Serviços Personalizados
Journal
Artigo
Indicadores
-
Citado por SciELO
-
Acessos
Links relacionados
-
Citado por Google
-
Similares em SciELO
-
Similares em Google
Compartilhar
Agronomía Colombiana
versão impressa ISSN 0120-9965
Agron. colomb. vol.32 no.3 Bogotá set./dez. 2014
https://doi.org/10.15446/agron.colomb.v32n3.41797
Doi: 10.15446/agron.colomb.v32n3.41797
1 Department of Agronomy, Faculty of Agricultural Sciences, Universidad Nacional de Colombia, Bogota (Colombia). jtorresb@unal.edu.co
2 Technical Direction, Centro de Investigaciones del Banano (Cenibabano), Asociacion de Bananeros de Colombia (Augura). Medellin (Colombia).
Received for publication: 23 October, 2014. Accepted for publication: 27 November, 2014.
ABSTRACT
Banana production for export in the Uraba region of Colombia represents economic and social benefits for the country and demands improved agronomic management practices, especially for fertilization. The objective of this research was to evaluate the effect of different doses of nitrogen on crop development during two production cycles in order to adjust fertilizer recommendations to values consistent with plant requirements. Sixth-generation banana Williams plants were subjected to five treatments of nitrogen doses at five stages of development and during two production cycles. Two linear models, according to the phenological stage and vegetative structures, were used, assessing the variables of dry weight and nitrogen content. The statistical differences found for the effect of the nitrogen dose on the dry matter accumulation per plant organ and nitrogen content in the plants for the development stages per production cycle and between the cycles allowed for the selection of the 321.8 and 483 kg ha-1 ha of nitrogen doses as the better ones. In this research, the nitrogen dose of 483 kg ha-1 was the most successful at obtaining the highest nitrogen content in the plants. This research confirmed the practical utility of nutrient extraction curves because they allowed for the selection of the fertilizer dose with the best response.
Key words: fruits, plant physiology, growth, plant nutrition, modeling.
Resumen
La producción de banano para exportación en la zona de Urabá, Colombia, es importante porque representa beneficios económicos y sociales para el país, exigiendo mejorar las prácticas de manejo agronómico especialmente la fertilización. El objetivo de esta investigación fue evaluar diferentes dosis de nitrógeno a través del desarrollo del cultivo durante dos ciclos de producción para ajustar recomendaciones a valores acordes con las necesidades de la planta por fase de desarrollo. Se empleó un cultivo de sexta generación aplicándole cinco tratamientos con dosis de nitrógeno, durante cinco etapas de desarrollo y dos ciclos productivos. Se ajustaron dos modelos lineales de acuerdo a la etapa fenológica considerada y las estructuras vegetativas presentes, evaluando simultáneamente las respuestas peso seco y contenido de nitrógeno. Las diferencias estadísticas encontradas para el efecto de las dosis de nitrógeno, sobre las etapas de desarrollo del banano por ciclo productivo y entre ciclos, para acumulación de materia seca por órgano y por planta, como para el contenido de nitrógeno en planta, permitieron seleccionar las dosis de 321,8 y 483 kg ha-1 de nitrógeno como las de mejor comportamiento. En esta investigación sobresalió la dosis de 483 kg ha-1 de nitrógeno por obtener el mayor contenido de nitrógeno en planta. La presente investigación ratifica la utilidad de las curvas de extracción de nutrientes porque permitieron seleccionar las dosis de mejor respuesta.
Palabras clave: frutas, fisiología de plantas, crecimiento, nutrición de plantas, modelación.
Introduction
Bananas and plantains are important for the diet of about 400 million people worldwide (Perea, 2003). The planted area of the banana and plantain in Colombia is close to 380,000 ha, producing about 3 million tons of bunches per year (Danies, 2005). In 2013, approximately 48,325 ha of bananas were grown for export, with 35,425 ha in Uraba, Antioquia and 12,900 ha in the north of the Magdalena province and the south of the Guajira province (Quesada, 2013; Augura, 2014). Globally, Colombia occupied position 22 of banana producers in 2005, with a 2.2% share of the world market, equivalent to 1,500,000 t (Danies, 2005), while, for 2012, it ranked as number 12 with 1,982,702 t, equivalent to USD $ 502,420,000; by 2013, the export volume decreased by 9.98%, but a 4.05% increase in price per kg mitigated this decrease in value (6.33%) (Danies, 2005; Muñoz, 2014). Export bananas occupy about 44,000 ha and generate about 60,000 jobs (Quesada, 2013).
Research on the banana from 1992 to today has been focused on fertilization parameters adjusted to the specific conditions of each country or region (Espinosa and Mite, 2002). An example of this was the ranges of mineral nutrients for both soil and leaf samples proposed in 1995 (López and Espinosa, 1995), which served as a basis for countries, including Colombia, to start the adjustment process for fertilizer applications in the main producing areas and cultivars. Within this context, the Uraba region received the guidelines that raised considerations on the application of some mineral nutrients and initiated research in this area (Sánchez and Mira, 2013).
In an experiment conducted in China to evaluate accumulation, absorption, and distribution of mineral nutrients in the banana (Musa ABB Pisan Awak), the total DM per plant was found distributed as 16.4% (17.6 kg) in leaves, 32.8% in pseudostem, 9.6% in corm, 37.3% in fruits, 1.1% in rachis, and 2.8% in roots; with respect to nutrient accumulation in plants, potassium reached the highest values (521.7 g/plant), followed by nitrogen and calcium (167 and 118.3 g/plant, respectively) and the highest production of 60 t ha-1 was obtained with a dose of 385.6 kg ha-1 of nitrogen (Yang et al., 2013).
Studies on nutrient absorption may not always be used as a diagnostic tool, as is done with foliar analysis, but they can give a solid quantitative basis for fertilization plans. Specifically, these studies help to elucidate the amount of nutrients in kg per ha that is absorbed by the crops to generate a particular production in a defined amount of time. This research can also be used to construct the curves of nutrient absorption that provide information about nutrient absorption during the crop cycle, thus, allowing for the determination of the periods of highest nutrient accumulation and the most appropriate times for fertilization (Bertsch, 2003). Absorption studies at the end of the development cycle of the crops are recommended in order to accurately define fertilization plans (Bertsch, 2009).
One of the main nutrients for crop development at the vegetative stage is nitrogen. Its content in plants may vary from 1 to 6% dry weight; it is absorbed by roots as nitrate, nitrite, and ammonium, with nitrate being the more mobile form in soil and the one that plants absorb to a higher extent (Havlin et al., 2013), but it is also the form that is lost through leaching (Navarro and Navarro, 2003; Zapata and Osorio, 2010). Typically, plant residues are an important source of nitrogen, but their contribution to the soil depends on the decomposition generated by the microbial biomass, a process that depends on the origin of the organic material (Zapata and Osorio, 2010). The ammonification of nitrogen during the mineralization of soil organic matter acidifies soil because it is an anaerobic process (Plaster, 2013), allowing nitrogen loss through volatilization as ammonia (Navarro and Navarro, 2003; Zapata and Osorio, 2010). Most studies that have evaluated nitrogen fertilization in the banana have been limited to measuring production as the principal response and obtained dissimilar results because of the interaction between the cultivar, soil, environment, and N rate (Robison and Galán, 2011). For example, Colque et al. (2005) and Aristizábal (2010), working with different soils and cultivars, achieved favorable responses in production with nitrogen doses lower than 300 kg ha-1, in contrast with that found by Castillo et al. (2011) and Yang et al. (2013), who registered the best production with nitrogen doses higher than 350 kg ha-1.
Few studies have addressed the relationship between banana fertilization and nutrient accumulation and distribution in different organs of the plants in order to assess production and fruit quality while taking into account the plant material and soil. Some authors (Castillo et al., 2011; Ndukwe et al., 2012) achieved the highest level of nitrogen accumulation in plants at harvest with doses between 250 and 450 kg ha-1, with values that differed in each study, based on the soil characteristics and contents of soil nitrogen. Because of the importance of this interaction, it was necessary to address this issue in the Uraba region, where fertilizer management relies more on the economic criteria than on the technical criteria, generating, on most plantations, applications with inappropriate levels rather than the ones recommended by Cenibanano for the varieties in the region. The goal of the present research was to evaluate the absorption, distribution, and accumulation of nitrogen in banana plants during two production cycles, using different levels of fertilization with nitrogen.
Materials and methods
Location and characteristics of the study area
The research was conducted in the Experimental and Demonstration Field of Augura (Carepa, Colombia), located at 7°46'46" N, 76°40'20" W, with an average elevation of 20 m a.s.l. The soils corresponded to the taxonomic classifications Fluventic Eutrudepts fine, Fluvaquentic Eutrudepts loamy fine over clay, and Vertic Endoaquepts loamy fine (Gutiérrez, 2007). The climatic conditions during the study period were 87% average relative air humidity, 23.2, 26.7, and 32.3°C minimum, average, and maximum average monthly temperatures, respectively, and 1,700 h year-1 average of sunshine hours. The average rainfall registered during the study was 845 mm for the first production cycle (August 2011 to April 2012) and 2,088 mm for the second production cycle (February to December 2012).
A sixth generation crop belonging to the group AAA Cavendish, subgroup: giant clone Williams, with an average height of 3.5 m and easily packed bunches was used. It is one of the two main export clones in many tropical regions (Robinson and Galán, 2011).
Assessed treatments and variables
Five treatments defined from a base fertilizer rate proposed by Cenibanano were selected and adjusted based on the results of a soil analysis prior to the start of the experiment. The base dose (kg ha-1) was 321.8 of nitrogen, 87.1 of phosphorus pentoxide, 678.8 of potassium oxide, 50.5 of calcium oxide, 117.5 of magnesium oxide, 64.2 of sulfur, 1.4 of boron and 9.3 of zinc. The treatments were absolute control (no fertilizer applied), control without nitrogen (0 kg ha-1), and 161.0, 321.8, or 483.0 kg ha-1 of nitrogen. Other mineral nutrients were applied at constant rates at the recommended doses.
In each experimental unit (area of approximately 1,563 m2 with an average of 250 plants), 15 plants were selected with the same growth characteristics (5 to 6 weeks of age and 120-150 cm height), for purposes of measuring and sampling 10 plants; in the course of the experiment, it was necessary to select five more plants per experimental unit to replace some plants that were affected by black Sigatoka, had developmental problems caused by weather conditions or plants growing at the field edges that could not be used due to the edge effect. To evaluate the selected variables, five phases of development were chosen during the production cycle (vegetative, differentiation, bloom, fruit filling, and harvest) that were identified by Cenibanano. In each phase, one of the selected plants was taken at the beginning of the study and dissected to obtain the required data.
The variables of production (kg ha-1), dry weight per organ and per plant (kg), and nitrogen content (kg per plant) were measured with the methodology reported by Martinez et al. (2006). The nitrogen content in the plant material was determined using the elemental analyzer TruSpec Leco CN® (Leco Inc., St Joseph, MI) in the Laboratory of Water and Soils of the Faculty of Agricultural Sciences, Universidad Nacional de Colombia, Bogota.
Agronomic management of the experimental plots
Agricultural practices employed in the study were the same ones used in the commercial plot, except that the fertilizer rates were adjusted according to the treatments. The onset of fertilization cycles was programmed from the most flowering period of the plants for the Uraba region, which usually occurred between September and October, starting this practice with the sucker. The fertilizer sources were urea, double sulfate of potassium and magnesium, calcium borate, 32% potassium chloride (YARA Colombia, Barranquilla, Colombia); cal and solufos (Enmiendas y Fertilizantes Agrícolas, Medellin, Colombia).
Statistical analysis
For data collection and subsequent statistical processing, five treatments were established in four replicates that were located in the field, based on the spatial variation of the soils, attributable to soil taxonomy. The statistical analysis involved descriptive and inferential components. In the first case, averages for each of the responses for each level of inter- and intra-subject factors were obtained. These averages are presented in bar charts and dotted lines to show the non-continuous nature of variables associated with the treatments. For the inferential component, the relationship between the different responses to different levels of factors was modeled by a linear model associated with a repeated measures design with two between-subjects factors (nitrogen doses and replicates) and a within-subjects factor (cycle production). The statistical method used in the modeling process was the analysis of multivariate variance. Importantly, covariate soil moisture modeling was involved, but it did not meet the statistical assumptions for incorporation and was removed from the model. Also, the univariate analysis of each response for the same design was used to supplement the multivariate analysis (see algorithm in SAS code). The high correlations between responses in the different cycles justified the use of multivariate analysis over the univariate one; however, in practical terms, the two analyses led to similar conclusions. These analyzes were supplemented by the response profiles in different cycles due to the clear interaction with time, so that the definitive conclusions were based mainly on the analysis of the profiles (Neter et al., 1996; Montgomery, 2012).
Results and discussion
Accumulation of dry matter
The statistical analysis for this variable showed highly significant differences for the development stages (P≤0.0001) and treatment (P≤0.0001) in conjunction with time (interactions). Figures 1A to E describe the interactions that differed by average response for two production cycles. It is noteworthy that, in the presence of an interaction, the significance associated with the main effects was not relevant, so that the choice of the most desirable treatment was based on the different graphic profiles.
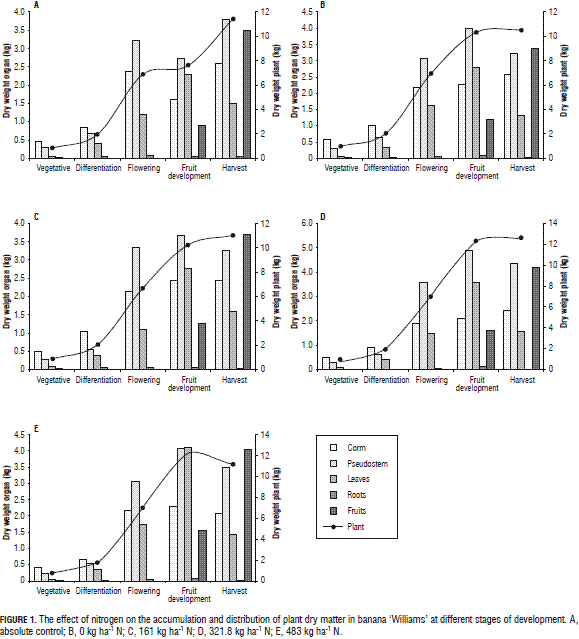
The distribution model for the organ and developmental stage (Fig. 1) showed no differences between the treatments in the dry weight distribution for organs during the development of banana 'Williams'; this indicated the average treatment effect for the two cycles in the accumulation of DM per organ and developmental stage. By observing the organs involved in the process of DM accumulation in the first two stages of development (Fig. 1A to D), a predominance of corm over the pseudostem and leaves could be seen, while, in bloom, the pseudostem achieved the highest dry mass, followed by the corm and leaves; this sequence was retained during the filling of the fruit bunch, whose dry weight in all treatments was lower than the weight of the leaves. At harvest, the highest dry matter accumulation was found in the bunch, followed by the pseudostem, corm, and leaves. Robinson and Galán (2011) confirmed this behavior as typical for the cultivar employed. Martínez and Cayón (2011), in Valery and Grand Nain cultivars, found that, at flowering, the highest DM accumulation was observed in leaves followed by pseudostem and corm, while, at harvest, the order was: bunch, pseudostem, and leaves. This result is not consistent with that observed by Castillo et al. (2011) in banana Dominico at harvest, with an order of dry matter accumulation of: pseudostem, corm, leaves, and bunch; these findings were different from what was found in the present research for the same stage of development, where the order was: bunch, pseudostem, and corm. The reduction in DM accumulation in the present study was, possibly, associated with the levels of monthly precipitation that occurred in the area close to the harvest period. These levels, even though close to normal, showed low frequencies with varying intensities, which allowed for prolonged dry periods that manifested in a reduced number of leaves with a consequent reduction in DM accumulation. Robinson and Galán (2011) claimed that the number of leaves may be affected by adverse weather conditions or diseases and that the leaf number may be between 5 to 10 for harvest time in subgroup Cavendish, to which the cultivar Williams belongs.
By analyzing the effect of the nitrogen dose on corm behavior at different stages of development (Fig. 1A to E), it could be seen that its dry weight tended to decrease towards harvest with the highest dose of nitrogen (483 kg ha-1, Fig. 1E); whereas, in the other treatments, it tended to remain the same or increase towards the harvest. This indicates that high levels of nitrogen stimulated the translocation of nutrients to other sources or bunches, which could have involved a reduction of DM in the corm (Robinson and Galán, 2011).
The pseudostem (Fig. 1A to 1D) had a higher tendency to accumulate dry matter in the treatments of 321.8 and 483 kg ha-1 N (Fig. 1D and 1E), until the fruit filling; after that, this value decreased in all of the treatments to very similar values, except in the treatment with 321.8 kg ha-1, showing the highest accumulation value. This effect could be explained by the fact that corm weight depends not only on nitrogen dose but also on a connection that exists between a mother plant and a sucker from one cycle to another, allowing for a potentiation of the effect of the applied nitrogen in the interaction with climatic and soil conditions (Robinson and Galán, 2011).
The highest accumulation of DM in the bunch was obtained in the treatments of 321.8 and 483 kg ha-1N for the two stages of development ( Fig. 1D and E), which coincides with the findings of Yang et al. (2013) at a dose of 385.6 kg ha-1 N. A comparison of the cycles shows that the second cycle had a higher accumulative proportion of dry matter than the first one; the explanation of this fact has already been mentioned and discussed for the pseudostem.
The production (t ha-1) and number of boxes with bunches for export achieved in each treatment are shown in Tab. 1. It could be observed that the doses of 321.8 and 483 kg ha-1 N produced the higher values of these variables, following the order mentioned for dry matter accumulation, where the main factor associated with the doses was the "ratio" or the number of boxes that can be filled with a bunch. The ratio depends not only on the weight of the bunch but also on the number of appropriately sized hands and fingers; usually, in the selection process, those fruits that do not meet the standards required for export are removed, determining the so-called depletion or waste material. In this study, the average value of discarded material was 10%, which allowed for calculating the effective ratio and the number of boxes per treatment per cycle.
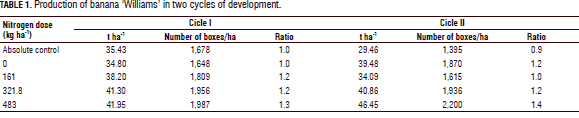
Complementing the multivariate analysis of variance, a univariate analysis was performed because all of the factors had the same effect on each response; this was the case for the total dry matter accumulation per plant, where the univariate analysis showed significant differences only between the developmental stages for the two cycles (P≤0.0001).
Comparing the accumulation curves (Fig. 1), it could be seen that the treatments of 321.8 and 483 kg ha-1 N (Fig. 2D and E) tended to increase the DM content during the development of the banana plants. The total plant dry weight corresponded to the sum of the organ dry weight; therefore, this increase was influenced by the organs that accumulated the highest weight; for that reason, it demonstrated the favorable effect of increasing doses of nitrogen over time.
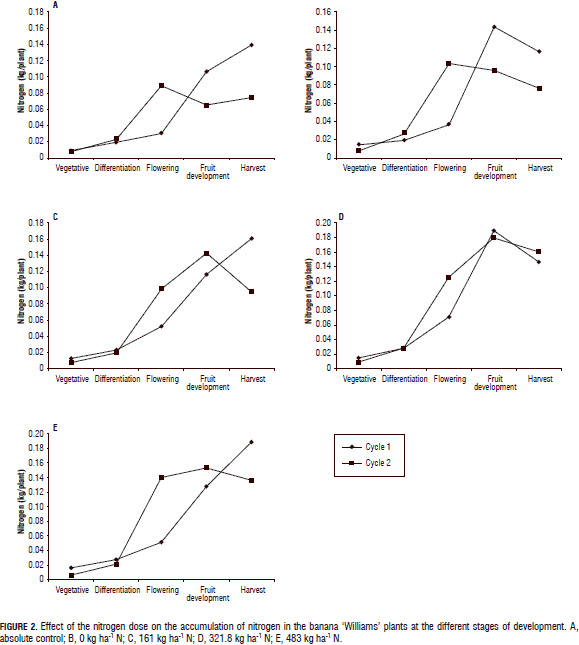
The dry matter accumulation in the pseudostem and plant decreased from the stage of fruit filling to harvest (Fig. 1B to D), an effect considered normal with the highest accumulation of DM in the bunch and translocation of nutrients to the organs of higher accumulation, such as corm and, especially, pseudostem, coupled with the reduction of the contribution of the leaves (Robinson and Galán, 2011).
Nitrogen content in the plants
The nitrogen behavior (Fig. 2A-E) was similar to that of the dry weight of the plants (Fig. 1A to 1D), confirming the positive effect of 321.8 and 483 kg ha-1 N for the two cycles (Fig. 2D and E), as compared to other treatments. Also, the differences between the cycles for all of the treatments, except for the treatment of 321.8 kg ha-1 N, were notable. In addition to being the treatment that resulted in the most accumulated nitrogen, this treatment showed similar values in the two cycles, approaching the treatment with 483 kg ha-1 N in the first cycle.
The high (greater than 350 kg ha-1) dose of nitrogen used in some studies achieved the highest yield, which implies better responses in dry matter accumulation and nitrogen content in the plant (Srikul and Turner, 1995; Orozco et al., 2006; Nyombi et al., 2010). These results differed from those found by Castillo et al. (2011), who applied nitrogen between 560 and 750 kg ha-1 on banana Dominico (Musa sp.) and obtained a nitrogen content of 38 g/plant, which was below the value reported in the present study, about 162 g/plant with the dose of 483 kg ha-1. Yang et al. (2013) found a 167 g nitrogen content in plants with doses of 385.6 kg ha-1 N in a cultivar of banana (Musa ABB, Pisang Awak), which was close to the value found in the present research (162 g with a nitrogen rate of 483 kg ha-1). The differences in the values of nitrogen accumulation in the plants, including the studies mentioned herein, are due to the cultivars employed and their interaction with the environmental conditions and soils, besides the dose of applied nitrogen (Robinson and Galán, 2011). Murthy and Kotur (1998) measured the nitrogen content in different plant organs during the development of banana Robusta and used doses of 340 kg ha-1 of nitrogen and varying times of application; these authors found the highest nitrogen content in the plants at harvest when they applied 33.91% of the dose in bloom, a result that contrasted with the one of present study, where the times of application employed in the region and nitrogen doses were those that had marked differences. It should be clarified that the results of nitrogen accumulation obtained by Castillo et al. (2011) and Yang et al. (2013) at harvest were different from the results found in the present study, where accumulation curves allowed for an observation of the evolution of nitrogen accumulation at each fertilizer dose used during the growth season, that permitted the selection of the dose with the best performance. Similar results were obtained by Bautista et al. (2012) and Suarez and Torres (2014) who analyzed nutrient accumulation curves for different treatments in Criolla potatoes and managed to select the best fertilizer dose with the best cost-benefit relationship.
Analyzing the correlation between the nitrogen content per plant and dry matter accumulation per plant and per organ showed that the highest significance was obtained for the total dry weight per plant for the two cycles (0.76 and 0.85, respectively, with P≤0.0001 for both correlations), a situation that indicates that, with a higher DM accumulation in the plants, the nitrogen content increased (Martínez and Cayón, 2011). This effect was manifested with the doses of 321.8 and 483 kg ha-1 N, having the highest nitrogen content with the dose of 483 kg ha-1N when the average values for the two cycles were compared for these two treatments (0.153 and 0.162 kg/plant nitrogen content at doses 321.8 and 483 kg ha-1 N, respectively).
The absolute control and 0 kg ha-1 N application (Fig. 2A and B) permitted the establishment of the quantitative differences in accumulation of dry matter and nitrogen content and the verification of the dependence between the mother plant and the sucker, including the nutrient cycling phenomenon that normally occurs when a mother plant is harvested, starts to decompose, and, before terminating its physiological functions, transfers the nutrients to the sucker (Robinson and Galán, 2011). The same authors showed that deficiencies in nitrogen are revealed in decreases of dry weight of the bunch, a situation that was found in this study, where the treatments without nitrogen or with a low dose (161 kg ha-1) resulted in a lower dry weight than those with doses of 321.8 and 483 kg ha-1 (Tab. 1). However, some of these values, despite being low, had proximity to the treatment of 321.8 kg ha-1 for the first cycle (absolute control with 3.67 kg DW/plant and the dose of 161 kg ha-1 with 3.80 kg DW/plant, respectively), showing a possible effect of nutrient recycling in these treatments for this cycle. This situation was not observed for the second cycle, where the effect of recycling was lower, possibly due to the action of time, when not applying nitrogen or a low application; a behavior that proves the nutritional dependence between the mother and sucker during the different development cycles in banana plants (Robinson and Galán, 2011).
The reduced effect of nutrient recycling in the second cycle for nitrogen showed a lower input of nutrients from the mother to the sucker in succession, an effect associated with the decreased soil organic matter between the first and the second cycle, with an average value that varied from 3.4 to 2.75%. The reduction in organic matter occurred not only for the soil treatment without nitrogen, but was observed in all of the soils of the experiment where the treatments were installed; this effect can be considered as a loss of soil quality. A management plan for soil organic matter could be established in the future that would be related to nitrogen fertilization plans to achieve better and more efficient processes, resulting in the accumulation of DM and nitrogen contents in 'Williams'banana plants in the study area. This research confirmed the usefulness of extraction curves for mineral nutrients because they allowed for the selection of the doses with a better response in nitrogen accumulation, facilitating the analysis and contrasting of the accumulation of dry matter per treatment and development phase.
Acknowledgements
The authors acknowledge the technical assistance of the employees of the Augura-Cenibanano (Banana Research Center) in Carepa, Antioquia, Colombia and the Yara Colombia company, the internship students of the University of Cordoba, Colombia who participated in the collection of field data, the undergraduate and master degree students of the Faculty of Agricultural Sciences, Universidad Nacional de Colombia, Bogota who collaborated in processing samples in the laboratory, and the personnel of the Laboratory of Water and Soils of the Faculty of Agricultural Sciences at the Universidad Nacional de Colombia, Bogota during this study.
Literature cited
Aristizábal L., M. 2010. Efecto de la frecuencia de fertilización con nitrógeno y potasio sobre el crecimiento, producción y severidad de las sigatokas del plátano (Musa AAB) Dominico Harton. Agron. 18, 19-28. [ Links ]
Augura. 2014. Coyuntura bananera colombiana 2013. Medellin, Colombia. [ Links ]
Bautista J., H.F., W.L. Ramírez M., and J. Torres B. 2012. Nutrient uptake of the diploid potato (Solanum phureja) variety Criolla Colombia, as a reference point to determine critical nutritional level. Agron. Colomb. 30, 436-447. [ Links ]
Bertsch, F. 2003. Absorción de nutrimentes por los cultivos. Asociación Costarricense de la Ciencia del Suelo, San Jose. [ Links ]
Bertsch, F. 2009. Absorción de nutrimentes por los cultivos (CD). Asociación Costarricense de la Ciencia del suelo. San Jose. [ Links ]
Castillo G., A.M., J.A. Hernández M., E. Avitia G., J. Pineda P., L.A. Valdéz A., and T. Corona T. 2011. Extracción de macronutrientes en banano 'Dominico' (Musa spp.). Phyton 80, 65-72. [ Links ]
Colque, O., E. Iquize, and A. Ferrufino. 2005. Efecto de la fertilización nitrogenada y potásica en la producción del banano Musa AAA en fincas comerciales de tres localidades del Trópico de Cochabanba. Inf. Agron. Cono Sur 26, 1-7. [ Links ]
Danies L., R. 2005. Sector bananero en Colombia 1995. Superintendencia de Sociedades, Bogota. [ Links ]
Espinosa, J. and F. Mite. 2002. Estado actual y futuro de la nutrición y fertilización del banano. Inf. Agron. Cono Sur 48, 4-10. [ Links ]
Gutiérrez, J.C. 2007. Estudio detallado de suelos y clasificación de tierras con fines de riego del campo experimental de Augura, Carepa Antioquia. Augura; Cenibanano, Medellin Colombia. [ Links ]
Havlin, J.L., S.L. Tisdale, W.L. Nelson, and J.D. Beaton. 2013. Soil fertility and fertilizers: an introduction to nutrient management. 8th ed. Pearson Prentice Hall, Upper Saddle River, NJ. [ Links ]
López M., A. and J. Espinosa M. 1995. Manual de nutrición y fertilización del banano: una visión práctica de la fertilización. International Plant Nutrition Institute, Quito. [ Links ]
Martínez A., A.M. and D.G. Cayón S. 2011. Dinámica del crecimiento y desarrollo del banano (Musa AAA Simmonds cvs. Gran Enano y Valery). Rev. Fac. Nal. Agr. Medellin 11, 6055-6064. [ Links ]
Montgomery, D.C. 2012. Design and analysis of experiments. 8th ed. John Wiley & Sons, New York, NY. [ Links ]
Muñoz G., E. 2014. Mercado global del banano. Centro de Aprovechamiento de Acuerdos Comerciales (CAAC); Ministerio de Comercio Exterior, Bogota. [ Links ]
Murthy, S.V.K. and S.C. Kotur. 1998. Effect of time and placement method of ammonium sulphate and superphosphate on the comparative efficiency of N and P absorption and N utilization in 'Robusta' banana (Musa Xparadisiaca) using labelled fertilizers. Ind. J. Agric. Sci. 68, 765-768. [ Links ]
Navarro B., S. and G. Navarro G. 2003. Química agrícola: el suelo y los elementos químicos esenciales para la vida vegetal. 2nd ed. Ediciones Mundi-Prensa, Madrid. [ Links ]
Neter, J., M. Kutner, W. Wasserman, and C. Nachtsheim. 1996. Applied linear statistical models. 4th ed. McGraw Hill, New York, HY. [ Links ]
Ndukwe, O.O., C.O. Muoneke, K.P. Baiyeri, and A. Tenkouano. 2012. Effect of organic and inorganic fertilizer on nutrient concentrations in plantain (Musa spp) fruit pup. Afr. J. Biotech. 11, 1651-1658. Doi: 10.5897/AJB11.2278 [ Links ]
Nyombi, K., P.J.A Van Asten, M. Corbeels, G. Taulya, P.A. Leffelaar, and K.E. Giller. 2010. Mineral fertilizer response and nutrient use efficiencies of East African highland banana (Musa spp., AAA-EAHB, cv. Kisansa). Field Crops Res. 117, 38-50. Doi: 10.1016/j.fcr.2010.01.011 [ Links ]
Orozco R., J. and O. Perez Z. 2006. Tensión de humedad del suelo y fertilización nitrogenada en plátano (Musa AAA Simmonds) cv. Gran Enano. Agrociencia. 40, 149-162. [ Links ]
Plaster, E.J. 2013. Soil science and management. 6th ed. Cengage Learning, Clifton Park, NY. [ Links ]
Perea, M. 2003. Biotecnología: bananos y plátanos. Editora Guadalupe, Bogota. [ Links ]
Quesada, V.H. 2013. ¿Qué podemos aprender de la experiencia bananera en Colombia? Estudio sobre relaciones laborales en las fincas certificadas de Fairtrade en la región de Urabá. Fairtrade International, Bonn, Germany. [ Links ]
Robinson, J.C. and V. Galán S. 2011. Plátanos y bananas. 2nd ed. Mundi-Prensa, Madrid. [ Links ]
Sánchez T., J.D. and J.J. Mira C. 2013. Principios para la nutrición del banano. Augura; Cenibanano, Medellin, Colombia. [ Links ]
Srikul, S. and D.W. Turner. 1995. High N supply and soil water deficits change the rate of fruit growth of banana (cv. "Williams") and promote tendency to ripen. Sci. Hortic. 62, 165-174. Doi: 10.1016/0304-4238(95)00765-L [ Links ]
Suarez M., C.A. and J. Torres M. 2014. Nutrient uptake of the criolla potato (Solanum phureja var. Galeras) for the determination of critical nutritional levels. Agron. Colomb. 32(1), 59-69. Doi: 10.15446/agron.colomb.v32n1.41811 [ Links ]
Yang, B.M., L.X. Yao, G.L. Li, C.M. Zhou, Z.H. He, and S.H. Tu. 2013. Absorption, accumulation and distribution of mineral elements in plantain banana. Plant Nutr. Fertilizer Sci. 19, 1471-1476. Doi: 10.11674/zwyf.2013.0623 [ Links ]
Zapata, R.D. and W. Osorio. 2010. La materia orgánica del suelo. pp. 357-396. In: Burbano O., H. and F. Silva M. (eds.). Ciencia del suelo: principios básicos. Sociedad Colombiana de la Ciencia del Suelo (SCCS), Bogota. [ Links ]