. Jung, S.-G. Kang, B.G. Heo, S.H. Lee, M. Sajewicz, T. Kowalska, and S. Gorinstein. 2008. Antioxidant properties of durian fruit as influenced by ripening. LWT - Food Sci. Tech. 41, 2118-2125. [ Links ]
Benzie, F.F. and J.J. Strain. 1996. The ferric reducing ability of plasma (FRAP) as a measure of ''antioxidant power'': The FRAP assay. Anal. Biochem. 239, 70-76. [ Links ]
Licodiedoff, S., L.A.D. Koslowski, and R.H. Ribani. 2013. Flavonol rates of gosseberry fruits Physalis peruviana determined by HPLC through the optimization and validation of the analytic method. Int. J. Food Sci. Nutr. Eng. 3, 1-6. [ Links ]
Fischer, G., G. Ebert, and P. Lüdders. 2000. Provitamin A carotenoids, organic acids and ascorbic acid content of cape gooseberry (Physalis peruviana L.) ecotypes grown at two tropical altitudes. Acta Hort. 531, 263-267. [ Links ]
Fischer, G., A. Herrera, and P.J. Almanza. 2011. Cape gooseberry (Physalis peruviana L.). pp. 374-396. In: Yahia, E.M. (ed.). Postharvest biology and technology of tropical and subtropical fruits. Vol. 2. Acai to citrus. Woodhead Publishing, Oxford, UK. [ Links ]
Gull, J., B. Sultana, F. Anwar, R. Naseer, M. Ashraf, and M. Ashrafuzzaman. 2012. Variation in antioxidant attributes at three ripening stages of guava (Psidium guajava L.) fruit from different geographical regions of Pakistan. Molecules 17, 3165-3180. [ Links ]
Icontec, Instituto Colombiano de Normas Técnicas y Certificación. 1999. Norma técnica colombiana uchuva NT C 4580. Bogota. [ Links ]
Izli, N., Y. Gökçen, H. Ünal, E. Isik, and V. Uylaser. 2014. Effect of different drying methods on drying characteristics, colour, total phenolic content and antioxidant capacity of goldenberry (Physalis peruviana L.). Int. J. Food Sci. Technol. 49, 9-17. [ Links ]
Katsube, T., Y. Tsurunaga, M. Sugiyama, T. Furuno, and Y. Yamasaki. 2009. Effect of air-drying temperature on antioxidant capacity and stability of polyphenolic compounds in mulberry (Morus alba L.) leaves. Food Chem. 113, 964-969. [ Links ]
Kwok, B.H.L., C. Hu, T. Durance, and D.D. Kitts. 2004. Dehydration techniches affect phytochemical contents and free radical scavenging activities of Saskatoon berries (Amelanchier alnifolia Nutt.). J. Food Sci. 69, 122-126. [ Links ]
López, J., A. Vega-Gálvez, M.J. Torres, R. Lemus-Mondaca, I. Quispe-Fuentes, and K. Di Scala. 2013. Effect of dehydration temperature on physico-chemical properties and antioxidant capacity of goldenberry (Physalis peruviana L.). Chilean J. Agric. Res. 73, 293-300. [ Links ]
Madrau, M.A., A. Piscopo, A.M. Sanguinetti, A. Del Caro, M. Poiana, F.V. Romeo, and A. Piga. 2009. Effect of drying temperature on polyphenolic content and antioxidant activity of apricots. Eur. Food Res. Tech. 228, 441-448. [ Links ]
Ong, M.K., C.F. Forney, P.G. Alderson, and A. Ali. 2013. Postharvest profile of a Solo variety 'Frangi' during ripening at ambient temperature. Sci. Hortic. 160, 12-19. [ Links ]
Prior, R.L., X. Wu, and K. Schaich. 2005. Standardized methods for the determination of antioxidant capacity and phenolics in foods and dietary supplements. J. Agric. Food Chem. 53, 4290-4302. [ Links ] [ Links ]
Re, R., N. Pellegrini, A. Proteggente, A. Pannala, M. Yang, and C. Rice-Evans. 1999. Antioxidant activity applying an improved ABTS radical cation decolorization assay. Free Radical Bio. Med. 26, 1231-1237. [ Links ]
Repo, de C.R. and Z.C.R. Encina. 2008. Determinación de la capacidad antioxidante y compuestos bioactivos de frutas nativas peruanas. Rev. Soc. Quím. Perú 74, 108-124. [ Links ]
Restrepo, A. 2008. Nuevas perspectivas de consumo de frutas: uchuva (Physalis peruviana L.) y fresa (Fragaria vesca L.) mínimamente procesadas fortificadas con vitamina E. M.Sc. thesis. Faculty of Agricultural Sciences, Universidad Nacional de Colombia, Medellin, Colombia. [ Links ]
Restrepo-Sánchez, D.-C., C.-E. Narváez-Cuenca, and L.-P. Restrepo- Sánchez. 2009. Extracción de compuestos con actividad antioxidante de frutos de guayaba cultivada en Vélez-Santarder, Colombia. Quim. Nova, 32, 1517-1522. [ Links ]
Sánchez-Moreno, C., J.A. Larrauri, and F. Saura-Calixto. 1998. A procedure to measure the antiradical efficiency of polyphenols. J. Sci. Food Agric. 76, 270-276. [ Links ]
Santos, P.H.S. and M.A. Silva. 2008. Retention of vitamin C in drying processes of fruits and vegetables-a review. Dry. Technol. 26, 1421-1437. [ Links ]
Schweiggert, R.M., C.B. Steingass, P. Esquivel, and R. Carle. 2012. Chemical and morphological characterization of Costa Rican papaya (Carica papaya L.) hybrids and lines with particular focus on their genuine carotenoid profiles. J. Agric. Food Chem. 60, 2577-2585. [ Links ]
Sulaiman, S.F. and K.L. Ooi. 2012. Polyphenolic and vitamin C contents and antioxidant activities of aqueous extracts from mature-green and ripe fruit fleshes of Mangifera sp. J. Agric. Food Chem. 60, 11832-11838. [ Links ]
Vasco, C., J. Ruales, and A. Kamal-Eldin. 2008. Total phenolic compounds and antioxidant capacities of major fruits from Ecuador. Food Chem. 111, 816-823. [ Links ]
Vazquez-Salinas, C. and S. Lakshminarayana. 1985. Compositional changes in mango fruit during ripening at different storage temperatures. J. Food Sci. 50, 1646-1648. [ Links ]
Valdenegro, M., S. Almonacid, C. Henríquez, M. Lutz, L. Fuentes, and R. Simpson. 2013. The effects of drying processes on organoleptic characteristics and the health quality of food ingredients obtained from goldenberry fruits (Physalis peruviana). Open Access Scientific Reports 2, 1-7. [ Links ]
Vega-Gálvez, A., K. Di Scala, K. Rodríguez, R. Lemus-Mondaca, M. Miranda, J. López and M. Perez-Won. 2009. Effect of air-drying temperature on physico-chemical properties, antioxidant capacity, colour and total phenolic content of red pepper (Capsicum annuum L. var. Hungarian). Food Chem. 117, 647-653. [ Links ]
Velioglu, Y.S., G. Mazza, L. Gao, and B.D. Oomah. 1998. Antioxidant activity and total phenolics in selected fruits, vegetables, and grain Products. J. Agric. Food Chem. 46, 4113-4117. [ Links ]
Wall, M.M. 2006. Ascorbic acid, vitamin A, and mineral composition of banana (Musa sp.) and papaya (Carica papaya) cultivars grown in Hawaii. J. Food Comp. Anal. 19, 434-445. [ Links ]