Serviços Personalizados
Journal
Artigo
Indicadores
-
Citado por SciELO
-
Acessos
Links relacionados
-
Citado por Google
-
Similares em SciELO
-
Similares em Google
Compartilhar
Agronomía Colombiana
versão impressa ISSN 0120-9965
Agron. colomb. vol.33 no.1 Bogotá jan./abr. 2015
https://doi.org/10.15446/agron.colomb.v33n1.49245
Doi: 10.15446/agron.colomb.v33n1.49245
1 Department of Civil and Agricultural Engineering, Faculty of Engineering, Universidad Nacional de Colombia. Bogota (Colombia). jhcamachot@unal.edu.co
2 Institute of Terrestrial Ecosystems, Swiss Federal Institute of Technology (ETH); Swiss Federal Institute for Forest, Snow and Landscape Research. Zurich and Birmensdorf (Switzerland)
Received for publication: 17 February, 2015. Accepted for publication: 30 March, 2015.
ABSTRACT
Techniques such as mid-infrared (MIR) spectroscopy can be used to identify the classes and properties of soils with high precision. In the present study, 312 soil samples of different orders, which were representative of Colombian agricultural zones, were studied, including Inceptisols and Vertisols of the Sinú valley (Córdoba), Andisols of the Eastern Andes Mountain range (Cundinamarca), and Oxisols of the Eastern plains of Colombia (Meta), with the aim of evaluating the ability of mid-infrared spectroscopy to estimate soil properties such as organic carbon, Ca, Mg, Na, K, P, Al, and pH. The results obtained through the analysis of the curves led to the observation that the greatest number of properties are reflected in the spectral region between 400 and 850 cm-1. In the analysis through infrared MIR spectroscopy, the spectrum for the three soils were manifested in similar spectral regions, but with different levels of reflectance, mainly due to the content of MO. In the descriptive analysis, the observed values showed a behavior similar to the values predicted with the models for those properties with representative spectral models. The majority of the properties did not show a normal distribution and the coefficients of variation were very high.
Key words: soils analysis, spectroscopy, pedometrics, modeling.
RESUMEN
Técnicas como la espectroscopia en el infrarrojo medio (MIR) pueden ser utilizadas para identificar las clases y propiedades de suelos con gran precisión. En el presente estudio se analizaron 312 muestras de suelos de diferentes órdenes, representativos de zonas agrícolas de Colombia, entre los que se encuentran Inceptisoles y Vertisoles del valle del Sinú (Córdoba), Andisoles de la cordillera oriental (Cundinamarca) y Oxisoles de la altillanura Colombiana (Meta), con el objetivo de evaluar la capacidad de la espectroscopía de infrarrojo medio para la estimación de propiedades del suelos como carbono orgánico, Ca, Mg, Na, K, P, Al y pH. Los resultados obtenidos en el análisis de las curvas, permiten observar que el mayor número de atributos están reflejados en la región espectral comprendida entre 400 y 850 cm-1. En el análisis obtenido por medio de la espectroscopia MIR, las tres clases de suelos presentaron regiones similares del espectro, pero con diferentes niveles de reflectancia, debido principalmente a los contenidos de MO. En el análisis descriptivo, los valores observados presentaron un comportamiento similar a los valores predichos a partir de los modelos, para aquellas propiedades con modelos espectrales representativos. La mayoría de las propiedades no presentaron una distribución normal y los coeficientes de variación fueron muy altos.
Palabras clave: análisis de suelos, espectroscopia, pedometría, modelamiento.
Introduction
Across time, man has used different methods of soil evaluation and quantification of the elements found in soil. This has been done through field evaluations and laboratory analysis in order to know and evaluate the quality of the soil, a procedure that in most cases is expensive and lengthy and requires a large amount of investment (Ge et al., 2011). Furthermore, the use of chemical reactives for laboratory analyses generates residues, which cause environmental contamination (Zornoza et al., 2008) because some of the consumables used are corrosive, toxic, and flammable and they even represent biological and infectious risks for living beings (Viscarra-Rossel et al., 2006).
Diffuse reflectance spectroscopy is a method that can be used to support or replace conventional methods of soil analysis. This technique has undergone a great deal of development during the last two decades, overcoming some limitations and acquiring a greater diversity of robust statistical methodologies that are able to more precisely relate the variability of the spectrum to the variability of the characteristics of the soil.
Soil spectroscopy is fast and convenient, less expensive, non-destructive, simple, and, on occasion, more precise than conventional analysis, intensified with the use of other techniques, such as multivariate statistics and geostatistics (Tittonell et al., 2008). The techniques involved in the use of soil spectroscopy can be adjusted for use by researchers, technicians, and farmers, thus supplying an adequate alternative for the study of the spatial variability of soil properties, as well as the characteristics and development of crops.
At present, infrared (IR) spectroscopy can be summarized as spectra, which, afterwards, are interrelated by means of calibration models on the basis of the behavior of the properties of interest in samples and with the obtained data and a mathematical model is established, which is used for the prediction of the properties of the samples that were not used for the calibration of the model (Cobo et al., 2010). This is a non-destructive, quick technique. Only one spectrum allows for the simultaneous establishment of multiple physical properties of crops and biochemical properties of soils (Cozzolino and Morón, 2006). It is also less expensive than conventional laboratory analyses, especially when it is necessary to analyze a large number of samples (Viscarra-Rossel et al., 2006; Camacho-Tamayo et al., 2014). The IR technique has the additional advantage of being able to use the spectral information as a measure of the integration of the soil quality and, therefore, can be used as a tool for the evaluation of the conditions of the soil.
Spectroscopy in the mid-infrared (MIR) region is one of the most important analytical techniques available for gathering information about qualitative and quantitative aspects of analytes in real time (Fuentes et al., 2008). The range of the wavelength is between 400 and 4,000 cm-1, equivalent to a wavelength of 2.5 to 25 nm. This range is especially important because it provides direct information about the constituents of a sample, as well as its characteristic molecular structure (Etzion et al., 2004). Although NIR is most commonly used, MIR spectroscopy is becoming more common, due to the specificity of the absorption bands in this spectral range (Du et al., 2008). Linker et al. (2006) showed that mid-infrared spectroscopy can be used for identifying the types of agricultural soils associated with characteristic elements of a soil (for example, calcium carbonate minerals, clay, and organic components). Such identification of the soil types is aimed toward a significant improvement in the determination of the nitrate bases in the soil (Linker et al., 2006).
Different studies have shown how MIR sensor data models outperform those using vis-NIR. This may be explained by the fact that the fundamental molecular vibrations of soil components occur in the MIR range, while only their overtones and combinations are detected in the vis-NIR range (Viscarra-Rossel et al., 2006). The MIR multivariate calibrations are consequently more robust, which makes sense because the organic carbon and clay minerals are the principal constituents of soils and have well-defined absorption regions, particularly in the MIR range. The present research aimed to evaluate the potential of mid-infrared reflectance (MIR) spectroscopy for the determination of organic carbon, Ca, Mg, Na, K, P and Al in samples of agricultural soils of various zones in Colombia.
Materials and methods
Sampling and laboratory analysis. From 312 soil samples of different orders were analyzed, which were representative of the agricultural zones of Colombia, comprising 62 Inceptisols and Vertisols from the Sinú Valley (Córdoba), 90 Andisols from the Eastern Andes Moun-tain range (Silvania, Cundinamarca) and 160 Oxisols from the Eastern Plains of Colombia plain (Puerto López, Meta), taken from the first 20 cm. Before obtaining the spectral responses of the soil samples, they were dried at a temperature of 35°C until they reached equilibrium moisture and then they were sifted using a 2 mm mesh, to analyze the fine fraction of the soil.
In the soil samples, the organic carbon was determined through the wet extraction method (Walkley and Black + volumetry), the Ca, Mg, Na and K contents were determined through extraction with ammonium acetate and analyzed by atomic absorption spectrophotometry, the phosphorus was determined through Bray II extraction, and the Al was determined through extraction with a neutral solution of NaCl. The spectral responses were acquired by means of a Prestige 21 (Shimadzu Corporation, Kyoto, Japan) sensor, which covered a range between 2.5 and 25 mm (500 to 4,000 cm-1) in the mid-infrared (MIR) range. Three readings were carried out for each soil sample and each point of the spectral response was the result of 128 scans, using as a pattern potassium bromide (KBr).
Calibration and validation of the models. The calibration of the models was carried out by means of partial least squares regression (PLSR) (Jöreskog and Wold, 1982), a procedure that is widely used in chemometrics and that represents a better approach to quantitative models among the variables of prediction (X) and response (Y), exhibiting a higher degree of performance than multiple linear regression (MLR). For this, 260 samples were considered, leaving 52 samples for validation. The formation of each group was carried out using the conditioned Latin hypercube sampling (cLHS) method (Minasny and McBratney, 2006), which consists of selecting initial values for generating a model and stratifying the range of each of the entry data of the model in order to guarantee that initial values of each range of entry data are selected. The models were constructed with ParLeS software, developed by Viscarra-Rossel (2008). The selection of the best model was done according to the value of the residual prediction deviation (RPD), the coefficient of determination (R2), and the root-mean-square error (RMSE).
Descriptive analysis. Once the models were constructed, descriptive analysis was carried out for each property, for the data observed in the laboratory as well as that predicted from spectral models, in order to establish the similarity between them as well as the behavior and tendency of the data. The parameters calculated for the properties were mean, median, coefficient of variation (CV), minimum and maximum, standard deviation, skewness, and kurtosis. For the CV, the classification given by Pimentel-Gomes and García (2002), who considered a low variability less than 10%, CV between 10 and 30% medium variability, CV between 30 and 60% high variability, and greater than 60% very high variability. The normality was also verified by means of a Kolmogorov-Smirnov test.
Results and discussion
Analysis of the spectral responses. In the spectral curves of the analyzed soils, there were differences between the spectra (Fig. 1). On analyzing the spectra by type of soil, a greater expression in the spectral region of 466 cm-1 and 70% reflectance was observed. According to Madari et al. (2006), this region corresponds to the expression of primary minerals such as Nontronite, Moscovite, and CH groups. On observing the spectra for each soil, bands were observed, where greater expression of some properties was exhibited. It can be inferred that, for the Inceptisols and Vertisols, the spectral region of greatest relevance was between 500 and 700 cm-1, with a reflectance of 63%. According to McDowell et al. (2012), the region of the spectral signature of soils is found between 600 and 1,500 cm-1, where the greatest characteristics of the fundamental vibrations of silicate minerals in the soil are exhibited. The Oxisols as well as the Inceptisols and Vertisols showed their maximum expression in the spectral region of 466 to 680 cm-1 and 69% reflectance. In the Oxisols of Meta, the spectral characteristics caused by iron oxides had great particularity, since they caused an increase in the reflectance for short wavelengths and a diminishing of the reflectance for slightly longer wavelengths (McDowell et al., 2012). In general, highly weathered soils exhibit greater reflectance, while darker soils, generally with a greater organic matter content, exhibit smaller reflectance values (Demattê et al., 2012; Camacho-Tamayo et al., 2014).
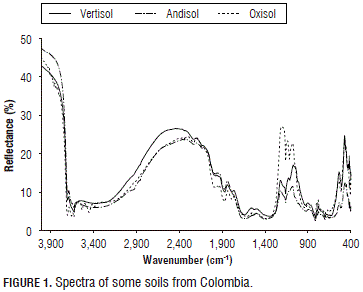
There is a strong relationship between degrees of soil weathering and spectral information. The Oxisols, developed from sediments, showed the highest reflectance intensities, with ascending spectral curves in the 900-1,400 cm-1 wave number region, contrary to the clay soils from volcanic residue in Andisols. Using the descriptive aspects of the spectra, it is possible to detect the influence of the presence of 1:1 minerals (kaolinite and gibbsite), 2:1 minerals, iron oxides (hematite and goethite) and organic matter in Andisols and Vertisols, components that predominant these soils (Malagón, 2003).
Calibration and validation of the models. The models of calibration and validation showed the prediction potential of MIR, which varies with the soil property to be evaluated and is indicated by the fit of the different models and the coefficient of determination (Cobo et al., 2010). According to Minasny et al. (2009), for agricultural applications, values of RPD greater than 2 indicate that the models provide precise predictions, values of RPD between 1.4 and 2 are considered to be reasonably representative, and values less than 1.4 indicate poor predictive value. The soil property of best behavior was CO, with excellent values of RPD ≥ 4.14/3.26 and R2 of 0.94/0.91, results similar to those reported by Mouazen et al. (2010), followed by Ca with RPD ≥ 3.22/2.32 and R2 0.90/0.81, an excellent value according to Conzen (2003), who indicated that an R2 close to one (1) indicates the construction of good models (Tab. 1).
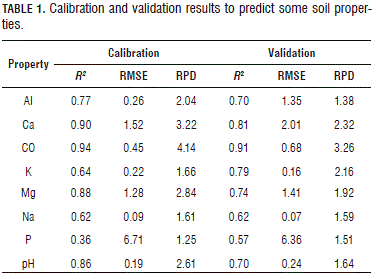
The pH, Al, Mg, K, and Na exhibited an acceptable behavior. These values coincided with those reported by Cobo et al. (2010). The soil property where the models showed poor predictions was P, with values of RPD 1.25/1.51 and R2 0.36/0.57, parameters of models that indicate a poor prediction of this property, a result similar to that found by Cobo et al. (2010).
In the crossed validation of the observed and predicted data, it was verified that the best R2 was for CO with 0.95, followed by Ca with 0.94, pH with 0.91, Al with 0.89, and Mg with 0.89 (Fig. 2), the latter cations being of great importance because they are dominant at the interchange sites. These results show that CO, Ca, Al, Mg and pH enjoy good prediction, in contrast to the properties P, K, and Na, which showed an R2 of 0.43, 0.74, and 0.69, respectively, being the lower values. Similar results were reported by Minasny et al. (2009), Reeves et al. (2001), McCarty et al. (2010), and McDowel et al. (2012). The soil properties with models of low predictive value such as Na and P can be attributed to low concentrations, at which the element is found in the soil, and for K, which also showed unreliable results, it is generally due to the fact that they are affected by their high mobility in the soil solution, which easily varies their content, providing less certain prediction results; nevertheless, similar results were reported by Cobo et al. (2010) in another soil class. Other causes of low representative models for these properties include the absence of sensitivity in routine analyses that are employed in laboratories and errors in the execution of routines (Cantarella et al., 2006).
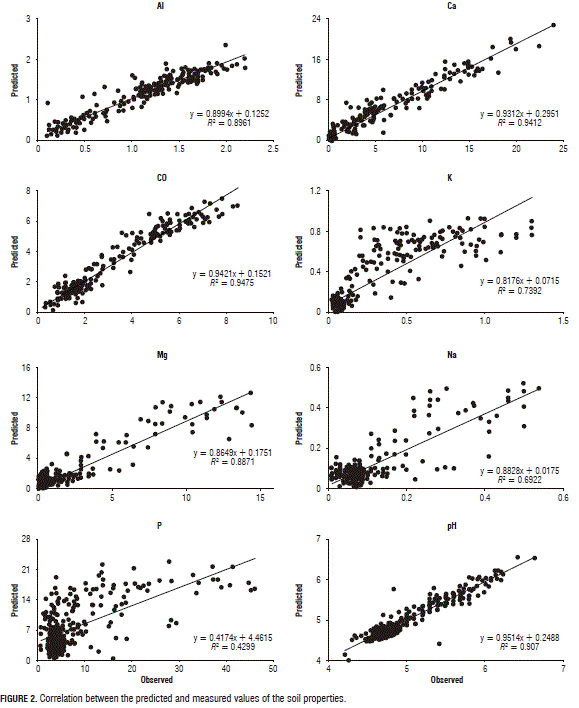
Descriptive analysis. The Al presented contents that varied from 0.10 to 5.40 cmolc kg-1 with a mean lower than 1.2 cmolc kg-1 (Tab. 2). This value is similar to that reported by Casierra-Posada and Aguilar-Avendaño (2007) for the concentration of Al3+ in a soil solution when a pH less than 5.0 was exhibited, where Al was found in the range of 0.1 to 1.0 cmolc kg-1. With these contents, this property is the principal limiting factor for agricultural production and should be taken into account for the management of soils in the production of crops (Camacho-Tamayo et al., 2010).
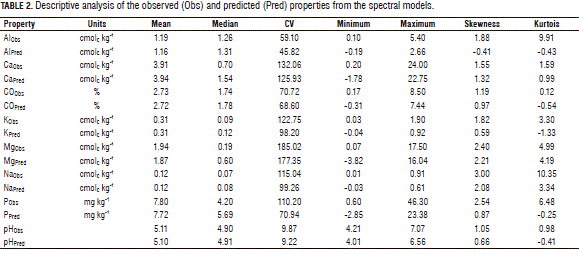
The Ca and Mg exhibited maximum contents of 24 and 17 cmolc kg-1 and minimum contnents of 0.2 and 0.07 cmolc kg-1, respectively. It can be affirmed that, of the analyzed properties, the soils exhibited the highest contents of Ca and Mg (Martins et al., 2011). Habitually, these contents in the soil depend on the materials of origin, the degree of weathering, and the lixiviation process and, thus, their contents can vary widely in soils. It is of note that Ca and Mg are better expressed in soil solutions when the pH is above neutrality, in soils that are moderately alkaline or alkaline. The coefficients of variation for the Ca and Mg were high and did not show a normal distribution (P>0.05).
The CO contents varied from 0.17 to 8.5%, with a very high coefficient of variation. It can be said that the low contents of this attribute belonged to the Oxisols and Inceptisols and the high ones to the Andisols. According to Carvajal et al. (2009), variations of CO are exhibited depending on the altitude, even with the same land use. The mean of the CO had a value of 2.73%. According to this, it can be said that the CO contents in various Colombian soils are of medium values. The total CO is an indicator of the effect of different productive systems on the organic fraction of the soil and is used to evaluate the changes in MO associated with the labor systems, use, and productive capacity of the soils (Martínez et al., 2008), because of which it is inferred that, in Colombia, the soils are being exploited with agricultural activities that, little by little, are causing the organic layer of the soil to deteriorate.
The K varied its content from 0.03 to 1.9 cmolc kg-1. The availability of this nutrient can change, depending on various factors, including the moisture of the soil (Roldán et al., 2004). It is to be expected that, in Colombia, because of its location in the Tropical Zone, the climate will be tropical and have uniform climactic conditions, but the inclusion of mountain ranges in the topography results in a variety of climates that influence the formation of soils, where the highly weathered one, such as Oxisols, contrast with the young soils, such as Inceptisols and Vertisols, which are found closer to sea level and are derived from volcanic materials, in those that the contents of clay and of K are generally high (Mengel and Kirkby, 2000). This diversifies the availability of certain properties of the soil, which is reflected in the high coefficients of variation, which, like the former properties, are high. The asymmetry and kurtosis in the predicted K were closer to zero and the K-S test did not exhibit normal distribution (P>0.05).
The Na had a minimum of 0.01 and a maximum of 0.91 cmolc kg-1, with a median of 0.07 cmolc kg-1. These contents are adequate for soils of agricultural production. Excess Na is one of the principal factors that affect plant growth. According to Muñoz et al. (2000), saline sodium soils exhibit an interchangeable concentration of sodium of 15% and a pH between 8.5 and 10.0, a situation in which the majority of crops begin to have problems in their development. In Colombia, there are zones where the soils are affected by excess Na (Laegdsmand et al., 2005) and, in the worst cases, there is incrustation caused by dispersion in kaolinitic soils under conditions of exposure to erosion (Summa et al., 2006). Otero et al. (2002) recommended special handling for these soils, above all for dispersion, which should be analyzed by ranges of indicators of electric conductivity and the relation of the activity of Na+ (Ca2+) -0.5, keeping in mind the particularities of the ionic species.
The P presented contents from 0.6 to 46.0 mg kg-1, with a mean of 7.8 mg kg-1 and high values for the coefficient of variation. These results are similar to those reported by Martins et al. (2011). As previously discussed, the principal chemical limitation in the expression of P is directly related to the pH and the content of allophane, which can be present in Andean soils and which, having an acidic tendency, can fix or immobilize up to 90% of the phosphorus that exists in the soil solution (Alcalá et al., 2002). On the other hand, according to IGAC (1988), in approximately 85% of the national territory, the soils have pH values below 5.5 and 57.6% have a pH > 5, in which case there are no great limitations on agricultural activity. It should be emphasized that the analyzed samples came from zones dedicated to farming and the corrections, performed over time, have changed the conditions of the soil. The coefficient of variation was low and the skewness and kurtosis were close to zero in the values of the measurements and estimations. This is similar to what Garzón et al. (2010) and Peña et al. (2009) reported for Colombian soils.
Conclusions
With the analysis through infrared MIR spectroscopy, the properties of the soils were manifested in similar spectral regions, but with different levels of reflectance. This difference was notable principally in the spectrum of the Andisols, where the spectral peaks were the lowest, a fact attributable to the compounds of MO, which tend to darken the soil, absorbing the infrared light, principally in the regions between 400 - 700 and 900 - 1,300 cm-1. On the other hand, the results of this paper show that, through the technique of mid-infrared (MIR) reflectance spectroscopy, a great quantity of samples can be processed, whose spectral responses provide information about various parameters in only one spectrum. In the present case, CO and Ca were those with the best prediction results, with R2 0.94 and 0.90, respectively, and RPD ≥ 3.
The quantification of the soil properties using this method allowed for a high degree of confidence in the measurements, although it could be adversely affected when the contents are low or show a small degree of variability. This quantification, with the calibration and validation of models, is less wasteful and faster and generates less environmental impacts, over conventional methods. This technique can partially replace conventional laboratory methods since it requires them to prepare models and feedback from these in time. Moreover, further studies are needed that include alternative methods of quantification of soil properties in the laboratory, which may permit an improvement of models of calibration and validation, especially for those properties whose models were not representative.
Acknowledgements
The authors thank the Direccion de Investigacion Sede Bogota (DIB; Universidad Nacional de Colombia) for the partial financial support of project code 13140.
Literature cited
Alcalá J., M., C. Ortiz S., and C.M. Gutiérrez C. 2002. Clasificación de los suelos de la Meseta Tarasca, Michoacán. Terra 19, 227-239. [ Links ]
Camacho-Tamayo, J.H., C.A. Luengas-Gómez, and F.R. Leiva. 2010. Análisis multivariado de propiedades químicas en Oxisoles con diferentes niveles de intervención agrícola. Acta Agron. 59, 273-284. [ Links ]
Camacho-Tamayo, J.H., Y. Rubiano S., and M.P. Hurtado S. 2014. Near-infrared (NIR) diffuse reflectance spectroscopy for the prediction of carbon and nitrogen in an Oxisol. Agron. Colomb. 32, 86-94. Doi: 10.15446/agron.colomb.v32n1.38967 [ Links ]
Cantarella, H., J.A. Quaggio, B. Van Raij, and M.F. Abreu. 2006. Variability of soil analysis in commercial laboratories: implications for lime and fertilizer recommendations. Commun. Soil Sci. Plant Anal. 37, 2213-2225. Doi: 10.1080/00103620600817523 [ Links ]
Carvajal, A.F., A. Feijoo, H. Quintero, and M.A. Rondón. 2009. Carbono orgánico del suelo en diferentes usos del terreno de paisajes andinos colombianos. J. Soil Sci. Plant Nutr. 9, 222-235. Doi: 10.4067/s0718-27912009000300005 [ Links ]
Casierra-Posada, F. and O. Aguilar-Avendaño. 2007. Estrés por aluminio en plantas: reacciones en el suelo, síntomas en vegetales y posibilidades de corrección. Una revisión. Rev. Colomb. Cienc. Hortic. 1, 246-257. [ Links ]
Cobo, J.G., G., Dercon, T. Yekeye, L. Chapungu, C. Kadzere, A. Murwira, R. Delve, and G. Cadisch. 2010. Integration of mid-infrared spectroscopy and geostatistics in the assessment of soil spatial variability at landscape level. Geoderma 158, 398-411. Doi: 10.1016/j.geoderma.2010.06.013 [ Links ]
Conzen, J.-P. 2003. Multivariate calibration: a practical guide for the method development in the analytical chemistry. Bruker Optik GmbH, Ettlingen, Germany. [ Links ]
Cozzolino, D. and A. Morón. 2006. Potential of near-infrared reflectance spectroscopy and chemometrics to predict soil organic carbon fractions. Soil Till. Res. 85, 78-85. Doi: 10.1016/j.still.2004.12.006 [ Links ]
Demattê, J.A.M., F.S. Terra, and C.F. Quartaroli. 2012. Spectral behavior of some modal soil profiles from São Paulo State, Brazil. Bragantia 71, 413-423. Doi: 10.1590/S0006-87052012005000038 [ Links ]
Du, C., R. Linker, and A. Shaviv. 2008. Identification of agricultural Mediterranean soils using mid-infrared photoacoustic spectroscopy. Geoderma 143, 85-90. Doi: 10.1016/j.geoderma.2007.10.012 [ Links ]
Etzion, Y., R. Linke, U. Cogan, and I. Shmulevich. 2004. Determination of protein concentration in raw milk by mid-infrared Fourier transforms infrared/attenuated total reflectance spectroscopy. J. Dairy Sci. 87, 2779-2788. [ Links ]
Fuentes N., M., C. Bosch O., and F. Sánchez S. 2008. Aplicación de la espectroscopia del infrarrojo medio en química analítica de procesos. Bol. Soc. Quím. Méx. 2 93-103. [ Links ]
Garzón G., C.A., C.A. Cortés, and J.H. Camacho-Tamayo. 2010. Variabilidad espacial de algunas propiedades químicas en un Entisol. Rev. UDCA Act. Div. Cient. 13, 87-95. [ Links ]
Ge, Y., C.L.S. Morgan, S. Grunwald, D.J. Brown, and D.V. Sarkhot. 2011. Comparison of soil reflectance spectra and calibration models obtained using multiple spectrometers. Geoderma 161, 202-211. Doi: 10.1016/j.geoderma.2010.12.020 [ Links ]
IGAC, Instituto Geográfico Agustín Codazzi. 1988. Suelos y bosques de Colombia. Bogota. [ Links ]
Jöreskog, K.G. and H. Wold. 1982. Systems under indirect observation: causality, structure, prediction, North-Holland. Elsevier, Amsterdam. [ Links ]
Laegdsmand, M., L.W. de Jonge, and P. Moldrup. 2005. Leaching of colloids and dissolved organic matter from columns packed with natural soil aggregates. Soil Sci. 170, 13-27. Doi: 10.1097/00010694-200501000-00003 [ Links ]
Linker, R., M. Weiner, I. Shmulevich, and A. Shaviv. 2006. Nitrate determination in soil pastes using attenuated total reflectance Mid-infrared spectroscopy: improved accuracy vía soil identification. Biosyst. Eng. 94, 111-118. Doi: 10.1016/j.biosystemseng.2006.01.014 [ Links ]
Madari, B.E., J.B. Reeves III, P.L.O.A. Machado, C.M. Guimarães, E. Torres, and G.W. McCarty. 2006. Mid- and near-infrared spectroscopic assessment of soil compositional parameters and structural indices in two Ferralsols. Geoderma 136, 245-259. Doi: 10.1016/j.geoderma.2006.03.026 [ Links ]
Malagón C., D. 2003. Ensayo sobre tipología de suelos colombianos - énfasis en génesis y aspectos ambientales. Rev. Acad. Colomb. Cienc. 27, 319-342. [ Links ]
Martínez H., E., J.P. Fuentes E., and E. Acevedo H. 2008. Carbono orgánico y propiedades del suelo. R.C. Suelo Nutr. Veg. 8, 68-96. [ Links ]
Martins, A.L.S., E.G. de Moura, and J.H. Camacho-Tamayo. 2011. Evaluation of corn production parameters and their spatial relationship with chemical attributes of the soil. Agron. Colomb. 29, 99-106. [ Links ]
McCarty, G.W., J.B. Reeves III, R. Yost, P.C. Doraiswamy, and M. Doumbia. 2010. Evaluation of methods for measuring soil organic carbon in West African soils. Afr. J. Agric. Res. 5, 2169-2177. [ Links ]
McDowell, M.L., G.L. Bruland, J.L. Deenik, S. Grunwald, and N.M. Knox. 2012. Soil total carbon analysis in Hawaiian soils with visible, near-infrared and mid-infrared diffuse reflectance spectroscopy. Geoderma 189-190, 312-320. Doi: 10.1016/j.geoderma.2012.06.009 [ Links ]
Mengel, K. and E.A. Kirkby. 2000. Principios de nutrición vegetal. Instituto Internacional de la Potasa. Basel, Switzerland. [ Links ]
Minasny, B. and A.B. McBratney. 2006. A conditioned Latin hypercube method for sampling in the presence of ancillary information. Comp. Geosci. 32, 1378-1388. Doi: 10.1016/j.cageo.2005.12.009 [ Links ]
Minasny, B., G. Tranter, A.B. McBratney, D. Brough, and B.W. Murphy. 2009. Regional transferability of mid-infrared diffuse reflectance spectroscopic prediction for soil chemical properties. Geoderma 153, 155-162. Doi: 10.1016/j.geoderma.2009.07.021 [ Links ]
Mouazen, A.M., B. Kuang, J. De Baerdemaeker, and H. Ramon. 2010. Comparison among principal component, partial least squares and back propagation neural network analyses for accuracy of measurement of selected soil properties with visible and near infrared spectroscopy. Geoderma 158, 23-31. Doi: 10.1016/j.geoderma.2010.03.001 [ Links ]
Muñoz, I., C.A. Mendoza, G.F. López, A.A. Soler, and M.M.M. Hernández. 2000. Manual de análisis de suelo. Edafología. Escuela Nacional de Estudios Profesionales Iztacala, UNAM, Mexico DF. [ Links ]
Otero G., J., C. Gómez, and R. Sánchez. 2002. Zonificación de los procesos de salinización de los suelos de Colombia. Instituto de Hidrología, Meteorología y Estudios Ambientales (IDEAM), Bogota. [ Links ]
Peña, R., Y. Rubiano, A. Peña, and B. Chaves. 2009. Variabilidad espacial de los atributos de la capa arable de un Inceptisol del piedemonte de la cordillera Oriental (Casanare, Colombia). Agron. Colomb. 27, 111-120. [ Links ]
Pimentel-Gomes, F. and C.H. García. 2002. Estatística aplicada a experimentos agronômicos e florestais; exposição com exemplos e orientações para uso de aplicativos. Fundação de Estudos Agrários Luiz de Queiroz, Piracicaba, Brazil. [ Links ]
Reeves III, J.B., G.W. McCarty, and V.B. Reeves. 2001. Mid-Infrared diffuse reflectance spectroscopy for the quantitative analysis of agricultural soils. J. Agric. Food Chem. 49, 766-772. Doi: 10.1021/jf0011283 [ Links ]
Roldán, M.F., C.A. Venialgo, and N.C. Gutiérrez. 2004. Potasio disponible, de reserva y energía de reemplazamiento en suelos y el nivel foliar en rye-grass. Universidad Nacional del Nordeste, Comunicaciones Científicas y Tecnológicas A-072. [ Links ]
Summa, V., F. Tateo, L. Medici, and M.L. Giannossi. 2006. The role of mineralogy, geochemistry and grain size in badland development in Pisticci (Basilicata, southern Italy). Earth Surface Processes and Landforms 32, 980-997. Doi: 10.1002/esp.1449 [ Links ]
Tittonell, P., K.D. Shepherd, B. Vanlauwe, and K.E. Giller. 2008. Unravelling the effects of soil and crop management on maize productivity in small-holder agricultural systems of western Kenya: An application of classification and regression tree analysis. Agric. Ecosyst. Environ. 123, 137-150. Doi: 10.1016/j.agee.2007.05.005 [ Links ]
Viscarra-Rossel, R.A. 2008. ParLeS: software for chemometric analysis of spectroscopic data. Chemometr. Intell. Lab. Syst. 90, 72-83. Doi: 10.1016/j.chemolab.2007.06.006 [ Links ]
Viscarra-Rossel, R.A., D.J.J. Walvoort, A.B. McBratney, L.J. Janik, and J.O. Skjemstad. 2006. Visible, near infrared or combined diffuse reflectance spectroscopy for simultaneous assessment of various soil properties. Geoderma 131, 59-75. Doi: 10.1016/j.geoderma.2005.03.007 [ Links ]
Zornoza, R., C. Guerrero, J. Mataix-Solera, K.M. Scow, V. Arcenegui, and J. Mataix-Beneyto. 2008. Near infrared spectroscopy for determination of various physical, chemical and biochemical properties in Mediterranean soils. Soil Biol. Biochem. 40, 1923-1930. Doi: 10.1016/j.soilbio.2008.04.003 [ Links ]