Services on Demand
Journal
Article
Indicators
-
Cited by SciELO
-
Access statistics
Related links
-
Cited by Google
-
Similars in SciELO
-
Similars in Google
Share
Agronomía Colombiana
Print version ISSN 0120-9965
Agron. colomb. vol.32 no.3 Bogotá Sept/Dec. 2014
https://doi.org/10.15446/agron.colomb.v32n3.45477
Doi: 10.15446/agron.colomb.v32n3.45477
1 La Libertad Research Center, Corporacion Colombiana de Investigacion Agropecuaria (Corpoica). Villavicencio (Colombia). jhbernal@corpoica.org.co
2 Department of Agronomy, Faculty of Agricultural Sciences, Universidad Nacional de Colombia. Bogota (Colombia)
Received for publication: 6 September, 2014. Accepted for publication: 27 November, 2014.
ABSTRACT
Sugar production in sweet sorghums is affected by the environment. Therefore, in this study on the effects of the genotype x environment interaction on sugar accumulation, plant traits associated with the sugar content in the stem were evaluated in ten sorghum genotypes grown in six contrasting environments. The results indicated that the stem dry weight, juice sugar concentration (°Brix), stem sugar content and juice volume were controlled by the genetic constitution of the genotype, with a large environmental contribution to their expression. The results allowed for the identification of the sweet sorghum genotypes that have a high potential for the biofuel agroindustry due to their high sugar contents in the environmental conditions of Palmira, Espinal, Cerete and Codazzi. Humid tropical environments such as Gaitan and Villavicencio were less favorable for the competitive production of sweet sorghums for bioethanol due to their low levels of solar radiation and soil fertility.
Key words: Poaceae, distribution of assimilates, biofuels, energy crops, lowland tropics.
RESUMEN
La producción de azucares en el cultivo de sorgo dulce es afectada por el por ambiente. Por tanto, en este estudio sobre el efecto de la interacción genotipo x ambiente en la acumulación de azucares, se evaluaron características de la planta asociadas con el contenido de azucares en el tallo en diez genotipos de sorgo cultivados en seis ambientes contrastantes. Los resultados indicaron que el peso seco del tallo, la concentración de azucares en el jugo (°Brix), el contenido de azucares en el tallo y el volumen de jugo eran controladas por la constitución genética de los genotipos con un gran aporte ambiental para su expresión. Los resultados permitieron identificar genotipos de sorgo dulce con gran potencial para la agroindustria de biocombustibles por sus altos contenidos de azucares en los ambientes de Palmira, Espinal, Cereté y Codazzi en relación con los ambientes de trópico húmedo con menor radiación solar y suelos de baja fertilidad como Gaitán y Villavicencio que fueron ambientes menos favorables para la producción competitiva de sorgo dulce para bioetanol.
Palabras clave: Poaceae, distribucion de asimilados, biocombustibles, cultivos energeticos, tropico bajo.
Introduction
Sweet sorghum (Sorghum bicolor [L.] Moench) is a C4 grass with high radiation and water use efficiency, high biomass and sugar yields, low water and nitrogen requirements, wide variability, and tolerance to droughts and salinity (Evans and Cohen, 2009). Sweet sorghum stems have high contents of soluble sugars, cellulose and hemicellulose (Antonopoulou et al., 2008). As a consequence, sweet sorghums could be a viable option for the environmentally sustainable production of first generation biofuels (De Vries et al., 2010).
The genetic improvement of sweet sorghum crops has mainly focused on developing new genotypes that have high sugar yields in order provide raw materials for the biofuels agroindustry. Different studies have reported a high positive correlation between sugar yields and Brix values, sugar content, stem production and stem moisture; and between stem production and plant height, stem diameter and juice yield (Shinde et al., 2012; Gutjhar et al., 2013).
Several studies have been carried out to characterize the interaction pattern of sugar accumulation and its components between sweet sorghum genotypes and the environment (Makanda et al., 2012; Elangovan et al., 2014). The results confirmed the presence of a significant interaction between the genotype and the environment (GE) as a consequence of the differential response of the genotypes to environmental changes. In all of the cases, the variation that resulted from the environment had the largest contribution to the total observed variation in the sugar contents (De Vries et al., 2010).
In sweet sorghums, the sugar content and the stem and juice yields are quantitative traits with polygenic inheritance, highly affected by the environment (Zou et al., 2011; Elangovan et al., 2014). There is great variation in the sugar content in the stems of sorghum varieties, e.g., °Brix in the juice extracted from the stem of 200 cultivars ranged from 8.0% to 19.1% (Zhao et al., 2008). This variation is even more complex because not all of the genotypes responded to environmental changes in the same way (Elangovan et al., 2014).
This study was conducted to estimate the effect of the genotype x environment interaction on sugar accumulation in improved varieties of sweet sorghum and to identify genotypes with a broad or specific adaptation to environmental conditions, such as the rainfall, average temperature, radiation and soil conditions of Colombia's lowland tropics.
Materials and methods
Genetic material and experimental locations
Nine improved open-pollinated sweet sorghum genotypes from Cirad and ICRISAT were evaluated along with a commercial variety, JJT18, across six locations (environments) during the rainy seasons of 2009 and 2010 (Tab. 1). Table 2 describes the geographical location and environmental characteristics of the experimental sites. The agronomic management practices were carried out by taking into consideration the environmental conditions of each location and the crop requirements.
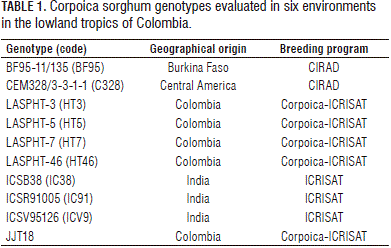
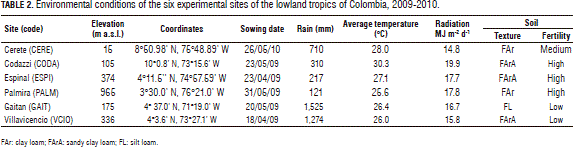
Phenological phases and thermal time
The crop cycle was separated into three phenological stages: vegetative (GS1), reproductive (GS2) and grain filling (GS3). Five plants per plot were randomly selected to determine the thermal time required for each genotype to achieve each phase in the six localities. The thermal time calculation was done with the vertical single-sine wave method proposed by Roltsch et al. (1999). Three cardinal temperatures were considered for its calculation: a base of 11°C, optimal between 30 to 34°C and maximum from 42°C (Lafarge and Tardieu, 2002).
Plant measurements of traits related to sugar yields
The stem yields were determined on the basis of stems harvested from two central rows at physiological maturity, between 106 and 115 d after emergence. The dry weight was estimated from a subsample after drying for a 72-h period in an oven at 70°C. The plant height and juice stem volume were measured at maturity from five randomlyselected plants from each plot. The °Brix measurements were performed with a hand-held PAL-1 refractometer (Atago USA Inc., Bellevue, WA) on the juice of five stems extracted after stem crushing in a three roll press. The estimated sugar content (g/stem) was calculated as shown in Eq. 1 (Gutjahret al., 2013).
where, 8.827 is the slope of the regression line between the °Brix and sugar concentration of sugars in g L-1 of juice.
Experimental design and statistical analysis
In each location, the genotypes were tested in a completely randomized block design with four replicates. Each experimental plot had six 5.0 m-long rows, with 0.7 m spacing between the rows and 0.10 m between the plants. All statistical analyses were performed using SAS® v9.0 (SAS Institute, Cary, NC). In terms of effects, the basic model for a multi-environmental trial is illustrated in Eq. 2.
where,
Yijk = is the kth observation of the ith genotype in the jth environment
m = is the overall mean
Gi = is the main effect of the ith genotype (considered fixed)
Ej = is the main effect of the jth environment (considered random)
B(E)k(j) = is the effect of the kth block within the jth environment
GE(ij) = is an interaction effect associated with genotype i and environment j
eijk = is the error associated with each measurement
The effect of the genotype and environment interaction was analyzed with the AMMI model (additive main effects and multiplicative interaction model), shown in Eq. 3. For the SAS analysis, a computational algorithm created by Vargas et al. (2001) was used.
where,
Yge = is the average yield of genotype g in environment e
m = is the overall mean
ag = is the genotype mean deviations
be = is the environment mean deviations
N = is the number of principal components retained in the model
ln = is the singular value for the principal component
ggn = is the eigenvector score for genotype g and component n
den = is the eigenvector score for environment e and component n
rge = is the residual for genotype g and environment e
Results
The overall picture of the relative magnitude of the genotype (G), environment (E) and G × E (GE) interactions is presented in Tab. 3 in the form of ANOVA. The analysis of variance clearly showed that the G, E and GE effects were significant (P≤0.01) for all of the traits. Table 3 also depicts the relative contribution of each source to the total variation (G + E + GE). It was observed that the environment was the most important source of variation for most of the traits, except for plant height. The contribution of the genotype was 71.9% of the plant height variation. For the thermal time, the environment accounted for 42.6, 28.6 and 71.0% of the variation in GS1, GS2 and GS3, respectively.
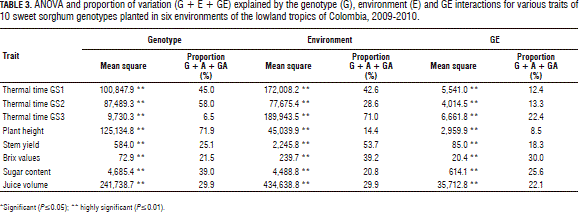
For the stem yield, the genotype explained 25.1%, while the environment accounted for 53.7% of the total variation. For the Brix values, sugar content and juice volume, the genotype explained 21.5, 39.0, and 29.9% of the total variation, respectively, while the environment accounted for 39.2, 20.8 and 29.9%, respectively (Tab. 3).
Genotype x environment interaction effects on plant growth and development
The performance and stability of the genotypes were visualized graphically with AMMI biplot (Fig. 1). For the thermal time accumulated in growth stages GS1, GS2 and GS3, the first two principal components (PC) explained 74.3, 79.9 and 97.0% of the GE variation, respectively. Genotypes HT3 and IC38 were relatively stable for the growing degree days accumulated during GS1 and GS2, owing to their proximity to the origin. The environments that accounted for most of the total variation of the interaction were Cerete and Codazzi for GS1 and GS2 and Cerete and Espinal for GS3. The environment in Codazzi caused a greater duration of the GS1 phase (665 degree-days, °Cd) and GS2 phase (601°Cd) in most of the genotypes, while the environment in Palmira caused a reduction in the thermal time for the completion of these two growth phases, 402 and 422°Cd, respectively. The environments in Codazzi and Espinal were responsible for the long duration of the GS1 and GS2 growth periods observed in IC91 (Fig. 1).
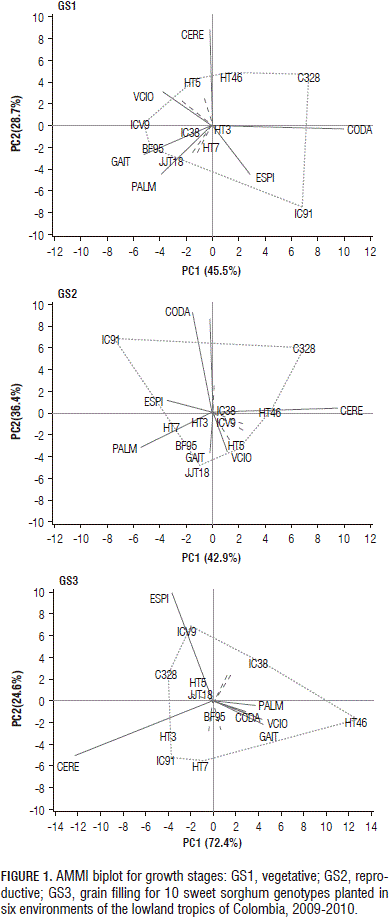
The sweet sorghum genotypes developed by Corpoica-ICRISAT's breeding program showed longer growth phases, as compared with the varieties from foreign countries. Genotypes HT7 (1,623°Cd), HT46 (1,691°Cd) and JJT18 (1,733°Cd) were largely distinguished from the others.
For plant height, the first two principal components explained 71.4% of the GE variation (Fig. 2). Genotypes BF95, HT3, HT5, HT7, JJT18 and ICV9 were relatively stable for plant height, owing to their proximity to the origin. According to the biplot in Fig. 2, the vertex genotypes were C328, HT46, IC91 and IC38. These genotypes performed better in the environment, falling within the sectors. C328 had the highest plant height in Cerete (341 cm) and Codazzi (333 cm), respectively. In addition, IC91 showed the greatest plant height in Cerete (336 cm), whereas IC38 had the highest plant height in Gaitan (235 cm).
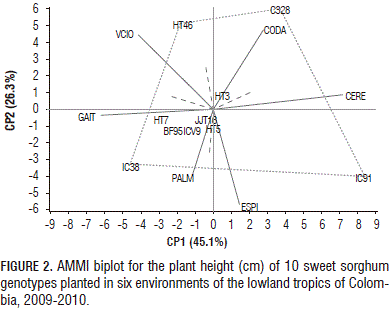
Sweet sorghum genotypes, HT3, HT5, HT7, HT46 and JJT18, developed by Corpoica-ICRISAT's breeding program, showed the greatest plant height. The plant height of HT7 ranked first in Cerete (413 cm), Gaitan (389 cm) and Villavicencio (406 cm). Cerete and Codazzi seemed to have favorable environments for plant height in almost all of the genotypes, while Espinal had a less favorable environment.
Stem yield
For the stem yield, the first two principal components explained 89.6% of the GE variation (Fig. 3). Genotypes HT3, HT5, HT7 were relatively stable for stem yield, owing to their proximity to the origin. HT46, C328, JJT18, HT7 and BF95 were placed far from the origin and exhibited larger interactions. In Cerete, the higher stem yields were recorded for JJT18 (47.1 t ha-1), HT5 (40.2 t ha-1), HT3 (37.0 t ha-1), HT7 (36.4 t ha-1) and C328 (24.3 t ha-1). In the present study, the higher stem yields were achieved by the sweet sorghum genotypes HT3 (16.3 t ha-1), HT5 (17.1 t ha-1), HT7 (18.1 t ha-1), HT46 (16.3 t ha-1) and JJT18 (19.1 t ha-1).
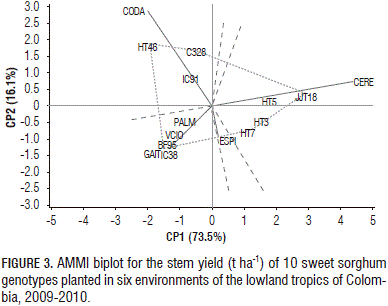
Cerete and Codazzi seemed to be favorable environments for stem yield in C328 and IC91. The environmental conditions of Codazzi and Cerete allowed most of the genotypes to achieve a high stem yield while the lower stem productions were recorded in Gaitan (7.5 t ha-1) and Villavicencio (8.3 t ha-1). The genotypes HT7 (11.9 t ha-1) and HT46 (10.9 t ha-1) were distinguished by their high stem yields in the less favorable environment of Gaitan.
Sugar accumulation and stem juice volume
In AMMI, the first two principal components explained 74.4% of the GE variation for the Brix values, 87.4% for the sugar content and 85.0% for the juice volume (Fig. 4).
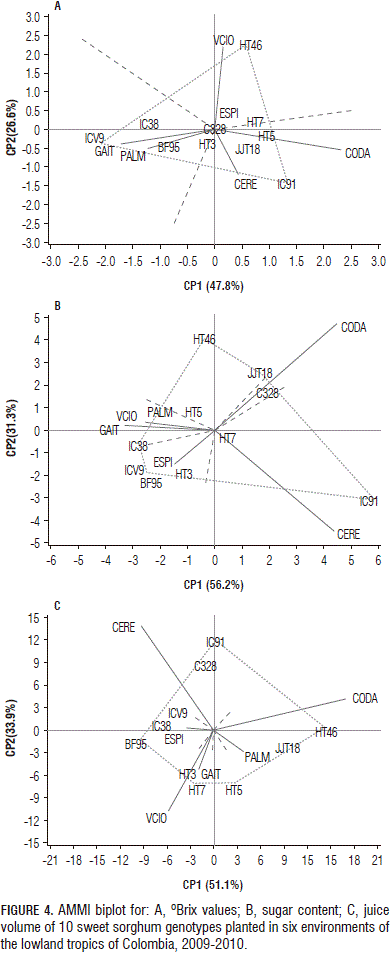
The genotypes C328, HT3, HT5, HT7 and JJT18 were relatively stable for °Brix values, owing to their proximity to the origin. The polygon formed by connecting the markers of the genotypes that were farther away from the biplot origin indicated that the best genotype in terms of the oBrix values in the environment was found in the sectors (Fig. 4). HT46 registered the highest °Brix values (21.3) for Villavicencio and Espinal (19.0) and IC91 reported higher values in Codazzi (16.9). On average, the genotypes with a higher juice sugar concentration were C328 (16.0), HT3 (16.0), HT46 (16.0) and JJT18 (16.0). Espinal, Palmira and Gaitan showed favorable environments for the juice sugar concentration in the stem of almost all of the genotypes, whereas the environments of Cerete and Codazzi were less favorable.
For the stem sugar content, HT5 and HT7 were relatively stable, whereas HT46, JJT18, ICV9, IC91, and IC38 showed greater variation (Fig. 4). HT46 and JJT18 registered greater values of sugar content in Codazzi (80.8 and 85.5 g, respectively), while genotype IC91 showed higher values in Cerete (88.8 g), along with C328 in Codazzi (59.6 g). On average, a higher stem sugar content was found in HT7 (47.7 g), HT46 (51.8 g), IC91 (43.3 g) and JJT18 (51.3 g).
Codazzi, Palmira and Cerete had environments in which the sweet sorghum genotypes showed higher average values for the stem sugar content, 47.6, 44.8 and 39.6 g, respectively, as compared to the lower values reported for Villavicencio, Gaitan and Espinal, 29.6, 28.3 and 20.5 g, respectively. Codazzi and Cerete had the biggest contribution to the total variation in the stem sugar content observed in the GE interaction. In these environments, the genotypes showed large variations in the sugar content and these environments could be considered as ones that allow for better discrimination of sweet sorghum genotypes through their ability to accumulate sugars.
IC91, HT46, HT5, HT7 and BF95 showed a greater variation in the stem juice volume between the environments; whereas IC38 and ICV9 were relatively stable (Fig. 4). In Codazzi, HT46 (793 mL) and JJT18 (797 mL) showed high juice volumes, while HT3 (477 mL) and HT7 (497 mL) registered high stem juice volumes in Villavicencio. On average, the genotypes with greater juice volumes were HT5 (356 mL), HT7 (382 mL), HT46 (342 mL) and JJT18 (465 mL). The Codazzi, Cerete and Villavicencio environments had the biggest contribution to the total variation in the stem juice volume observed in the GE interaction (Fig. 4). Codazzi, Palmira and Villavicencio provided environments in which the sweet sorghum genotypes showed higher average values for the stem juice volume, 463, 338 and 336 mL, respectively.
Discussion
Phenological development
The variation in the thermal time was explained by the genotype, environment and GE interactions. HT3 and HT7 were relatively stable in terms of the thermal time required to end the growing periods GS1 and GS2. The sweet sorghum genotypes HT3, HT5, HT7, HT46 and JJT18 showed a greater accumulation of growing degree days for each growing period in almost all of the environments, as compared to the foreign genotypes.
The severe water stress observed during growing periods GS1 and GS2 in the genotypes of some of the environments probably affected the predictions of the phenology model, which was based on degree-days. Donatelli et al. (1992) reported a reduction in life cycle in sorghum genotypes subjected to water stress during the reproductive phase. McMaster et al. (2005) validated the concept of thermal time for a wide range of conditions, but suggested the introduction of a genotype response to water stress in the model.
The higher accumulation of thermal time seen in C328 during growing period GS1 in Cerete and Codazzi was probably due to a photoperiod response. The day lengths registered during GS1 in Cerete (12.6 h) and Codazzi (12.7 h) were relatively longer than those reported in Espinal (12.3 h), Palmira (12.3 h), Gaitan (12.3 h) and Villavicencio (12.2 h). In tropical conditions, Miller (1968) reported photoperiod responses in minutes for sorghum varieties.
Plant height
The genotype plant heights were affected by the genetic constitution, with a minor environmental contribution. The lower environment effect on the total height variation seen among the genotypes would suggest that this characteristic is controlled by a few genes. An earlier genetic study found four loci that affect sorghum height: Dw1, Dw2, Dw3 and Dw4 (Quinby and Karper, 1954). Genetic linkage mapping studies have shown that sorghum height is controlled by only a few major QTLs (Salas Fernandez et al., 2009) that have high heritability (0.83-0.95; Murray et al., 2008; Ritter et al., 2008). Therefore, many studies have focused on height as an indirect way to identify the genes related to biomass yield (Salas Fernandez et al., 2009).
A greater plant height was found in the sweet sorghum varieties developed by Colombia's breeding program, which also showed a longer duration in growing periods GS1 and GS2. The favorable conditions of the soil fertility in Cerete and Codazzi combined with an optimal climatic water balance during GS1 and GS2 allowed most of the genotypes to allocate more assimilates to the formation and elongation of internodes, which caused a superior plant height. C328 and IC91 had a favorable environment in Codazzi and Cerete for a longer duration of GS1 and GS2, which ultimately led to the expression of a greater plant height.
Massacci et al. (1996) reported that water stress in sweet sorghums leads to a reduction in the internode elongation as a result of a decrease in the enzyme activities responsible for sugar degradation, which in turn stimulates stem sucrose accumulation.
Stem yields
The environment was responsible for 53.7% of the total variation in the stem yield, with a minor contribution from the genotype. Codazzi and Cerete seemed to be favorable environments for a greater stem dry matter accumulation in almost all of the sorghum genotypes. Sweet sorghum genotypes HT5, HT7 and JJT18, which are adapted to Colombia's tropical lowlands, were outstanding, with greater stalk yields. In Cerete, genotypes C328 and IC91 found a favorable environment for stem growth and yield.
In Cerete and Codazzi, most of the genotypes had a longer growing period for GS1 and GS2 and favorable soil fertility and rainfall during the crop growth cycle, which contributed to the formation of large sinks, stems, and fully expanded leaves, which provided sufficient photoassimilates to meet the sink demands.
Gutjahr et al. (2013) found higher biomass yields and sugar accumulation in sorghum genotypes that had a long duration to flowering. Alhajturki et al. (2012) reported decreased carbohydrate stem accumulation in sweet sorghum varieties growing during the dry season. In sweet sorghum varieties, temporal waterlogging during the vegetative and early reproductive stages caused reductions in biomass production, leaf area, photosynthetic rate, stomatal conductance and transpiration (Promkhambut et al., 2010).
Sugar accumulation and stem juice volume
The results clearly showed that the environment caused the most variation in the stem sugar concentration (°Brix), stem sugar content and juice volume across the sorghum genotypes, indicating that these traits are under quantitative polygenic inheritance. Murray et al. (2008) indicated that the sugar concentration in sweet sorghum is affected by many genes (is subject to polygenic control) and by gene-environment interactions. Lv et al. (2013) reported that the stalk juice weight was a quantitative trait with more than one QTL responsible for this trait.
In Espinal and Palmira, the reduction in the stem growth as a result of water stress and lower temperatures during GS1 and GS2 was responsible for the higher stem sugar concentration of the genotypes. Similar results were reported by Makanda et al. (2012) in sorghum hybrids evaluated in four environments. High overall means for stem Brix were attributed to temperatures of between 25 to 30°C, recorded in the favorable environments, while environments with maximum temperatures of 30 to 45°C resulted in lower sugar concentrations. Meanwhile, Alhajturki et al. (2012) suggested that the accumulation of sugar in sweet sorghum under water stress was an osmotic adjustment mechanism that allowed the plant to maintain water absorption for higher rates of photosynthesis and plant growth.
On average, higher values of stem sugar content in most of the genotypes were registered in Cerete, Codazzi and Palmira. The sugar content in stems incorporates two major components for sugar production in sweet sorghum: juice sugar concentration (°Brix) and the stem size available for sugar accumulation. The soils with better fertility, along with mean temperatures and water availability that were suitable for crop growth, found in Cerete and Codazzi allowed genotypes C328, HT3, HT7, HT46, IC91 and JJT18 to achieve a high stem sugar content.
HT5, HT7 and JJT18 showed higher juice volumes, while C328 and ICV9 registered a lower juice volume. C328 achieved the highest juice volume in Codazzi. The genotypes in Codazzi, Palmira and Villavicencio were distinguished by presenting higher values in juice volume, while Espinal had the lowest.
Gutjhar et al. (2013) reported that a high variability in stem biomass did not allow the finding of a correlation between stem sugar content and juice sugar concentration in sorghum genotypes. The sugar yield was more dependent on the stem weight and the juice volume rather than on the sugar concentration, which was high in most of the genotypes. The principal drivers for dry matter accumulation, the genetic constitution and the environment were responsible for stem sugar storage.
Conclusions
The sweet sorghum genotypes with a greater sugar yield potential showed a longer growth cycle period, taller plants, juice stems and a greater sugar accumulation. A tradeoff between stem yield and stem sugar concentration (°Brix) was observed across the sorghum genotypes. The environments that resulted in a greater stem yield among the genotypes reduced the stem sugar concentration. The results allowed for the identification of the sweet sorghum genotypes that have a high potential for the biofuel agroindustry, due to their high sugar yields, in Palmira, Espinal, Cerete and Codazzi. On the other hand, the humid tropical environments with less solar radiation and low fertility soils, such as Gaitan and Villavicencio, had less favorable environments for competitive bioethanol production.
Literature cited
Alhajturki, D., M. Aljamali, A. Kanbar, and F. Azmah. 2012. Potential of some sweet sorghum (Sorghum bicolor L.) genotypes under two water regimes for sugar and bio-ethanol production. Sugar Tech 14, 376-382. Doi: 10.1007/s12355-012-0181-x [ Links ]
Antonopoulou, G., H.N. Gavala, I.V. Skiadas, K. Angelopoulos, and G. Lyberatos. 2008. Biofuels generation from sweet sorghum fermentative hydrogen production and anaerobic digestion of the remaining biomass. Bioresour. Technol. 99, 110-119. Doi: 10.1016/j.biortech.2006.11.048 [ Links ]
De Vries, S.C., G.W.J. Van de Ven, M.K. Van Ittersum, and K.E. Giller. 2010. Resource use efficiency and environmental performance of nine major biofuel crops, processed by firstgeneration conversion techniques. Biomass Bioenerg. 34, 588-601. Doi: 10.1016/j.biombioe.2010.01.001 [ Links ]
Donatelli, M., G.L. Hammer, and R.L. Vanderlip. 1992. Genotype and water limitation effects on phenology, growth, and transpiration efficiency in grain sorghum. Crop Sci. 32, 781-786. Doi: 10.2135/cropsci1992.0011183X003200030041x [ Links ]
Elangovan, M., P. Kiran Babu, N. Seetharama, and J.V. Patil. 2014. Genetic diversity and heritability characters associated in sweet sorghum [Sorghum bicolor (L.) Moench]. Sugar Tech 16, 200-2010. Doi: 10.1007/s12355-013-0262-5 [ Links ]
Evans, J.M. and M.J. Cohen. 2009. Regional water resource implications of bioethenol production in the Southeastern United States. Glob. Change Biol. 15, 2261-2273. Doi: 10.1111/j.1365-2486.2009.01868.x [ Links ]
Gutjahr, S., M. Vaksmann, M. Dingkuhn, K. Thera, G. Trouche, S. Braconnier, and D. Luquet. 2013. Grain, sugar and biomass accumulation in tropical sorghums. I. Trade-offs and effects of phenological plasticity. Funct. Plant Biol. 40, 342-354. Doi: 10.1071/FP12269 [ Links ]
Lafarge, T. and F. Tardieu. 2002. A model co-ordinating the elongation of all leaves of a sorghum cultivar was applied to both Mediterranean and Sahelian conditions. J. Exp. Bot. 53, 715-725. Doi: 10.1093/jexbot/53.369.715 [ Links ]
Lv, P., G. Ji, Y. Han, S. Hou, S. Li, X. Ma, R. Du, and G. Liu. 2013. Association analysis of sugar yield-related traits in sorghum [Sorghum bicolor (L.)]. Euphytica 193, 419-431. Doi: 10.1007/s10681-013-0962-7 [ Links ]
Makanda, I., J. Derera, P. Tongoona, and J. Sibiya. 2012. Genetic and GGE biplot analyses of sorghum germplasm for stem sugar traits in Southern Africa. Afr. J. Agric. Res. 7, 212-223. Doi: 10.5897/AJAR11.989 [ Links ]
Massacci, A., A. Battistelli, and F. Loreto. 1996. Effect of drought stress on photosynthetic characteristics, growth and sugar accumulation of field-grown sweet sorghum. Funct. Plant Biol. 23, 331-340. Doi: 10.1071/PP9960331 [ Links ]
McMaster, G.S., W.W. Wilhelm, and A.B. Frank. 2005. Developmental sequences for simulating crop phenology for waterlimiting conditions. Aust. J. Agric. Res. 56, 1277-1288. Doi: 10.1071/AR05068 [ Links ]
Miller, F.R., D.K. Barnes, and H.J. Cruzado. 1968. Effect of tropical photoperiods on the growth of sorghum when grown in 12 monthly plantings. Crop Sci. 8, 499-502. Doi: 10.2135/cropsci1968.0011183X000800040032x [ Links ]
Murray, S.C., A. Sharma, W.L. Rooney, P.E. Klein, J.E. Mullet, S.E. Mitchell, and S. Kresovich. 2008. Genetic improvement of sorghum as a biofuel feedstock: I. QTL for stem sugar and grain nonstructural carbohydrates. Crop Sci. 48, 2165-2179. Doi: 10.2135/cropsci2008.01.0016 [ Links ]
Promkhambut, A., A. Younger, A. Polthanee, and C. Akkasaeng. 2010. Morphological and physiological responses of sorghum (Sorghum bicolor L. Moench) to waterlogging. Asian J. Plant Sci. 9, 183-193. Doi: 10.3923/ajps.2010.183.193 [ Links ]
Quinby, J.R. and R.E. Karper. 1954. Inheritance of height in sorghum. Agron. J. 46, 211-216. Doi: 10.2134/agronj1954.00021962004600050007x [ Links ]
Ritter, K.B., D.R. Jordan, S.C. Chapman, I.D. Godwin, E.S. Mace, and C.L. McIntyre. 2008. Identification of QTL for sugar-related traits in a sweet × grain sorghum (Sorghum bicolor L. Moench) recombinant inbred population. Mol. Breed. 22, 367-384. Doi: 10.1007/s11032-008-9182-6 [ Links ]
Roltsch, W.J., F.G. Zalom, A.J. Strawn, J.F. Strand, and M.J. Pitcairn. 1999. Evaluation of several degree-day estimation methods in California climates. Int. J. Biometeorol. 42, 169-176. Doi: 10.1007/s004840050101 [ Links ]
Salas Fernandez, M.G., P.W. Becraft, Y. Yin, and T. Lubberstedt. 2009. From dwarves to giants? Plant height manipulation for biomass yield. Trends Plant Sci. 14, 454-461. Doi: 10.1016/j.tplants.2009.06.005 [ Links ]
Shinde, D.A., V.A. Lodam, S.S. Patil, and B.D. Jadhav. 2012. Character association in sweet sorghum [Sorghum bicolor (L.) Moench]. Agric. Sci. Digest. 32, 48-51. [ Links ]
Vargas, M., J. Crossa, F. Van Eeuwijk, K.D. Sayre, and M.P. Reynolds. 2001. Interpreting treatment × environment interaction in agronomy trials. Agron. J. 93, 949-960. Doi: 10.2134/agronj2001.934949x [ Links ]
Zhao, X.N., G.Y. Li, Y. Liu, P. Lu, B.Q. Dun, M.Q. Yue, and P. Zang. 2008. Genetic diversity and correlation analysis of main agronomic characters in domestic and foreign sweet sorghum germplasm. J. Plant Genet. Resour. 9, 302-307. [ Links ]
Zou, G., S. Yan, G. Zhai, Z. Zhang, J. Zou, and Y. Tao. 2011. Genetic variability and correlation of stalk yield-related traits and sugar concentration of stalk juice in a sweet sorghum (Sorghum bicolor L. Moench) population. Aust. J. Crop Sci. 5, 1232-1238. [ Links ]