Introduction
Phosphorus is one of the most important nutrients contributing to plant growth (Ritcher and Rao, 2005; Steiner et al., 2007). Preliminary studies show that a majority of highly weathered tropical, equatorial, and volcano-ash derived soils suffer limitation of P which is found mostly in inert forms (Ranno et al., 2007; Quesada et al., 2011).
The occurrence of inert forms can be attributed to the ability of many soil constituents to bind P: clay minerals, iron and aluminum hydroxides, hydroxyl-Al polymers, organomineral complexes and carbonates (Gérard, 2016). These interactions with soil leads to the P being unavailable to plants, and while P is added as a fertilizer, it is transformed into forms that are minimally available to crops, even under more favorable conditions such as slightly acidic pH (Daza-Torres et al., 2008; Navarro and Navarro, 2013).
The addition of biochar to improve the physical and chemical properties of soil is an ancient practice (Denevan, 1996; Steiner et al., 2007; Gul et al., 2015). Recent studies indicated that in very low-fertility soils, such as in the South American and Amazonian savannas, the addition of biochar improves the availability of P (Lehmann et al., 2003; Steiner et al., 2007; De Luca et al., 2009; Yao et al., 2012; Siddiqui et al., 2016). However, other studies of soil columns demonstrate that the application of biochar significantly increases the retention of P in the soil (Novak et al., 2009; Zhao et al., 2016). There have been few studies on the longterm impacts of biochar amendments and how these may influence both P sorption and other soil properties (De Luca et al., 2009; Yao et al., 2012; Gul et al., 2015). Thus, the effect of the application of plant-origin carbon is variable and depends on the type of biochar used, and the reaction conditions during the process of pyrolysis and the soil type (Yao et al., 2012; Mukherjee and Zimmerman, 2013).
Eucalyptusglobulus is one of the most planted tree species in the world, mainly in temperate regions. In Colombia, it has become the second most planted tree species and principally is used in wood production. The E. globulus plantations in Colombia, although generally with good volumetric growth, are phenotypically, quite heterogeneous. In the same plantation are outstanding trees with good development and individuals of poor growth and twisted bark, among other characteristics not desirable in a commercial plantation (Sánchez-Buitrago, 2013). These no commercial trees could be employed in the production of biochar for new applications in agriculture.
The aim of this study was to evaluate the effect of adding different proportions of Eucalyptus globullus biochar to an acidic soil on the phenomena of P sorption and desorption, as well as to characterize the long-term availability of P by incubating P in different soil/biochar treatments in laboratory conditions.
Materials and methods
Soil and biochar
Soil samples were collected at the "Marengo Agricultural Center" located in the Bogota Savanna (Cundinamarca, Colombia), with an approximate altitude of 2,543 m a.s.l. According to USDA, the soil is a Fluventic Humic Dystrudepts, with a thick and dark A horizon originating in lake sediments with a sprinkling of volcanic ash and alluvial clay contributions (Malagón, 2003). A soil sample made up of 20 subsamples was taken in a 1.0 ha area, to a depth of 20 cm (in a zig-zag sampling pattern across the area). Physicochemical properties were evaluated and are shown in table 1.
TABLE 1 Physicochemical characterization of the soil and biochar.
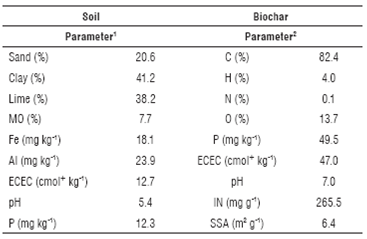
1Soil methods: Texture: Bouyoucos methocl; M0: Walkleyand Blackmethocl; pH: soil/waterratio 1:2; ECEC (effective cationic exchange capacity): neutral ammonium acétate methocl; P: Bray II; Al: KC11 M extraction; Fe: DPTA extraction Al: DPTA extraction.2The biochar characterization methods are described in detail in a prior study (Jiménez et a/., 2006). Carbon, hydrogen and nitrogen contení were determined by an elemental analyzer (LEC0 CHN-600); IN: (iodine number): Icontec NTC 4467 method; ECEC: Neutral ammonium acétate method; P: Bray II; pH: Icontec, NTC 4467 method; SSA (specific surface area): BET method.
In general, the soil presents a certain amount of fertility problems, such as acidity and the presence of excessive amounts of aluminum and iron. The pH value indicates that the soil is sufficiently acidic for this property to govern the retention of P through a combination of sorption mechanisms of clays and colloidal Fe and Al colloids (Daza-Torres et al., 2008; Navarro and Navarro, 2013; Quesada et al., 2011). The biochar used was obtained from Eucalyptus globullus, at a finally temperature of 450°C for 3 h, the pyrolysis methodology has been previously described (Jiménez et al., 2006). The main parameters of this biochar are summarized in table 1.
Before beginning the P tests, biochar particle size was reduced and filtered through a sieve below 150 µm in mesh size. Four treatments with different soil/biochar percentages and one treatment control (only soil) were prepared. The percentages of biochar in the four treatments were 5, 10, 20, and 35% w/w.
Phosphorus sorption and desorption
To obtain P-sorption isotherms, 2.5 g of each soil treatment (three repetitions) were weighed in 60 mL polyethylene bottles. Then, 25 mL P solution (KH2PO4) was added to each bottle, prepared in CaCl2 0.01 mol L-1 and agitated over 24 h. The tested concentrations were C1: 10 mg kg-1, C2: 100 mg kg -1, C3: 250 mg kg-1; C4: 500 mg kg-1; C5: 1000 mg kg-1, and a control series without added P. The normal P field application dose is between the values for C1 and C2. After the samples reached equilibrium (24 h), they were centrifuged for 10 min at 3,000 rpm, and a 1.0 mL aliquot of the clarified supernatant was taken to determine the amount of P. Inhibition of microbial growth using toluene or chloroform was not applied to any of the tests because this inhibition can increase the solubility of P via processes of cellular lysis (Reddy et al., 1998).
Once the sorption test was completed, the supernatant was removed via filtration, and the solid remainders were extracted using 20 mL Bray II solution (Gómez and Rojas, 2006) and filtered again using Whatman paper No 42. The extraction process was repeated sequentially eight times for each concentration level and for each soil/biochar mix. This number of extractions was selected based on prior work on the same soils using these treatments.
The SigmaPlot 12.0 program (Systat Software, San Jose, CA, USA) was used to fit the sorption isotherms to the Langmuir equation (1), which has been used successfully in other studies of this type (Ranno et al., 2007; Zhang et al., 2012; Xu et al., 2014; Guedes et al., 2016):
Where Pads is the sorbed quantity in the solid phase (mg kg-1), C is the concentration in solution (mg L-1) in equilibrium in the aqueous phase, Pmax is the maximum sorption or capacity (mg kg-1), and K is a constant related to the energy of P retention (L mg-1).
Effect of contact time
The effect of time on the availability of added P, equivalent to a field dose of 32 kg ha-1 P2O5, was tested. The soils (20 g) were incubated aerobically in 100 mL polyethylene plastic containers at 19±2°C and 75% of field capacity. The soil/ biochar/P contact times were 0, 7, 14, 21, and 30 d for all treatments. A 2.5 g sample was taken for each incubation time and treatment. Available P was determined using the Bray II method (Gómez and Rojas, 2006). All the values were corrected subtracting edaphic P extracted from control soil whit no P exogenous addition. The test was conducted three times.
Sample analysis
P concentrations in solution (1 mL) were determined spectrophotometrically, using the blue molybdenum method with 2% reproducibility (Murphy and Riley, 1962). The adsorbed/desorbed P was calculated as the difference between the quantity of initial P added and the remaining quantity in solution.
Results and discussion
Phosphorus sorption and desorption
The data obtained for the sorption isotherms were sufficiently fitted to the Langmuir equation, with an r 2 in all cases of upper to 0.9925 (Tab. 2).
TABLE 2 Parameters of the Langmuir isotherm, describing sorption in different soil/biochar treatments.
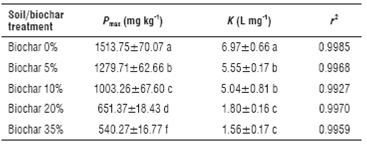
Figure 1 shows the data for the sorption and desorption isotherms (average of the three assays, with standard deviations below the size of the symbol). Sorption increases when the concentration of P in solution increases for all the treatments. P sorption in this soil and soil/ biochar mixtures is characterized by L-type isotherms, showing a progressive saturation of the solid phase when P in solute concentration increases, this behavior is more evident when biochar is added to soil. The comparison of isotherms graphics suggests that the influence of biochar is greater at higher P levels and high percentages of added biochar. In the lowest P concentration, sorption did not vary appreciably with biochar addition. However, when the P concentration increases, sorption is more sensitive to the addition of biochar, especially at the 35% proportion.
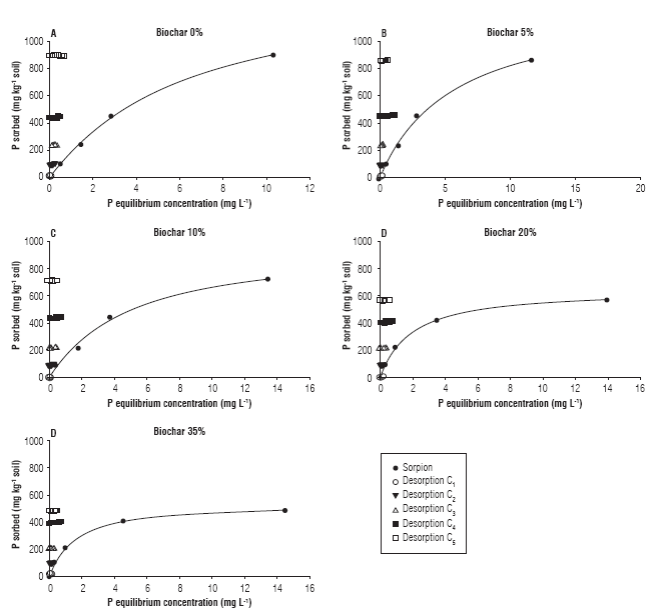
FIGURE 1 P sorption isotherms and phosphorus desorption points for each concentration of soil/biochar treatments.
The Langmuir isotherm parameters (Tab. 2) indicate that this soil presents high phosphorus fixation capacity and shown that P sorption decreases when the proportion of biochar increases, as Pmax varies from 1,513.75 to 540.27 for biochar percentages from 0 to 35%. This behavior is analogous to that found in other types of biochar applied to acidic soils (Nelson et al., 2011; Xu et al., 2014; Soinne et al., 2014). A decrease in soil acidity is frequently reported after biochar applications (Xu et al., 2014; Gul et al., 2015). A lower adsorbed quantity could be attributed to an increase in soil pH values with the addition of biochar (pH 7, Tab. 1). The pH determinations for different samples showed an increase from 5.4 in the soil up to 6.3 in the treatment with 35% biochar. The increase in pH value for acidic soils translates into an increase in the number of negative charges of soil colloids, leading to an increase in anionic repulsion and reduction of sorption of phosphate anions (Murphy and Stevens, 2010; Mukherjee et al., 2011). K values showed a decrease as the percentage of biochar increased in the treatment, indicating that sorption is lower and interactions between quantities of adsorbed P and the biochar are weaker. The suggested mechanism for interactions between phosphate anions and the surface of the biochar is the formation of hydrogen bridges with functional elements of the biochar surface: hydroxyl groups, carboxyl groups, carbonyl groups, and even phenolics (Chun et al., 2004; Chen et al., 2008); however, the capacity of biochar to retain phosphates is much lower than that of the soil because the biochar was obtained at high temperatures, high heating velocities, and long contact periods. Under these conditions, the volatile matter and surface functional groups decreased, with an increase in less-acidic oxygenated groups (Jiménez et al., 2006; Lehmann, 2007).
Figure 1 also shows along with P-sorption isotherms, the desorption points for each concentration and percentage of biochar. In the desorption process, one may infer that desorbed concentrations are low in all cases, including for greater concentrations. The generally low phosphate desorption rates indicated the strong interaction between soil and P (Ren et al., 2015). To better observe the effect of the presence of biochar, the accumulated desorbed P concentration was calculated (eight extractions) expressed as mg kg-1 of soil (Fig. 2). The results demonstrate that the presence of biochar favors P desorption, leading to a greater quantity of free P in the soil solution, prior studies shown the same tendency (De Luca et al., 2009). The accumulated quantity of desorbed edaphic P increases from 46.90±1.92 to 79.71±2.32 mg kg-1 when adding 35% biochar, indicating that plant char favors the bioavailability of P to 85%, even when P has not been added in exogenous form to the soil. In this case, the increase could be due to the liberation of P by the biochar itself, as it contains available P (Tab. 1) at an increased pH value. This behavior has been described by other researchers (Lehmann et al., 2003; Steiner et al., 2007; De Luca et al., 2009). Except at the highest concentration, no statistically significant differences between the concentrations were found by adding low proportions of biochar to soil (5 and 10%). For greater concentrations of added P, the values are in the range of 73.36±2.23 to 113.75±3.40 mg kg-1 from 0 to 35% biochar in the treatment, supposing an increase close to 78%. The results indicate that the presence of biochar favors a slow and gradual availability of P in soil solution. These results are also consistent with sorption isotherms and with what has been found with other soils amended with biochar (De Luca et al., 2009; Mukherjee and Zimmerman, 2013).
Effect of contact time
Normally, it is recommended to fertilize with P when the nutrient concentrations in solution are below 15 mg kg-1, and production output increases considerably when phosphorated fertilizers are added to soils containing 10 mg kg-1. In the incubation test (Fig. 3) for soil that was not amended with biochar, the results demonstrate that only 8.14±0.51 mg kg-1 of the P is in useable form by plants, indicating that 18.6% of the P added as a fertilizer has reacted with the free aluminum and iron in the soil (Tab. 1), as is typical in this type of soil (Quesada et al., 2011). The liberation of P during the test period is low, with a slight increase over 30 d (from 8.14±0.51 at the beginning of incubation until 8.92±0.57 mg kg-1). To the extent that the percentage of added biochar increases, the concentration of free P is greater at both the outset and at the end of the contact period. The greatest effects on availability are for the 20 and 35% biochar treatments, in which the P concentrations in solution after incubation increased from 10.90±0.66 to 13.70±0.80 mg kg-1 (25%) and from 12.60±0.79 to 17.4±0.95 mg kg-1 (38%), respectively. The treatments free greater concentrations of P slowly and progressively, maintaining sufficient P in solution for plants to use. This process could take place under natural conditions and lead to better crop performance in fields where biochar is applied along with fertilizers (Steiner et al., 2007). Still, great care should be taken in extrapolating lab results to the field because, under natural conditions, a greater concentration of P in soil solution can also lead to greater losses through leaching, depending on the precipitation that takes place.
Conclusions
This study was able to confirm that the application of biochar from Eucalyptus globullus obtained at high temperatures, high heating velocity, and long contact periods improves the availability of P in acidic soils. The interac-tions between P and biochar are weaker than with the soil itself and the sorption capacity is nearly one hundred times less when biochar at 35% is added. This phenomenon could be explained by the increase in negative charges in soil with biochar, which repels phosphated species and liberates them into the soil solution.
Using desorption tests, it was demonstrated that the presence of biochar increases the liberation of phosphorus compared to natural soil, in percentages between 78 and 84% after eight consecutive desorptions. Thus, we would expect that under field conditions, an acidic soil with bio-char amendment would more easily liberate this nutrient when post-fertilization precipitation takes place.
In light of the incubation results, the presence of biochar favors the slow liberation of phosphorated fertilizers into the soil solution, thus maintaining the necessary quantity over time for correct plant nutrition.
The results indicate that biochar, especially at 20 and 35%, leads to changes in soil properties that might be of benefit for agricultural soils, accordingly we conclude that Eucalyptus globullus biochar is promising to potentially improve soil P availability in acidic soils. Still, tests under field conditions are recommended, where other natural phenomena such as leaching might affect the final concentration of P in soil solution.