Serviços Personalizados
Journal
Artigo
Indicadores
-
Citado por SciELO
-
Acessos
Links relacionados
-
Citado por Google
-
Similares em SciELO
-
Similares em Google
Compartilhar
Agronomía Colombiana
versão impressa ISSN 0120-9965
Agron. colomb. vol.32 no.3 Bogotá set./dez. 2014
https://doi.org/10.15446/agron.colomb.v32n3.45941
Doi: 10.15446/agron.colomb.v32n3.45941
1 Agricultural Research Group, Faculty of Agricultural Sciences, Universidad Pedagogica y Tecnologica de Colombia. Tunja (Colombia). jgalvarezh@gmail.com
Received for publication: 2 October, 2014. Accepted for publication: 27 November, 2014.
ABSTRACT
The growth and development of cape gooseberry plants obtained from cuttings is affected by microorganisms in the substrate and by the concentration of plant growth regulators applied during planting. Using a factorial design, the effect of four concentrations of indole-3-butyric acid (IBA) (0, 800, 1,200, and 1,600 mg L-1) and four suspensions of Trichoderma harzianum (0, 2·106, 3·106, and 4·106 cfu/mL) on the growth of cape gooseberry cuttings was evaluated. Apical cuttings were used, 20 cm long, from 20-month-old plants of the Colombian ecotype, which were planted in pots (2 L) using peat moss with burned rice husks as the substrate at a 3:1 ratio (v/v). The 800 mg L-1 of IBA application showed the highest accumulation of total dry mass (DM) and root DM. Moreover, 800 mg L-1 of IBA resulted in the highest leaf chlorophyll content, showing significant differences from 1,600 mg L-1, which had the lowest value. The 3·106 cfu/mL suspension of T. harzianum caused a greater accumulation of root DM and total DM and a higher leaf area in the plants. The most favorable interaction between the factors, which favors the growth of cape gooseberry plants obtained from cuttings, was observed with the application of 800 mg L-1 of IBA and 3·106 cfu/mL of T. harzianum.
Key words: plant growth regulators, auxin, biocontrol, cuttings, growth.
RESUMEN
El crecimiento y desarrollo de los esquejes de uchuva es afectado por los microorganismos presentes en el sustrato y por la concentración de hormonas aplicada al momento de la siembra. Mediante un diseño factorial se evaluó el efecto de cuatro concentraciones de acido indol-3-butirico (AIB) (0, 800, 1.200 y 1.600 mg L-1) y cuatro suspensiones de Trichoderma harzianum (0, 2・106, 3・106 y 4・106 ufc/mL) en el crecimiento de esquejes de uchuva. Se usaron esquejes apicales de 20 cm de largo obtenidos de plantas de 20 meses de edad, ecotipo Colombia, los cuales fueron sembradas en materas de 2 L en un sustrato de turba rubia y cascarilla de arroz quemada en relación 3:1 en volumen. Al aplicar 800 mg L-1 de AIB las plantas presentaron la mayor acumulación de masa seca (MS) total y MS de raíces. Además, la concentración de 800 mg L-1 de AIB presento el mayor contenido foliar de clorofila mostrando diferencias significativas con la dosis de 1.600 mg L-1, la cual tuvo el menor valor. La suspensión de 3・106 ufc/mL de T. harzianumprovoco una mayor acumulación de MS de raíces, MS total y área foliar de la planta. La interacción entre factores que más favoreció el crecimiento de las plantas de uchuva, provenientes de esquejes, se dio cuando se aplicaron 800 mg L-1 AIB y 3・106 cfu/mL de T. harzianum.
Palabras clave: fitoreguladores, auxina, biocontrol, estaca, crecimiento.
Introduction
The cape gooseberry (Physalis peruviana L.) is a native plant from the South American Andes. This plant is the best known species of the Physalis genus and is characterized by a sweet fruit (highest Brix grades of 17.2) that contains a high content of vitamins A and C, iron and phosphorus (Galvis et al., 2005; Fischer et al., 2011). This fruit, along with mango, passion fruit, pitahaya, tree tomato, and baby banana, is part of the promising exportable fruits from Colombia, of which cape gooseberry ranks first (Fischer et al., 2007).
The propagation of this plant is mostly done by seed and, to a lesser extent, asexually (López et al., 2008), but the cape gooseberry also responds well to asexual propagation through cuttings (Fischer et al., 2014). Asexual propagation ensures a greater uniformity of the crop and the same genetic characteristics as the parent plant (Hartmann et al., 2010), which is practical for the management of cultures of identical plants.
In some cases, propagation through cuttings is successful and the plant material does not need further treatment to generate a whole plant, but in others, this type of propagation is unsuccessful due to environmental conditions or intrinsic factors of the plant and it becomes necessary to subject the cuttings to different treatments, such as the application of plant growth regulators (PGR), microorganisms and the use of certain substrates in order to generate a new vigorous plant (Moreno et al., 2009). In this regard, López et al. (2008) found that cape gooseberry cuttings selected from the top of the reproductive shoot showed better results in rooting than cuttings taken from the middle or lower position of the shoot. Miranda and Perea (2012) explained that the better rooting of apical shoot cuttings is due to the higher concentration of endogenous promoting substances that originate in apical buds; also, younger cuttings have more cells that are capable of becoming meristematic.
The application of auxin to induce the rooting of cuttings has been reported by several authors (Ramírez-Villalobos et al., 2004; Miranda, 2005; Roh et al., 2005; Moreno et al., 2009; Ruiz-Solsol and Mesén, 2010), who showed that these plant growth regulators are essential for rooting in different plant species. Moreno et al. (2009) used indole-3-butyric acid (IBA) at concentrations of 200 to 800 mg L-1 to induce cape gooseberry rooting and found that the rooting was directly proportional to the increase in the IBA concentration, although the authors did not establish at what concentration the growth curve had a slope that was equal to zero or negative, meaning that the auxin concentrations that increased or reduced the growth were not determined. In general, IBA is applied to the base of cuttings; however, Pacholczak et al. (2012) found that, in Cotinus coggygria, a greater effect on rooting was seen using 200 mg dm-3 of foliar IBA, as compared to dipping the base of the stems in the hormone solution.
The fungus Trichoderma harzianum has been used as a biological control for diseases; for example, in onions, the substrate application of T. harzianum reduced infection by Fusarium oxysporumand caused an increase in the bulb diameter (Coskuntuna and Özer, 2008). It is also widely used in the control of postharvest diseases ever since Batta (2007) established that the application of an emulsion based on T. harzianum in fruits such as apple, strawberry, pear, peach, kiwi, and grape reduced the injury diameter caused by Rhizopus stolonifer, Botrytis cinerea, and Penicillium expansum and provided protection for up to two months against these diseases. Moreover, in addition to its antibiotic properties and fungal antagonism, T. harzianum also promotes the growth of plants. Apart from the above mentioned benefits, Trichoderma sp. also generates a greater availability of nutrients, such as phosphorus and iron, in the soil and stimulates the production of growth regulators in plants (Hoyos-Carvajal et al., 2009), which may be advantageous for crops, such as passion fruit (Cubillos-Hinojosa et al., 2009). Therefore, in order to stimulate growth and generate more vigorous plants at transplanting, the present study aimed to determine the effect of the application of indole 3-butyric acid and T. harzianum on the development and growth of cape gooseberry cuttings.
Materials and methods
The experiment was carried out in a plastic greenhouse at the Universidad Pedagogica y Tecnologica de Colombia, Tunja (Boyaca, Colombia), which had an inside average temperature of 18°C and relative humidity of 75% and is located at 2,690 m a.s.l.
Cuttings, 20 cm in length and with a pair of leaves, were taken from the top of the shoots of 20-month-old cape gooseberry plants, ecotype Colombia, because this type of cuttings presented better rooting (López et al., 2008). The cuttings were immersed in different auxin solutions for 5 min and then planted in 2 L pots filled with a Californian peat moss mixed with burned rice husks at a proportion of 3:1 (v/v). After planting, the individual suspensions of a commercial strain of T. harzianum were applied to the substrate.
A completely randomized 4x4 factorial design was used, where the first factor corresponded to the application of indole-3-butyric acid (IBA) (RADIX® 1500, Intercontinental Import Export, Mexico DF) at different concentrations (0, 800, 1,200, or 1,600 mg L-1) and the second factor to the application of T. harzianum at different suspensions (0, 2·106, 3·106, or 4·106 cfu/mL) for a total of 16 treatments. Each treatment had three replicates, resulting in 48 experimental units (EU), where each EU accounted for two cape gooseberry cuttings.
During the experiment, the substrate remained at field capacity and the plants were kept under a shade net (50% light reduction) during the first 50 d in order to avoid severe dehydration of the cuttings; furthermore, the flowers were removed in order to encourage vegetative growth. Beginning after 40 d of planting and for 4 months, a foliar fertilizer (g L-1) was applied weekly at a concentration of 200.00 of nitrogen (urea), 2.50 of MgO, 0.30 of B, 5.00 of S, 0.25 of Cu, 1.00 of Fe, 1.60 of Zn, 0.55 of Mn, and 0.03 Mo, which also contained 0.50 g L-1 naphthalene acetic acid (NAA).
After 110 d of the trial, the dry mass (DM) and fresh mass (FM) of the roots, stems, and leaves were measured with an electronic balance with 0.01 g accuracy (Acculab VIC 612, Bradford, MA), along with leaf area with a Li-Cor analyzer 3000 A (Licor, Lincoln, NE) and root length. Also, the leaf chlorophyll content was measured with a SPAD 502 Plus chlorophyll meter (Spectrum Technologies, Aurora, IL).
The results were subjected to the normality test of Kolmogorov-Smirnov and analysis of homogeneity of variance (Levene). Subsequently, an analysis of variance (ANOVA) was conducted to determine statistical differences between the treatments, followed by the multiple comparison Tukey test (P≤0.05). For the statistical analysis, SAS® v.8.1e (SAS Institute, Cary, NY) was used.
Results and discussion
Total leaf area
According to the ANOVA, for leaf area, there was no significant difference between the IBA levels. The average leaf area for the different IBA concentrations was 353.7 cm2 with a standard deviation of 45.8 cm2. Although no significant differences between the concentrations of IBA were found, the difference between the treatments with the lowest and highest leaf areas was over 100 cm2 (Tab. 1), which could result in greater light absorption and, therefore, more photosynthetic activity and growth (Taiz and Zeiger, 2010).
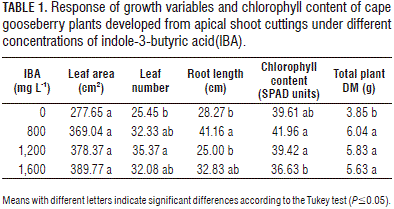
The relationship between the leaf area and IBA doses showed a polynomial behavior (Fig. 1), different from the findings of Moreno et al. (2009) who observed a lineal behavior. This result, which indicated that the auxin did not promote growth, may have been due to high concentrations of auxin (IAA), which stimulate the synthesis of abscicic acid (ABA), ethylene, and jasmonic acid, and, in certain concentrations, reduce plant growth (Hansen and Grossmann, 2000; Grossmann, 2010) or, in this case, nearly inhibit it. Therefore, under higher concentrations of 1,200 mg L-1 of IBA and more, the leaf growth of cape gooseberry cuttings may not increase.
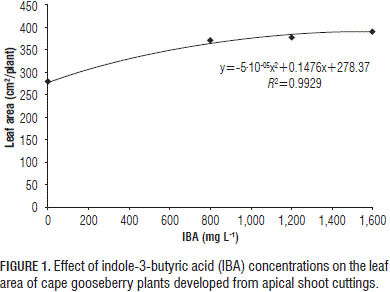
The plants with a higher leaf area were those that received a 3·106 cfu/mL suspension of T. harzianum, but significant differences were only found between this treatment and the 2·106 cfu/mL suspension (Tab. 2). No direct relation-ship between the leaf area and increased population of the fungus was observed, which indicates that this variable was not stimulated by the increase of the concentration of T. harzianum, but responded favorably to the 3·106 cfu/mL suspension.
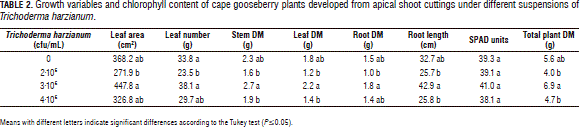
Similar results were reported by Donoso et al. (2008), who observed that the application of 2·106 cfu/mL of T. harzianumon cuttings promoted leaf DM accumulation in Pinus radiata; furthermore, Lo and Lin (2002) found that the application of a native strain of T. harzianum on Cucumis sativus plants increased plant growth and resulted in a higher leaf area (197%) and total DM (272%), as compared to the control treatment.
Fresh and dry mass of roots
The root FM and DM showed significant differences for the two evaluated factors and for their interaction. The plants treated with 800 mg L-1 and 1,600 mg L-1 of IBA developed the highest root FM (Fig. 2A). A similar DM behavior was observed, with a curve that peaked at 800 mg L-1. The interaction showed that the combination of 800 mg L-1 of IBA and 0 cfu/mL of T. harzianum was the best way to promote root growth (Tab. 3).
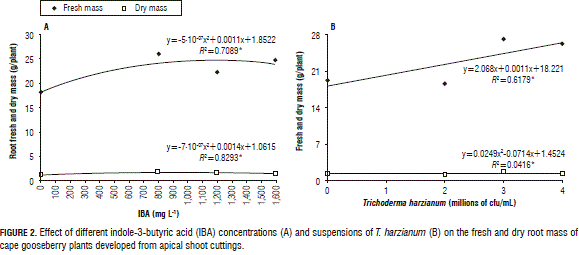
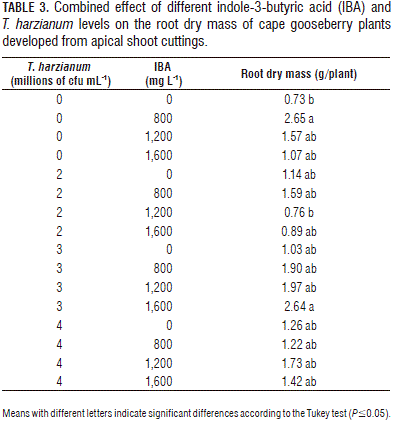
The mass increase of the roots with the 800 mg L-1 of IBA applications was probably due to the fact that auxin promotes adventitious root formation in cuttings, which increases root growth (Acosta et al., 2008; Hartmann et al., 2010). Therefore, we infer that, without an IBA application, there was less root growth in the cuttings, resulting in a lower absorption of water and nutrients and, consequently, a slower growth of the plants. Santelices (2007) found similar results with Nothofagus glauca, where rooting increased proportionally with the IBA level at a concentration of 1.5%. Roh et al. (2005) observed that, 45 d after the application of 2,000 ppm of IBA on Ardisia crenata, the rooting percentage was 76% higher than the control plants. A higher and earlier rooting promotes rapid root growth and, therefore, a greater accumulation of FM and DM in this organ.
The DM accumulation tended to decrease with applications of IBA higher than 800 mg L-1. Thus, the lower accumulation of DM in the 1,200 and 1,600 mg L-1 treatments possibly could have been due to the chain of reactions that induce a high concentration of auxins, triggering ethylene production, which, according to Acosta et al. (2008), strongly inhibit root growth through the reduction of cell length growth.
In Myrciaria dubia, Olival and López (2005) reported that the application of 100 mg kg-1 of naphthalene acetic acid (NAA) induced a rooting percentage of 24.5, but that increasing the dose to 200 and 300 mg kg-1 caused the rooting to decrease by 6.7 and 2.3%, respectively, lower than the control (4.5%). Therefore, it can be deduced that increasing the IBA concentration above 800 mg L-1 results in root growth that does not increase but remains constant and possibly decreases. In this regard, Taiz and Zeiger (2010) affirmed that root growth only requires very low concentrations of auxin, while higher concentrations (which promote stem elongation) inhibit it.
The treatments of 800 mg L-1 of IBA with 0 cfu/mL of T. harzianum and 1,600 mg L-1 of IBA with 3·106 cfu/mL of T. harzianum generated cape gooseberry plants with a higher DM accumulation (Tab. 3). However, these applications do not show a significant trend when analyzed separately. The fact that the interaction was significant but the separate factors were not was probably due to the fact that, during rooting and subsequent plant growth, many variables, e.g. the substrate, the concentration of endogenous plant growth regulators and the plant nutrition, are involved, in addition to the relationship that can be established between the microorganism and the IBA (Hermosa et al., 2012).
For the root FM there were significant differences between the higher concentration treatments (3·106 and 4·106 cfu/mL) and the lower ones (without T. harzianum and the 2·106 cfu/mL treatment) (Fig. 2B). These results are different from those found with other plant organs (Tab. 2), where slower growth was presented by the control and the 4·106 cfu/mL suspensions, which shows that T. harzianum promotes root growth but not necessarily shoot growth. This higher accumulation of FM in the roots was probably due to the fact that, when the fungal hyphae establishes a close relationship with the root (Hoyos-Carvajal et al., 2009), it increases the specific area of this organ and, thus, moisture retention.
Root length
For this variable, significant differences for the interaction of T. harzianum and IBA concentrations were found, as well as for each of the factor levels. The root length did not show an increase proportional to the auxin concentration since the treatments with lower average values corresponded to the control and application of 1,200 mg L-1 (Tab. 2). This differs from Moreno et al. (2009), who observed that, in cape gooseberry treated with 200 to 800 mg L-1 IBA, the root length was directly proportional to the hormone concentration. It also contrasts with reports for Physalis ixocarpacuttings, where root length was higher than the control at concentrations of 1,500 and 3,000 mg L-1 IBA (García et al., 2001), which shows the largest differences for the effect of this hormone between plant species of the same genus.
The increased root length growth with 800 mg L-1 of IBA could be explained by the ability of auxin to decrease the rigidity of the cell wall by acidifying the same, which causes the production of proteins (expansins) that are also involved in cell wall acidification (Taiz and Zeiger, 2010) causing cell growth to increase its volume.
In this sense, Santelices (2007) established that root length is directly proportional to the concentration of IBA. Similar results were obtained by Ruiz-Solsol and Mesén (2010) in Plukenetia volubilis, with concentrations of 0.10, 0.15, and 0.20% of IBA, observing the longest roots with 0.20% of IBA.
In the case of the T. harzianum suspensions, the highest root length was presented with the suspension of 3·106 cfu/mL and the lowest response was obtained with 2·106 and 4·106 cfu/mL (Tab. 2). This result coincides with the higher accumulation of DM in the plants when the suspension of 3·106 cfu/mL was applied (Tab. 2).
Total dry mass
According to the ANOVA, the total DM showed significant differences between the levels of IBA and T. harzianum, but not for the interaction between these factors. The plants with 800 mg L of IBA accumulated more DM, but without significant differences with the concentrations of 1,600 and 1,200 mg L-1 (Fig. 3).
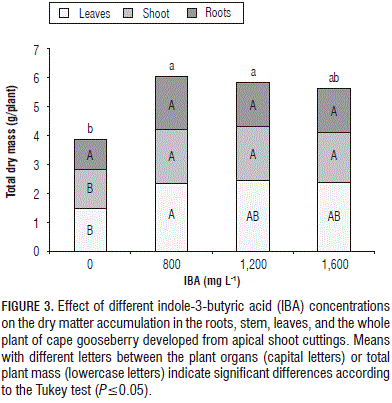
Figure 3 shows that the shoot was the organ that provided the largest amount of DM in the plants, with an average of 40% for all treatments. The dry biomass accumulation of the shoot is associated with FM accumulation and its length. Blythe et al. (2004) found that the foliar application of IBA and NAA or the dipping of cuttings in a solution of auxin (4.920 mM IBA + 2.685 mM NAA) resulted in a higher stem length and more shoot formation in Aglaonema modestum, Ficus benjamia, and Gardenia augusta.
Furthermore, the accumulation of DM tended to decrease when the IBA concentration exceeded 800 mg L-1. Similarly, it was observed that the DM was lower than when the hormone was not applied, which means that IBA generally promotes plant growth.
There was a tendency for reduction of the total DM accumulation when increasing the IBA concentration above 800 mg L-1, which is associated with a reduced accumulation, especially in roots, probably because ethylene and ABA can inhibit their growth, because these plant growth regulators may be produced when substantially increased au xin concentrations occur (Hansen and Grossman, 2000).
By comparing the different doses of T. harzianum with each other, the accumulation of total DM was greater for the treatment of 3·106 cfu/mL, showing statistical differences with the dose of 4·106 cfu/mL, but not with the control (Tab. 2), which shows that the real growth of the plants, expressed in DM, was not favored by the highest concentration of the fungus but only by a specific population of this Trichoderma. This increase in growth, as caused by T. harzianum, has been reported by other authors, e.g. bean plants increased growth when inoculated with T. harzianum, which enhanced the DM and stem length (Hoyos-Carvajal et al., 2009). Also, the fungus concentration of 4·106 cfu/mL could have generated competition for nutrients due to its high population, affecting the availability of these nutrients for the plant; also, T. harzianum produces enzymes that cause cell wall degradation, not only in the plant but also in the fungus, and that are favored by root exudates (Woo et al., 2007).
In this regard, plant growth depends on many factors, including the environmental conditions to which the plant is subjected. The fungus T. harzianum has a strong contact with the roots of plants (Hoyos-Carvajal et al., 2009), increasing their specific area and solubilizing different nutrients, such as phosphorus and nitrogen, and has chelating properties, mainly for Fe and Mnions (Altomare et al., 1999), which favor plant development.
The growth inhibition caused by T. harzianum at a certain concentration has been reported in some plants. Cubillos-Hinojosa et al. (2009) affirmed that the total DM increased in passion fruit seedlings (Passiflora edulis) propagated from seeds and treated with suspensions of 104, 106, and 108 cfu/mL; however, the speed and the germination percentage were lower with the application of 104 cfu/mL of the strain TCC-005-104 of T. harzianum than without an application. Furthermore, Sosa et al. (2006) found that Brachiaria decumbens plants inoculated with T. harzianum and arbuscular mycorrhizae showed less DM accumulation than when only T. harzianum was applied and argued that this inhibition could be due to factors not considered in the study, such as the host plant of T. harzianum strain used, type of substrate and the environmental conditions.
Chlorophyll content
A lower concentration of chlorophyll (SPAD units) was found in the leaves of the treatment of 1,600 mg L-1 of IBA, whereas that treatment with 800 mg L-1 of IBA showed the highest amount of chlorophyll (Tab. 1). As mentioned above, excess IBA can cause the production of ethylene and ABA, which, along with other plant growth regulators, including jasmonic acid, regulate the expression of certain genes involved in the degradation of chlorophyll (Hansen and Grossman, 2000); so, this ethylene production could be involved in the lowest value of the SPAD units registered in the cape gooseberry plants receiving more than 800 mg L of IBA.
The leaf chlorophyll content was not significantly affected by the different T. harzianum suspensions (Tab. 2), showing an average of 39.4 SPAD units. The beneficial fungus did not significantly affect the chlorophyll content or, presumably, the photosynthetic activity; nevertheless, it facilitated the absorption of nutrients and water, which makes the overall development of the plant more efficient (Hoyos-Carvajal et al., 2009). This differs from the observation of Lo and Lin (2002) in cucurbits, where the application of native strains of T. harzianum increased the chlorophyll content by up to 125%, as compared to the control; this difference could have been due to the species or the methodology used.
Conclusions
The results of the present study showed that IBA promoted the growth (FM, DM, and leaf area) of cape gooseberry plants developed from cuttings and that the application of 800 mg L-1 of IBA favored root growth, but IBA concentrations above 800 mg L-1 did not promote root growth. The application of 3·106 cfu/mL suspensions of T. harzianum increased the growth of all plant organs, whereas the most favorable interaction between the two factors, which increased the growth of the cape gooseberry plants, occurred when 800 mg L-1 of IBA and T. harzianum at a concentration of 3·106 cfu/mL were applied.
Literature cited
Acosta E., M., J. Sánchez B., and M. Bañón A. 2008. Auxinas. pp. 377-398. In: Azcón-Bieto, J. and M. Talón (eds.). Fundamentos de fisiología vegetal. 2nd ed. McGraw-Hill Interamericana, Barcelona, Spain. [ Links ]
Altomare, C., W.A. Norvell, T. Björkman, and G.E. Harman. 1999. Solubilization of phosphates and micronutrients by the plant-growth-promoting and biocontrol fungus Trichoderma harzianum Rifai 1295-22. Appl. Environ. Microbiol. 56, 2926-2933. [ Links ]
Batta, Y.A. 2007. Control of postharvest diseases of fruit with an invert emulsion formulation of Trichoderma harzianum Rifai. Postharv. Biol. Technol. 43, 143-150. Doi: 10.1016/j.postharvbio.2006.07.010 [ Links ]
Blythe, E.K., J.L. Sibley, J.M. Ruter, and K.M. Tilt. 2004. Cutting propagation of foliage crops using a foliar application of auxin. Sci. Hortic. 103, 31-37. Doi: 10.1016/j.scienta.2004.04.011 [ Links ]
Coskuntuna, A. and N. Özer. 2008. Biological control of onion basal rot disease using Trichoderma harzianum and induction of antifungal compounds in onion set following seed treatment. Crop Prot. 27, 330-336. Doi: 10.1016/j.cropro.2007.06.002 [ Links ]
Cubillos-Hinojosa, J., N. Valero, and L. Mejía. 2009. Trichoderma harzianum como promotor del crecimiento vegetal del maracuyá (Passiflora edulis var. Flavicarpa Degener). Agron. Colomb. 27, 81-86. [ Links ]
Donoso, E., G.A. Lobos, and N. Rojas. 2008. Efecto de Trichoderma harzianum y compost sobre el crecimiento de plántulas de Pinus radiata en vivero. Bosque 29, 52-57. Doi: 10.4067/S0717-92002008000100006 [ Links ]
Fischer, G., G. Ebert, and P. Lüdders. 2007. Production, seeds and carbohydrate contents of cape gooseberry (Physalis peruviana L.) fruits grown at two contrasting Colombian altitudes. J. Appl. Bot. Food Qual. 81, 29-35. [ Links ]
Fischer, G., A. Herrera, and P.J. Almanza. 2011. Cape gooseberry (Physalis peruviana L.). pp. 374-396. In: Yahia, E.M. (ed.) Posthar vest biolog y and technolog y of tropical and sub- tropical fruits. Vol. 2: Acai to citrus. Woodhead Publishing, Cambridge, UK. [ Links ]
Fischer, G., P.J. Almanza-Merchán, and D. Miranda. 2014. Importancia y cultivo de la uchuva (Physalis peruviana L.). Rev. Bras. Frutic. 36, 1-15. Doi: 10.1590/0100-2945-441/13 [ Links ]
Galvis, J.A., G. Fischer, and O. Gordillo. 2005. Cosecha y poscosecha de uchuva. pp. 165-190. In: Fischer, G., D. Miranda, W. Piedrahita, and J. Romero (eds.). Avances en cultivo, poscosecha y exportación de la uchuva (Physalis peruviana L.) en Colombia. Unibiblos, Universidad Nacional de Colombia, Bogota. [ Links ]
Grossmann, K. 2010. Auxin herbicides: current status of mechanism and mode of action. Pest Manage. Sci. 66, 113-120.Doi: 10.1002/ps.1860 [ Links ]
Hansen, H. and K. Grossman. 2000. Auxin-induced ethylene triggers abscisic acid biosynthesis and growth inhibition. Plant Physiol. 124, 1437-1448. Doi: 10.1104/pp.124.3.1437 [ Links ]
Hartmann, H.T., D.E. Kester, F.T. Davies, and R. Geneve. 2010. Hartmann & Kester's plant propagation: principles and practices. 8th ed. Prentice Hall, Englewood Cliffs, NJ. [ Links ]
Hoyos-Carvajal, L., S. Orduz, and J. Bissett. 2009. Growth stimulation in bean (Phaseolus vulgaris L.) by Trichoderma. Biol. Control 51, 409-416. Doi: 10.1016/j.biocontrol.2009.07.018 [ Links ]
Jiménez, C., N. Sanabria de Albarracin, G. Altuna, and M. Alcano. 2011. Efecto de Trichoderma harzianum (Rifai) sobre el crecimiento de plantas de tomate (Lycopersicon esculentumL.). Rev. Fac. Agron. (LUZ) 28, 1-10. [ Links ]
Lo, C.T. and C.Y. Lin. 2002. Screening strains of Trichoderma spp for plant growth enhancement in Taiwan. Plant Pathol. Bull. 11, 215-220. [ Links ]
López A., F.J., N.R. Guío T., G. Fischer, and D. Miranda L. 2008. Propagación de uchuva (Physalis peruviana L.) mediante diferentes tipos de esquejes y sustratos. Rev. Fac. Nal. Agr. Medellin 61, 4347-4357. [ Links ]
Miranda, D. 2005. Criterios para el establecimiento, los sistemas de cultivo, el tutorado y la poda de la uchuva. pp. 29-53. In: Fischer, G., D. Miranda, W. Piedrahita, and J. Romero (eds.). Avances en cultivo, poscosecha y exportación de la uchuva (Physalis peruviana L.) en Colombia. Unibiblios, Universidad Nacional de Colombia, Bogota. [ Links ]
Miranda, D. and M. Perea. 2012. Sistemas de propagación en fruticultura. pp. 73-98. In: Fischer, G. (ed.). Manual para el cultivo de frutales en el trópico. Produmedios, Bogota. [ Links ]
Moreno, N.H., J.G. Álvarez-Herrera, H.E. Balaguera-López, and G. Fischer. 2009. Propagación asexual de uchuva (Physalis peruviana L.) en diferentes sustratos y a distintos niveles de auxina. Agron. Colomb. 27, 341-348. [ Links ]
Olival, C. and A. López. 2005. Efecto del ácido naftalenacético en el enraizamiento de estacas de Myrciaria dubia (Hbk.) Mc Vaugh, Camu, Camu. Folia Amazónica 14, 43-49. [ Links ]
Pacholczak, A., A. Ilczuk, E. Jacygrad, and K. Jagiello-Kubiec. 2012. Effect of IBA and biopreparations on rooting performance of Cotinus coggygria Scop. Acta Hort. 990, 383-389. [ Links ]
Ramírez-Villalobos, M., A. Urdaneta-Fernández, and G. Vargas-Simón. 2004. Tratamientos con ácido indolbutírico y lesionado sobre el enraizamiento de estacas de icaco (Chrysobalanus icaco L.). Agron. Trop. 54, 203-218. [ Links ]
Roh, M.S., A.K. Lee, and J.K. Suh. 2005. Production of high quality Ardisia plants by stem tip cuttings. Sci. Hortic. 104, 293-303. Doi: 10.1016/j.scienta.2004.09.001 [ Links ]
Ruiz-Solsol, H. and F. Mesén. 2010. Efecto del ácido indolbutírico y tipo de estaquilla en el enraizamiento de sacha inchi (Plukenetia volubilis L.). Agron. Costarr. 34, 259-267. [ Links ]
Santelices, R. 2007. Efecto del ácido indolbutírico (AIB) y la presencia de hojas en el arraigamiento de estacas de Nothofagus glauca (Phil.) Krasser cosechadas en dos épocas diferentes. Ecol. Austral 17, 151-158. [ Links ]
Sosa R., T., J. Sánchez N., E. Morales G., and F. Cruz C. 2006. Interacción micorrizas arbusculares-Trichoderma harzianum (Moniliaceae) y efectos sobre el crecimiento de Brachiaria decumbens (Poaceae). Acta Biol. Colomb. 11, 43-54. [ Links ]
Taiz, L. and E. Zeiger. 2010. Plant physiology. 5th ed. Sinauer Associates, Sunderland, MA. [ Links ]
Woo, S.L., M. Roucco, R. Ciliento, P. Ambrosino, R. Marra, F. Vinale, S. Lanzuise, S. Ferraioli, I. Soriente, S. Gigante, D. Turra, F. Scala, and M. Lorito. 2007. A novel understanding of the three-way interaction between Trichoderma spp. the colonized plant and fungal pathogens. pp. 291-308. In: Robson, G.D., P. Van West, and G. Gadd (eds.). Exploitation of fungi. Cambridge University Press, Cambridge, UK. Doi: 10.1017/CBO9780511902451.017 [ Links ]