Services on Demand
Journal
Article
Indicators
-
Cited by SciELO
-
Access statistics
Related links
-
Cited by Google
-
Similars in SciELO
-
Similars in Google
Share
Agronomía Colombiana
Print version ISSN 0120-9965
Agron. colomb. vol.33 no.1 Bogotá Jan./Apr. 2015
https://doi.org/10.15446/agron.colomb.v33n1.46759
Doi: 10.15446/agron.colomb.v33n1.46759
1 Department of Agronomy, Faculty of Agricultural Sciences, Universidad Nacional de Colombia. Bogota (Colombia). cgarciad@unal.edu.co
2 Technical Department, Flores del Amanecer. Tenjo (Colombia)
3 Faculty of Engineering, Universidad de La Sabana. Chia (Colombia)
Received for publication: 23 October, 2014. Accepted for publication: 30 March, 2015.
Abstract
Cabbage clubroot is a major constraint for the production of cruciferous species. This research aim at exploring the effect of Trichoderma koningiopsis (as the wettable powder formulated product Tricotec®) and dolomite on the disease and crop growth of cabbage. This study was carried out in a field with a prolonged history of clubroot incidence. The trial was set up in a randomized complete block design with five repetitions. The following treatments were applied: 0) absolute control; 1 and 2) liming with dolomite at 1.0 and 2.1 t ha-1; 3) Tricotec® at 200 g ha-1; 4) combination of treatments 1 and 3; 5) combination of treatments 2 and 3. The treatment with just Tricotec® applications gave the lowest disease incidence and severity and the heaviest plant dry weight. Joint applications of Tricotec® and dolomite at 2.1 t ha-1 reduced the crop growth variables and had the highest disease intensity. The Trichoderma concentration in the soil was increased by a factor of 10 in the plots that received just Tricotec®, but not in the limed plots. These results showed that T. koningiopsis, in a Tricotec® formulation, is a promising candidate for the control of cabbage clubroot and that its biological activity is affected by dolomite when applied jointly at 2.1 t ha-1.
Key words: biological control, plasmodiophorida, soil-borne pathogens, Rhizaria, agricultural lime.
Resumen
La enfermedad hernia de las crucíferas, es un factor limitante en la producción de estas hortalizas. El objetivo de este ensayo fue evaluar el efecto de Trichoderma koningiopsis (producto formulado Tricotec® en formulación de polvo mojable) y de cal dolomítica, sobre la enfermedad y del crecimiento de repollo. El estudio se estableció en un lote con registro de la enfermedad. Se empleó un diseño de bloques completamente aleatorizados con cinco replicas. Se aplicaron los siguientes tratamientos: 0) testigo absoluto, 1 y 2) cal dolomítica 1,0 y 2,1 t ha-1, 3) Tricotec®en dosis de 200 g ha-1, 4) combinación de los tratamientos 1 y 3; 5) combinación de los tratamientos 2 y 3. Los tratamientos donde únicamente se aplicó Tricotec® presentaron los menores valores de incidencia y severidad y el mayor peso fresco de la planta. Aplicaciones conjuntas de Tricotec® y cal dolomita en dosis de 2.1 t ha-1 redujeron las variables de crecimiento del cultivo además de presentar la mayor intensidad de la enfermedad. Las concentraciones de Trichoderma en suelo aumentaron en un factor de 10 para los tratamientos donde se aplicó unicamente Tricotec® pero no en los tratamientos donde se encaló. Estos resultados muestran que T. koningiopsis como producto formulado Tricotec® es un biocontrolador promisorio para el control de la hernia de las crucíferas y que su actividad biológicaes afectada por la cal dolomita cuando se aplica en dosis de 2.1 t ha-1.
Palabras clave: control biológico, plasmodiophorida, patógenos de suelo, Rhizaria, cal agrícola.
Introduction
The crop area planted with cruciferous plants in Colombia is over 3,500 ha (roughly 8,400 acres), representing 3.84% of the total area for vegetable crops and providing more than 450,000 labor wages per year (Jaramillo and Díaz, 2006). Four towns on the Bogota Plateau, southwest of the capital, provide more than 80% of the cabbage (Brassica oleraceae L. var. capitata L.) and 90% of the cauliflower (B. oleraceae L. var. botrytis L.) and broccoli (B. oleraceae L. var. italica L.) of the domestic market (Tamayo and Jaramillo, 2004). Clubroot, caused by the soil-inhabiting protozoan obligate parasite Plasmodiophora brassicae Woronin, is the most common and economically important disease of these crops. P. brassicae is generally regarded as belonging to the supergroup Rhizaria, the phylum Cercozoa and the class Phytomyxea (Nikolaev et al., 2004). This disease is responsible for yield losses in the order of 20 to 50% (Jaramillo and Díaz, 2006).
Although P. brassicae is favored by acid soils, it can also thrive under alkaline conditions if and when the temperature, moisture and inoculum potential are high (Dixon, 2007). In general, favorable conditions for the development of clubroot are related to soil acidity, high moisture and temperatures, abundant inoculum and crop susceptibility. P. brassicae is easily spread by seedlings and tillage implements. In this way, it has spread globally from its most probable origin, near the domestication site of Brassicae on the European shores of the Mediterranean (Howard et al., 2010).
The life cycle of P. brassicae alternates between soil, root hairs and cortical cells of the host plants (Ludwig-Müller and Schuller 2008; Kageyama and Asano, 2009). Resting spores, structures with long viability, are released from the cells after root decay. Their germination gives rise to the first stage of biflagellate zoospores, called primary zoospores. These zoospores glide in the aqueous soil phase towards the surface of root hairs where they undergo encystment. Several mitotic divisions take place within each invaded cell, resulting in a multinucleate cytoplasmic mass called primary plasmodium; three to 5 days after invasion (dai), the primary plasmodia break down and evolve into sacs that contain a second stage of zoospores, which swim upon release and penetrate other root hairs in a similar fashion or, alternatively, penetrate cortical tissues where they promote the synthesis of cytokines that stimulate cell division and metabolism of the indole-3-acetic acid; this abnormal metabolic activity leads to cellular hyperplasia and hypertrophy that produce the typical clubroot root deformities; at about 15 dai, the pathogen's nuclei undergo synchronous mitosis in each invaded cell, resulting in a secondary multinucleate plasmodium that, upon fragmentation at about 23 dai, becomes a sac full of thick-walled resting spores (Ludwig-Müller et al., 1997; Ludwig-Müller and Schuller, 2008).
Root cell proliferation and hypertrophy strengthen the carbohydrate sink, thereby affecting nutritional levels in the aerial parts of the plant (Ludwig-Müller et al., 2009). Root symptoms become evident when digging out the infected plants, swellings and deformities, generally referred to as clubbing (Kageyama and Asano, 2009).
Clubroot management is particularly complicated by the long viability of resting spores in the soil, up to 20 years in the absence of hosts and under adverse conditions, as verified by Wallenhammar (1996). The long survival of resting spores is attributed to the presence of chitin, proteins and lipids in their walls, which protect them from microbial degradation (Dixon, 2007).
Given the persistence of the resting spores of this pathogen, the optimal management strategy for clubroot is to avoid its establishment in the soil. After establishment occurs, available tactics include cultural, biological or chemical measures (Jaramillo and Díaz, 2006) aimed at reducing disease incidence and severity through lowering the inoculum potential by crop rotation, fallowing, and using susceptible weeds as trap plants (Donald and Porter, 2009) or through modifying a favorable soil acidity through liming (Howard et al., 2010). Liming consists of applying acidity-neutralizing salts (Huang et al., 2011). Dolomite (CaCO3·MgCO3) is a preferred amending salt, also being a source of calcium (21.6%) and magnesium (13.1%). The origins of Brassica oleracea cultivars date back to the wild cabbage that is present today in the calcareous soils of the European Mediterranean and on the limestone cliffs on both sides of the British Channel or Canal de la Mancha. Núñez (2000) pointed out that this origin circumstance may explain the poor performance of cruciferous plants when grown in acid soils. The balance is even more disadvantageous for the plants in their interaction with P. brassicae, since the pathogen is favored by acid soils (Strelkov et al., 2006; Dixon, 2007).
Microbial antagonism is well documented and the genus Trichoderma has been particularly studied. Radheshyam et al. (2012) reviewed the different mechanisms used by species of this genus as biocontrol agents for soil-borne pathogens. Different Trichoderma isolates have been found to be antagonistic to fungi, such as Fusarium oxysporum f.sp. dianthi, inhibiting its mycelia growth by 89% (Márquez et al., 2002). Moreno et al. (2009) found that Trichoderma koningiopsis significantly reduced stem colonization by Fusarium oxysporum f. sp. radicis-lycopersici. Also, was also found to T. koningii stimulates the germination of tomato seeds, promoting chitinolytic enzymes in seeds implicated in plant protection against Rhizoctonia solani and Fusarium oxysporum f.sp. lycopersici (Clavijo and Cotes, 1998). Isolates of different Trichoderma species have been assessed for their efficacy against plasmodiophorids in tomatoes (Nielsen and Larsen, 2004) and potatoes (Hoyos-Carvajal et al., 2008). Researchers from New Zealand (Cheah and Page, 1997), China (Wang et al., 2011) and the Philippines (Cuevas et al., 2011) have successfully used different isolates of Trichoderma against P. brassicae. In the Philippines, Cuevas et al. (2011) assessed the economic impact of a technological package consisting of the application of Trichoderma isolates together with liming and fertilization.
Samuels et al. (2006) acknowledged the existence of a species complex around T. koningii. Within this complex, T. koningiopsis appears with marked differential characteristics, such as having a mostly tropical distribution, greater heterogeneity in molecular markers and high potential as a biocontrol agent.
A Colombian isolate of T. koningiopsis, named Th003, has successfully reduced the incidence of plant diseases caused by fungi of the genera Fusarium and Rhizoctonia in tomatoes and potatoes; the best commercial formulation is a dry wettable powder with a shelf-life between 13.9 and 14.4 months at 8 and 18°C, respectively (Santos et al., 2012).
This study was carried out on the grounds of the commercial availability of isolate Th003 of T. koningiopsis as Tricotec® and previous knowledge of the adverse effect of liming on P. brassicae, with the purpose of assessing the effectiveness of these practices independently and jointly in reducing disease levels, with the view of recovering land for the production of cruciferous crops and improving the profitability of these crops in infected fields.
Materials and methods
This study focused on the efficacy of isolate Th003 of T. koningiopsis (experimental product formulated by Corpoica, Tricotec®) and dolomite as soil amendments in the management of the clubroot disease of cabbage (B. oleracea v. capitata), caused by P. brassicae. Specifically, it aimed to assess the effects of applying Tricotec® fortnightly since planting until 15 days after transplanting (dat) in field at 109conidium/g and dolomite at 1 and 2.1 t ha-1 one month before transplanting on plant, disease and soil variables. The plant variables included phenological data and fresh and dry weight at harvest; the disease expression was assessed as severity and incidence; the soil data were pH evolution and Trichoderma quantification. The research was conducted from October 2012 to January 2013 in the Marengo Research Center (MRC) of the Universidad Nacional de Colombia, using the Valverde F1 hybrid from Emerald seeds, an accession of Ox Heart cabbage that is highly susceptible to clubroot.
Thirty-day-old seedlings that had been produced on sterile peat in a glasshouse, with daily watering and fertilization using Hoagland solution, were transplanted to a field plot with a history of clubroot. The soil was an Oxicdystropept, loam, with a pH of 5.9, an OC of 3.62% and a CIC of 29.9. The land used for this research was divided into 30, 10 m2 plots to accommodate six treatments with five replications. Forty-eight seedlings were planted in each plot with distances of 40 cm between the plants and 60 cm between the rows for a density of 4.8 plants/m.
The treatments were: control, no dolomite and no Tricotec®; 1.0tDol, 1.0 t ha-1 dolomite; 2.1tDol, 2.1 t ha-1 dolomite); Tricotec, three fortnightly applications of Tricotec® at 200 g ha-1; 1.0tDol+Tricotec, 1.0 t ha-1 dolomite and three fortnightly applications of Tricotec® at 200 g ha-1; 2.1tDol+Tricotec, 2.1 t ha-1 dolomite and three fortnightly applications of Tricotec® at 200 g ha-1. The dolomite was incorporated with one rotovator pass. The first two applications of Tricotec® were done by injecting 5 mL of the suspension directly into the substrate of each seedling. The third application was done as a drench 15 dat to the field using a knapsack without a nozzle and with a discharge of 94 mL s-1 and water at the rate of 200 L ha-1, between 07:00 and 08:00 AM.
The experimental design used a randomized complete block, following the moisture gradient of the lot. A Principal Components Analysis (PCA) was used to identify the plant phenology and disease variables that contributed most to the data variation. The selected components and associated variables were then subjected to a Friedman non-parametric analysis and, when treatment effects were detected, Tukey's test applied to the range of variables, P=0.05, to assess the differences. Given the type of analyses used, the results and graphs corresponded to the medians of each variable or the ranked values for each sampling time and treatment.
Data were collected at seven fortnightly sampling dates beginning at 15 dat to cover the extent of the crop cycle. Unless indicated otherwise, the following response variables were assessed on five plants of each plot for a total of 150 plants per sampling date.
Disease incidence. This variable was the number of diseased plants out of the five sampled and was expressed as a percentage.
Disease severity. A disease severity index (DSI) that was calculated as suggested by Wang et al. (2012), based on their severity scale (Fig. 1).
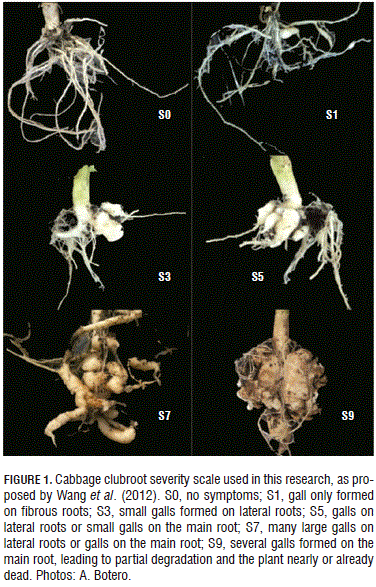
DSI = S (severity class × no. of plants in the class) × 100 / (total no. of plants in the rep) × 9 (1)
This response variable was documented with a photograph of each observed plant.
Plant height. Measure of the length from stem base to tip of the plant.
Fresh weight of the root and aerial part of the plant. This variable was measured at every sampling date.
Dry weight of the root and aerial part of the plant. This variable was measured after drying the plant material at 70°C for 7 d.
Fresh weight/dry weight ratio for the root. This ratio was calculated from the primary data of the dry and fresh weights.
Evolution of soil pH. This variable was measured in soil samples extracted from each plot after liming. The 150 samples were placed in clay pots in a growth chamber at 18°C at soil capacity; the pH was measured weekly with a pH meter in a dilution of soil/water = 1.
Isolation and quantification of Trichoderma. At 90 dat, samples of rhizospheric soil from each of the 150 extracted plants were taken; the 25 samples corresponding to each treatment were pooled and homogenized to obtain a larger sample of 500 g, which was kept in plastic bags at 4°C for 2 d. A subsample of 10 g was taken from each of the six samples, placed in 90 mL of distilled sterile water and shaken for 25 min to follow the standard suspension procedure, 1:10. An aliquot of 1 mL was suspended in a test tube with 9 mL of distilled sterile water. This step was repeated consecutively four times to reach a soil: water ratio of 1:10,000 (w:v). Three aliquots of 50 mL each were taken from the last suspension and placed in separate Rose Bengal Agar plates, a selective medium for Trichoderma.
Plates were incubated in a growth chamber for fungi (Lab-line ® Biotronette Plant Growth Biochamber, Lab-line Instruments, Melrose Park, IL) at 22±4°C. Trichoderma colonies were identified by their typical green sporulation (Barnett and Hunter, 1972; Bissett, 1991, quoted by Hoyos-Carvajal et al., 2009). The quantification was expressed as colony forming units per gram of soil (cfu/g).
Results
The PCA identified three factors that together accumulated 85.8% of the total variation and an Eigen value of 1.56. Total dry weight and aerial dry weight were the variables associated with factor 1, with 44.4% of the total variation and an Eigen value greater than 0.4. The second factor gathered 29% of the variation and the associated variables were fresh and dry weights of the root system, with an Eigen value greater than 0.4. Finally, the third factor, with 12.4% of the total variation, was associated with the fresh weight/dry weight ratio of the roots, with an Eigen value of 0.52. Following these guidelines, a Friedman test was done for the following variables: disease incidence and severity, total and aerial dry weights and the ratio of fresh weight/dry weight of the roots. Plant height was not considered since the associated factor in the PCA did not have sufficient weight.
Incidence. Clubroot first appeared at 47 dat in all of the treatments with an average incidence of 34%, which increased gradually to reach 50% at 71 dat. Differences in the disease incidence among the treatments were observed from 71 d onwards. At 71 dat, control and 2.1tDol+Tricotec had the highest incidence, 1.0tDol, 2.1tDol and 1.0tDol+Tricotec had intermediate values and Tricotec had the lowest incidence, P=0.0174 (Fig. 2). Differences in the incidence were observed up to the last observation date, 99 dat, when control and 2.1tDol+Tricotec retained the top incidence values and 2.1tDol moved from an intermediate value to the lowest incidence, P=0.0144 (Fig. 2).
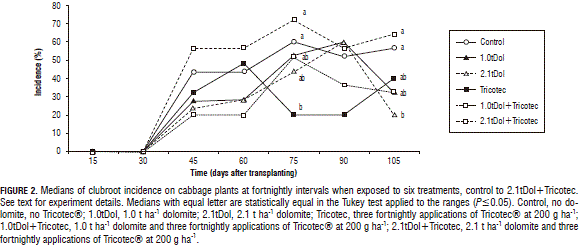
Severity. Intermediate DSI values were associated with control, while the highest value (59.5) occurred in 2.1tDol+Tricotec (Fig. 3) at 75 dat. Significantly different DSIs were observed at 105 dat (P=0.0598); the range comparison using Tukey's test indicated that the higher DSIs were found in control and 2.1tDol+Tricotec, intermediate values in 1.0tDol, Tricotec and 1.0tDol+Tricotec, and the lower ones in 2.1tDol.
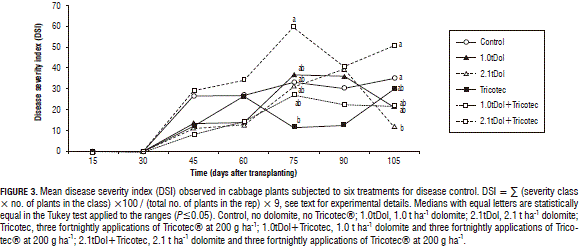
Aerial dry weight. Friedman's test revealed significant treatment effects, P=0.0203. Tricotec had the heaviest weight at harvest, 1.0tDol+Tricotec and 2.1tDol+Tricotec had the lowest, and control, 1.0tDol and 2.1tDol had intermediate values (Tab. 1).
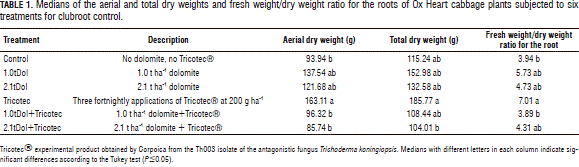
Total dry weight. Again, Friedman's test showed significant treatment effects, P=0.0382. Tricotec had the heaviest weight, 2.1tDol+Tricotec the smallest, and those for the remaining three treatments were intermediate (Tab. 1).
Fresh weight/dry weight ratio for the root. Friedman's test showed significant treatment effects at P=0.0408. Tricotec had the highest ratio; control and 1.0tDol+Tricotec the lowest and 1.0tDol, 2.1tDol and 2.1tDol+Tricotec were intermediate.
Evolution of soil pH. This variable was examined at 73 and 97 dat, when the treatments had effects on the clubroot expression. Friedman's test detected significant differences among the treatments at both dates, with P=0.0198 and P=0.0436, respectively, when the highest pH was obtained with 1.0tDol+Tricotec and the lowest with control (Tab. 2).
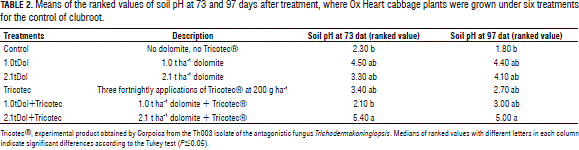
Isolation and quantification of Trichoderma. No significant differences were detected among treatments by Friedman's test in the number of cfus, P=0.2995. Nonetheless, Trichoderma appeared in two out of the three agar plates corresponding to the plots treated with Tricotec®, as compared to only one plate for plots without Tricotec®.
Economic analysis. The economic analysis was based on yield projections made from the last sampling. The number of commercial heads per hectare was calculated as the percentage of compact heads that had reached growth stage 49. The price of the heads was assumed to be US$0.13 per kilogram, based on local market price at the date of harvest. Because the market in Colombia does not discriminate for head size, this price was applied to all of the heads that met the criteria of marketability, regardless of size. The highest percentage of marketable heads was obtained in Tricotec treatment (64%) while the lowest was in control (12%) and 2.1tDol+Tricotec (28%); the 1.0tDol, 2.1tDol and 1.0tDol+Tricotec treatments produced intermediate values with 48%, 52% and 40%, respectively. Production costs ranged from US$5,840.6 to US$6,341.3 per hectare (Tab. 3); the highest was for treatment 2.1tDol+Tricotec, where the increase was caused by the application of dolomite lime and Tricotec®. Profitability was calculated without taking into account the time factor and assuming that there was no opportunity cost in the investment. Considering only these elements, treatments 1.0tDol, 2.1tDol and Tricotec generated a profit, while control, 1.0tDol+Tricotec and 2.1tDol+Tricotec generated losses (Tab. 3). The best treatment considering profitability was Tricotec, with production costs of US$5,924.1 and a gross income of US$10,900, which produced a gain of US$4,976 and a yield of 84%.
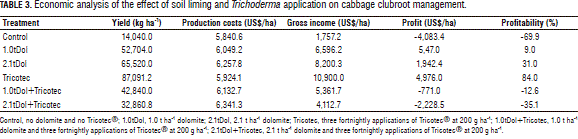
Discussion
Healthier roots that favor biomass accumulation can explain the higher total weight of the plants that received applications of Tricotec®. The favorable effect observed for Tricotec® agrees with Cheah et al. (2000), who found a 42-58% decrease in severity in plants treated with Trichoderma, as compared with non-treated plants. Field experiments conducted in the Philippines with liming and Trichoderma applications also resulted in a drastically reduced incidence of cabbage clubroot from 23% to 2% and lowered the relative costs from P4.75 to P1.50 (Philippine pesos), compared with the use of chemical pesticides, while cabbage head weights increased from 0.75 to 1.45 kg (Cuevas and Bul-long, 2009).
Besides disease reduction, the observed increase in plant weight may also have resulted from the growth promoting function that has been proposed for Trichoderma, which has been observed in oil palm (Naher et al., 2012), potted sugarcane (Naseby et al., 2000; Serna-Cock et al., 2011), soybeans (John et al., 2010) and wheat (Sharma et al., 2012).
The highest incidence and severity occurred when Tricotec® was combined with dolomite at the dose of 2.1 t ha-1. This enhancement of clubroot may have been due to the effect of the high dolomite dosage, which raised the pH from 6.2 to 6.5. In fact, although both Trichoderma and P. brassicae thrive under acidic conditions, their range overlap favors the latter; the fungus's range extends from 2.8 to 3.2 (Steyaert et al., 2010), whereas the upper values of the range for the pathogen reach from 5.5 to 6.5 and even 7.0 (Donald and Porter, 2004). We may assume that the application of dolomite at 2.1 t ha-1 went beyond the unfavorable critical pH point for Trichoderma, while remaining favorable for P. brassicae.
The soil pH had a determinant effect on the soil microbial communities, including phytopathogenic microorganisms; this effect can be attributed to the microbial physiology, soil nutrient availability and the solubility of metals. Fungal and bacterial biomass composition is directly affected by pH, as measured by Rousk et al. (2009); there is a negative correlation in the population parameters of bacteria and fungi that varies with the pH. A possible mechanism to explain the negative correlation between bacterial growth and fungal growth along a pH gradient is that a low pH is physiologically disadvantageous to bacteria, decreasing bacterial competition and thus favoring fungal growth. Besides, bacterial physiology is adapted to a narrower pH range than fungi. At a lower pH, bacterial population composition is reduced and increased at a higher pH, affecting the population and composition of fungi under a more competitive environment. In agreement with these investigations, Steyaert et al. (2010) found that conidiation of three Trichoderma species was favored by a low pH, finding its optimal pH between 2.8 and 3.2. Studies made by Hamzah et al. (2012) found that Trichoderma virens grew at a pH between 5.0 and 7.0, having its best growth rate at pH 5.5. In research by Colussi et al. (2012) and Xiong et al. (2004), it was found that the metabolic activity of this fungus increased at a low pH.
Soil pH had a determinant effect on the biology of the soil inhabiting or visiting pathogenic fungi. At the molecular level, low pH values activate the transcription factor PacC, which is responsible for regulating gene expression in conidial production and germination of Magnaporthe oryzae (Landraud et al., 2013). Steyaert et al. (2010) studied the effects of the environmental pH on the conidial production of T. hamatum, T. atroviride and T. pleuroticola; they found that sporulation is strictly dependent on pH in buffered media, but that dependence is relaxed in non-buffered media with sporulation occurring throughout the entire range of pH conditions. This dependence on buffering was an unexpected result since the hypothesis was that PacC regulated the conidial production. These results underline the complexity of pH effects on Trichoderma and bring about the need to study them in a wide spectrum of species (Steyaert et al., 2010).
Liming alone did not result in effective clubroot control because raising the pH to between 6.69 and 6.73 was not sufficient to inhibit the pathogen. Donald and Porter (2004, 2009) found that an appropriate control of P. brassicae requires a pH higher than 7.2. The moderate severity in the untreated plants (control) was likely due to the homeostatic properties of edaphic microbiomes that keep population densities of P. brassicae in check. The moderate severity observed when combining the low dosage of dolomite with applications of Tricotec® (1.0tDol+Tricotec) may be explained by the fact that the minor change in the pH was innocuous for Trichoderma and somewhat restrictive for the pathogen.
The plants treated with Tricotec® reached commercial maturity one week earlier than the rest of the plants, which represents an advantage for the grower as it reduces labor costs. Overall, the results led to the conclusion that Tricotec®, at the dosage and frequencies used in the study, is an efficient biological product in managing cabbage clubroot because it reduced the incidence and severity levels, increased the head size and proportion of marketable heads and induced early harvesting. It is necessary to explore the response of soil pH to different liming salts and dosages to ensure that any changes in pH remain within favorable limits for this biocontrol agent.
Literature cited
Barnett, H.L. and B.B. Hunter. 1972. Illustrated genera of imperfect fungi. 3rd ed. Burgess Publishing Company, Minneapolis, MN. [ Links ]
Bissett, J. 1991. A revision of the genus Trichoderma. II. Infrageneric classification. Can. J. Bot. 69, 2357-2372. Doi: 10.1139/b91-297 [ Links ]
Cheah, L.-H. and B.B.C. Page. 1997. Trichoderma spp. for potential biocontrol of clubroot of vegetable brassicas. pp. 150-153. In: Proc. 50th New Zealand Plant Protection Conf. of New Zealand Plant Protection Society; Manaaki Whenua Press, Lincoln, New Zealand. [ Links ]
Cheah, L.-H., S. Veerakone, and G. Kent. 2000. Biological control of clubroot on cauliflower with Trichoderma and Streptomyces spp. N. Z. Plant Protection 53, 18-21. [ Links ]
Clavijo, A. and A.M. Cotes 1998. Evaluación de la actividad quitinasa en procesos de control biológico de Rhizoctonia solani y Fusarium oxysporum f. sp. lycopersici en tomate, mediante fitoinvigorización de semillas en presencia de Trichoderma koningii. Rev. Colom. Biotecnol. 1, 58-66. [ Links ]
Colussi, F., W. Garcia, F.R. Rosseto, B.L.S. de Mello, M. de Oliveira Neto, and I. Polikarpov. 2012. Effect of pH and temperature on the global compactness, structure, and activity of cellobiohydrolase Cel7A from Trichoderma harzianum. Eur. Biophys. J. 41, 89-98. Doi: 10.1007/s00249-011-0762-8 [ Links ]
Cuevas, V.C. and M.S. Bul-long. 2009. Yield, production cost and incidence of club root disease of crucifers under soil fertility management practices using various combinations of soil additives. Philipp. Agric. Sci. 92, 398-406. [ Links ]
Cuevas, V.C., C.A. Lagman Jr., and A.C. Cuevas. 2011. Potential impacts of the use of Trichoderma spp. on farmers' profit in the field control of club root disease of crucifers caused by Plasmodiophora brassicae Wor. Philipp. Agric. Sci. 94, 171-178. [ Links ]
Dixon, G.R. 2007. Clubroot. pp. 25-28. In: Rimmer, S.R., V.I. Shattuck, and L. Buchwaldt (eds.). Compendium of brassica diseases. APS Press, St. Paul, MN. [ Links ]
Dixon, G.R. 2009. The occurrence and economic impact of Plasmodiophora brassicae and clubroot disease. J. Plant Growth Regul. 28, 194-202. Doi: 10.1007/s00344-009-9090-y [ Links ]
Donald, E.C. and I.J. Porter. 2004. A sand - solution culture technique used to observe the effect of calcium and pH on root hair and cortical stages of infection by Plasmodiophora brassicae. Australas. Plant Path. 33, 585-589. Doi: 10.1071/AP04068 [ Links ]
Donald, E.C. and Porter, I.J. 2009. Integrated control of clubroot. J. Plant Growth Regul. 28, 289-303. Doi: 10.1007/s00344-009-9094-7 [ Links ]
Hamzah, A., M.A. Zarin, A.A. Hamid, O. Omar, and S. Senafi. 2012. Optimal physical and nutrient parameters for growth of Trichoderma virens UKPM-1M for heavy crude oil degradation. Sains Malays. 4, 71-79. [ Links ]
Hoyos-Carvajal, L., S. Jaramillo-Villegas, and S. Orduz-Peralta, 2008. Evaluación de Trichoderma asperellum como bioregulador de Spongospora subterranea f. sp. subterranea. Rev. Fac. Nal. Agr. Medellín 61, 4496-4502. [ Links ]
Hoyos-Carvajal, L., S. Orduz, and J. Bissett. 2009. Genetic and metabolic biodiversity of Trichoderma from Colombia and adjacent neotropic regions. Fungal Genet. Biol. 46, 615-631. Doi: 10.1016/j.fgb.2009.04.006 [ Links ]
Howard, R.J., S.E. Strelkov, and M.V. Harding. 2010. Clubroot of cruciferous crops - new perspectives on an old disease. Can. J. Plant Pathol. 32, 43-57. Doi: 10.1080/07060661003621761 [ Links ]
Huang, P.M., Y. Li, and M.E. Sumner. 2011. Handbook of soil sciences: resource management and environmental impacts. 2nd ed. CRC Press Taylor & Francis, Boca Raton, FL. [ Links ]
Jaramillo N., J.E. and C.A. Díaz D. 2006. El cultivo de las crucíferas: brócoli, coliflor, repollo, col china. Technical Manual No. 20. Corpoica, Rionegro, Colombia. [ Links ]
John, R.P., R.D. Tyagi, D. Prévost, S.K. Brar, S. Pouleur, and R.Y. Surampalli. 2010. Mycoparasitic Trichoderma viride as a biocontrol agent against Fusarium oxysporum f. sp. adzuki and Pythium arrhenomanes and as a growth promoter soybean. Crop Prot. 29, 1452-1459. Doi: 10.1016/j.cropro.2010.08.004 [ Links ]
Kageyama, K. and T. Asano. 2009. Life cycle of Plasmodiophora brassicae. J. Plant Growth Regul. 28, 203-211. Doi: 10.1007/s00344-009-9101-z [ Links ]
Landraud, P., S. Chuzeville, G. Billon-Grande, N. Poussereau, and C. Bruel. 2013. Adaptation to pH and role of PacC in the rice blast fungus Magnaporthe oryzae. PLoS ONE 8, e69236. Doi: 10.1371/journal.pone.0069236 [ Links ]
Ludwig-Müller, J., B. Schubert, K. Pieper, S. Ihmig, and W. Hilgenberg. 1997. Glucosinolate content in susceptible and resistant chinese cabbage varieties during development of clubroot disease. Phytochemistry 44, 407-414. Doi: 10.1016/S0031-9422(96)00498-0 [ Links ]
Ludwig-Müller, J. and A. Schuller. 2008. What can we learn from clubroots: alterations in host roots and hormone homeostasis caused by Plasmodiophora brassicae. Eur. J. Plant Pathol. 121, 291-302. Doi: http://dx.doi.org/10.1007/s10658-007-9237-2 [ Links ]
Ludwig-Müller, J., E. Prinsen, S.A. Rolfe, and J.D. Scholes. 2009.Metabolism and plant hormone action during clubroot disease. J. Plant Growth Regul. 28, 229-244. Doi: 10.1007/s00344-009-9089-4 [ Links ]
Márquez, M., M.M. Martínez, and M. Franco. 2002. Aislamiento de Trichoderma sp. y actinomycetes a partir de suelos de clavel (Dianthus caryophyllus) y evaluación de su capacidad antagonica in vitro sobre Fusarium oxysporum f. sp. Dianthi. Agron. Colomb. 19, 81-87. [ Links ]
Moreno, C.A., F. Castillo, A. González, D. Bernal, Y. Jaimes, M. Chaparro, C. González, F. Rodríguez, S. Restrepo, and A.M. Cotes. 2009. Biological and molecular characterization of the response of tomato plants treated with Trichoderma koningiopsis. Physiol. Mol. Plant Pathol. 74, 111-120. Doi: 10.1016/j.pmpp.2009.10.001 [ Links ]
Naher, L., S.G. Tan, U.K. Yusuf, C-L. Ho, and F. Abdullah. 2012. Biocontrol agent Trichoderma harzianum strain FA 1132 as an enhancer of oil palm growth. Pertanika J. Trop. Agric. Sci. 35, 173-182. [ Links ]
Naseby, D.C., J.A. Pascual, and J.M. Lynch. 2000. Effect of biocontrol strains of Trichoderma on plant growth, Phytium ultimum populations, soil microbial communities and soil enzyme activities. J. Appl. Microbiol. 88, 161-169. Doi: 10.1046/j.1365-2672.2000.00939.x [ Links ]
Nielsen, S. and J. Larsen. 2004. Two Trichoderma harzianum-based bio-control agents reduce tomato root infection with Spongospora subterranea (Wallr.) Lagerh., f. sp. subterranea, the vector of Potato mop-top virus. J. Plant Dis. Protect. 111, 145-150. [ Links ]
Nikolaev, S.I., C. Berney, J.F. Fahrni, I. Bolivar, S. Polet, A.P. Mylnikov, V.V. Aleshin, N.B. Petrov, and J. Pawlowski. 2004. The twilight of the Heliozoa and rise of the Rhizaria, an emerging supergroup of amoeboid eukaryotes. Proc. Natl. Acad. Sci. U.S.A. 101, 8066-8071. Doi: 10.1073/pnas.0308602101 [ Links ]
Núñez S., J. 2000. Fundamentos de edafología. 2nd ed. Editorial EUNED, San Jose. [ Links ]
Radheshyam, S., A. Joshi, and R.C. Dhaker. 2012. A brief review on mechanism of Trichoderma fungus use as biological control agents. Intl. J. Innov. Bio-Sci. 2, 200-210. [ Links ]
Rousk, J., E. Bååth, P.C. Brookes, C.L. Lauber, C. Lozupone, J.G. Caporaso, R. Knight, and N. Fierer. 2010. Soil bacterial and fungal communities across a pH gradient in an arable soil. ISME J. 4, 1340-1351. Doi: 10.1038/ismej.2010.58 [ Links ]
Samuels, G.J., S.L. Dodd, B.-S. Lu, O. Petrini, H-J. Schroers, and I.S. Druzhinina. 2006. The Trichoderma koningii aggregate species. Stud. Micol. 56, 67-133. Doi: 10.3114/sim.2006.56.03 [ Links ]
Santos, A., M. García, A.M. Cotes, and L. Villamizar. 2012. The effect of the formulation on the shelf-life of biopesticides based on two Colombian isolates of Trichoderma koningiopsis Th003 and Trichoderma asperellum Th034. Rev. Iberoam. Micol. 29, 150-156. Doi: 10.1016/j.riam.2011.11.002 [ Links ]
Serna-Cock, L., C. Arias-García, and L.J. Valencia H. 2011. Effect of biofertilization on the growth of potted sugarcane plants (Saccharum officinarum). Rev. Bio. Agro. 9, 85-95. [ Links ]
Sharma, P., A.N. Patel, M.K. Saini, and S. Deep. 2012. Field demonstration of Trichoderma harzianum as a plant growth promoter in wheat (Triticum aestivum L.). J. Agr. Sci. 4, 65-73. Doi: 10.5539/jas.v4n8p65 [ Links ]
Steyaert, J.M., R.J. Weld, and A. Stewart. 2010. Ambient pH intrinsically influences Trichoderma conidiation and colony morphology. Fungal Biol. 114, 198-208. Doi: 10.1016/j.funbio.2009.12.004 [ Links ]
Strelkov, S.E, J.P. Tewari, and E. Smith-Degenhardt. 2006. Characterization of Plasmodiophora brassicae populations from Alberta, Canada. Can. J. Plant Pathol. 28, 467-474. Doi: 10.1080/07060660609507321 [ Links ]
Tamayo M., P.J. and J.E. Jaramillo N. 2004. Enfermedades de las crucíferas en Colombia, guía para su diagnóstico y manejo. Tech. Bull. No. 24. Corpoica, Rionegro, Colombia. [ Links ]
Wallenhammar, A.-C. 1996. Prevalence of Plasmodiophora brassicae in a spring oilseed rape growing area in central Sweden and factors influencing soil infestation levels. Plant Pathol. 45, 710-719. Doi: 10.1046/j.1365-3059.1996.d01-173.x [ Links ]
Wang, J., Y. Huang, Y-I. Peng, L. Zhao, H. Xiong, and Y. Qin. 2011. Suppression of clubroot on Chinese cabbage and oilseed rape by Trichoderma spp. in China. pp. 56-58. In: International Conference on Agricultural and Biosystems Engineering. Advances in Biomedical Engineering. Vol. 1-2. Hong Kong, China. [ Links ]
Wang, J., Y. Huang, S. Lin, F. Liu, Q. Song, Y. Peng, and L. Zhao. 2012. A strain of Streptomyces griseoruber isolated from rhizospheric soil Chinese cabbage as antagonist to Plasmodiophora brassicae. Ann. Microbiol. 62, 247-253. Doi: 10.1007/s13213-011-0253-2 [ Links ]
Xiong, H., N. Von Weymarn, M. Leisola, and O. Turunen. 2004. Influence of pH on the production of xylanases by Trichoderma reesei Rut C-30. Process Biochem. 39, 731-736. Doi: 10.1016/S0032-9592(03)00178-X [ Links ]