Introduction
The tomato (Solanum lycopersicum) has the highest crop distribution in the world (Ruiz Celma et al., 2009). Tomato is one of the staple foods in Colombia, with an annual per capita consumption of 9.4 kg (Ulrichs et al., 2008). This horticultural commodity is a basic component of the Colombian diet and is used almost on a daily basis as part of raw or home cooked preparations. Despite high production, the high percentage of postharvest losses in tomato makes necessary to find a preservation method to maintain characteristics and extend shelf-life (Barringer, 2008). Dehydration is mainly used in food preservation to create a product stable over time and to reduce product volume (Marjanovi et al., 2015; Das Purkayastha et al., 2013).
Color and texture are two decisive factors in the sensory quality of dehydrated foods. In addition, the drying can change the superficial characteristics of food, altering the color (Fellows, 2009). Some studies have shown that dehydration with heated air causes the loss of red coloring at temperatures above 40°C (Kerkhofs et al., 2005) or 60°C (Das Purkayastha et al., 2013). In addition, the texture is affected by the loss of differential permeability in the protoplasmatic membrane, the loss of turgency pressure in the cells and the denaturalization of proteins during the process (Jangam et al., 2010).
The regular consumption of tomatoes has been linked to a reduction in the risk of developing certain types of cancers and cardiovascular problems, and to a wide range of other potential benefits (Azeez et al., 2017; Dumas et al., 2003; Gümüs, 2015). Positive effects are attributed to the antioxidants, especially vitamin C, and phenolic components and carotenoids such as lycopene and β-carotene present in the tomato (Pinela et al., 2009).
One of the more abundant components in the tomato is lycopene, which is responsible for the red color (Shi and Xue, 2009) and is considered an antioxidant with high biological activity, representing almost 80% of the carotenoid content in the tomato (Arslan and Özcan, 2011; Das Purkayastha et al., 2013). Some studies have indicated that the increase in temperature during the drying process degrades these components; however, various studies have shown a significantly increased carotenoid content in dehydrated tomatoes as compared to fresh ones. Additionally, the tomato contains phenolic components that can withstand some industrial processes such as drying (Fuentes et al., 2013). There are several studies that have observed an increasing trend for this type of compound (Chang, et al., 2006; Mechlouch et al., 2012). However, the results of recent studies on dried fruit material have shown a clear decrease in polyphenols (Kerkhofs et al., 2005). In general, the majority of authors have demonstrated an increase in antioxidant activity contents during processing (Abano et al., 2011; Motamedzadegan and Tabarestani, 2010), which some studies have specifically reported for the drying process (Mechlouch et al., 2012).
The present study aimed to evaluate the changes in the physicochemical, antioxidant, and microstructural properties of the tomato variety Chonto during a drying process at three different temperatures.
Materials and methods
The tomato (Solanum lycopersicum) variety Chonto was used, with a maturity stage of 6 (100% superficial red color) (Heuvelink, 2005), from the municipality of Sachica in the department of Boyaca (Colombia), with diameters of approximately 6 cm. In preparing the fruits, they were cut into slices that were approximately 3 mm thick. Afterwards, an experimental drying chamber was used to carry out the drying at three different temperatures (50, 60 and 70°C) and an air velocity of 4 m s-1, three replications were carried out for each evaluated temperature.
Color
The color was measured using a Minolta colorimeter, Model C-300 (Konica Minolta, Shanghai, China), with the CIE system and the coordinates L*, a*, b* for the description of the color. Three replications were carried out for each temperature analyzed to take the data on the pulp of tomato. Afterwards, the hue (h ab ) and chromaticity (C* ab ) were calculated using equations 1 and 2 (Albanese et al., 2014).
Texture
A SMS texturometer, Model TA-XT plus (Stable Micro Systems Ltd., Godalming, UK) was used to measure texture. A texture profile analysis (TPA) was used to characterize the texture of the samples, with a 75 mm compression disc and an insertion distance of 5 mm. Five replications were carried out for each evaluated temperature. For the texture properties (hardness, fracturability, elasticity, cohesiveness and masticability), a principal components analysis PCA was carried out to analyze the various texture variables simultaneously, this a technique that seeks to reduce the dimensionality of the data, maintaining by this technique the greatest amount of data information, which is determined by the variance obtained.
Total carotenoid content
A 1 g tomato sample was added to 3 mL of acetone and agitated at 2,000 rpm for one minute, and then centrifuged at 9,000 rpm at 7°C for 5 min. The supernatant was separated and set aside; the residue was again submitted to various extractions, adding 3 mL of acetone and removing the supernatant until the residue had no coloration. Afterwards, 3 mL of petroleum ether were added to all of the supernatant and agitated at 1,000 rpm for one minute; the upper phase was separated, which corresponded to the petroleum ether, and set aside. This liquid-liquid extraction was repeated until the petroleum ether did not extract more color. Finally, the ether extract was dried with anhydrous sodium sulfate and filtered, and then brought to 25 mL with petroleum ether.
The extract was measured for absorbance at a wavelength of 450 nm, UV-visible spectrophotometer, and was quantified using a calibration curve of β-carotene with concentrations between 0.54 and 4.75 µg of β-carotene/mL of extract (Abano et al., 2011). Five replications were carried out for each evaluated temperature.
Total phenol content
A 1 g tomato sample was submitted to extraction with 10 mL of acetone, agitated at 1,000 rpm for 3 min and stored at 4°C in darkness for 4 h; then, agitated at 9,000 rpm for 5 min, filtered and brought to 25 mL in a cylinder.
To determine the total phenol content, 1.2 mL of distilled water, 20 µL of extract, 100 µL of Folin-Ciocalteu reagent and 300 µL of calcium carbonate were mixed at 20% w/v in a short pathlength cell; and then stored in darkness for 2 hours and absorbance was measured at a wavelength of 765 nm. The total phenol content was quantified using a calibration curve of caffeic acid with concentrations between 23.9 and 299.0 µg of caffeic acid/mL of extract (Sahlin et al., 2004). Five replications were carried out for each evaluated temperature
Antioxidant activity
The ABTS method of radical cation discoloration was used to determine antioxidant activity. To generate the ABTS radical cation, 38.41 mg of the ABTS diammonium salt and 6.63 mg of the potassium persulfate were taken and dissolved in 10 mL of deionized water; the mixture was stored at room temperature in darkness for 16 hours. The solution used for the analysis was prepared by dissolving the above solution in ethanol until an absorbance between 0.700 and 0.800 at 734 nm was reached. Then, 1 mL of solution and 10 µL of extract were added to a short pathlength cell and left to incubate for 6 minutes. The absorbance was measured at a wavelength of 734 nm before and after incubation to record the difference, quantifying the values with a calibration curve of Trolox with concentrations between 50.5 and 406.0 µmol L-1 of Trolox of extract (Sahlin et al., 2004). Five replications were carried out for each evaluated temperature
Electron microscope scan
The metallization was performed in a Balzers brand sputter, SDC-050, (<10-1 torr) with argon as the etching gas (plasma) on a gold-palladium plate (anode) (8:2). The film was deposited on the samples (cathode) at a discharge current of de +/- 50 mA and a typical thickness of +/- 200 nm.
A FEI QUANTA 200 scanning electron microscope (Thermo Fisher Scientific, Hillsboro, OR, USA) was used, taking micrographs with the XT microscope control program, version 2.01, recording observations of the pulp and seed at 500 x and producing two micrographs for each of the process temperatures.
Statistical analysis
Statistical analysis of the data was carried out with analysis of variance (Anova. Additionally, Tukey’s test was used to observe whether or not there were significant differences between the drying temperatures the software for made this analysis was R®. Principal component analysis (PCA) was employed on the texture properties to observe the simultaneous effects of the variables, the software for made this analysis was Matlab®.
Results and discussion
Color
The L* parameter indicates the luminosity of the sample; and coordinates a* and b* help to show the hue (h ab ) and the chromaticity (C*ab) (Askari et al., 2009). The luminosity of the samples was not affected by the change in temperature (Tab. 1) and the mean values indicated that there was no connection between this parameter and the process temperature. The hue values decrease as temperature increased; and, at 70°C, a value that was significantly lower with respect to the fresh value and the other temperatures of the process was seen. At 50 and 60°C, the values were similar to those of fresh tomatoes for the hue angle; however, at 70°C, a hue angle of 1.72° was seen (Tab. 1), a strong red hue, contrary to the theory that the hue angle increases at high temperatures (Al-Muhtaseb et al., 2010).
TABLE 1 Influence of the temperature on the color coordinates. The values are reported as the mean ± standard deviation of the samples; the letter indicates the clusters obtained in the Tukey’s test to discover significant differences.
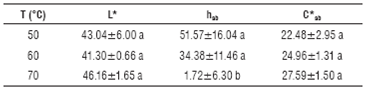
The probability of browning in the samples was lower at higher hue values. Hawlader et al. (2006) indicated that a decrease in the values of hue angle is an indication of the formation of components that confer a brown pigment, moving away from yellow coloring. The chromaticity indicated that high saturation can assume the color of the sample; in this case, there was no significant difference between the samples (P>0.05) (Tab. 1), although a study created a chromaticity scale specific for the tomato which indicates that if this parameter has values over 20, the coloration of the fresh tomato is excellent (Saad et al., 2016). All of the samples surpassed this value, indicating commercial acceptance in terms of chromaticity. The most appropriate temperatures for the process are 50 and 60°C because their colorations are closer to fresh tomatoes. The caramelization of sugars and the Maillard reaction were produced more rapidly with an increase in temperature, hence, prolonged process times and overly high temperatures promote this reaction, forming brown pigments that are detrimental to product quality, which in the case of the tomato, is believed to occur at temperatures over 60°C (Das Purkayastha et al., 2013), as confirmed by the results of the present study.
Texture
The principal components analysis was carried out discriminating the samples from the different process temperatures (50, 60 and 70°C). As can be seen in figure 1 (Score plot), which shows the influence of the samples on variance, and figure 2 (Loading plot), which shows the influence of the variables on the variance, the graphs of the principal components analysis shows how the first two principal components together explain 75.37% of the variance of the experimental data, which are a group of lineal combinations found to describe the variance of the samples. The results show significant separation between the samples at the three different temperatures, indicating significant changes in the texture for each temperature of the drying process. Studies indicate that hardness is seriously affected by drying with heated air due to the effects of thermal treatments on the superficial structure (Askari et al., 2009). Figure 2 shows the distribution of the studied texture variables (hardness, fracturability, elasticity, cohesiveness and masticability), which indicates that elasticity and cohesiveness are highly related and have an inverse relationship with fracturability. When comparing the two figures, one can conclude that, at 50°C, they are associated characteristics, equal to the samples dehydrated at 70°C. The samples dried at 70°C presented a higher masticability and hardness since overlaying figure 1 and figure 2 shows that, at this temperature, the samples have better correlation with these variables. Samples dehydrated at 60°C also showed this behavior, unlike the other two, with higher correlation with fracturability. Additionally, intermediate hardness and masticability were seen in the 50 and 70°C samples. This can be explained by the fact that a product with more water loss is going to acquire a fragile structure and fracturability, but at a certain point, the structure starts to form a crust around the sample, thereby impeding water loss. And so, the structure becomes billable and rubbery, so that the tomato is in transition between 60 and 70°C. Gobbi (2009) indicated that, for dehydrated products, crispness is a highly acceptable property by consumers and that fracturability is proportional to crispness in the product.
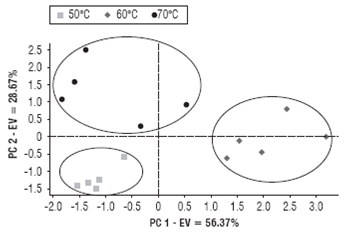
FIGURE 1 Principal components analysis for tomato samples dried at different temperatures (score plot).
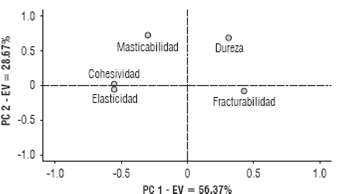
FIGURE 2 Principal components analysis PCA for tomato samples dried at different temperatures (loading plot).
Generally, the drying process drastically alters the texture of the product without concern for how the transformation is carried out (Askari et al., 2009) because one cannot expect a dehydrated product to having a texture that is similar to the fresh product. However, it is necessary to find a texture that is agreeable to consumers and that, along with the other quality characteristics, contributes to a commercial acceptance.
Total carotenoids
Table 3 shows the effect of the temperature in the different antioxidants characteristics of tomato. The fresh tomatoes presented a mean value of 30.07 mg of β-carotene/100 g of dry material. The results of some studies show β-carotene contents in the same range with values between 50 and 60 mg of β-carotene/100 mg of tomato (Zapata et al., 2007); other studies have established much lower carotenoid values, between 0.5 and 1 µg of β-carotene/100 g of tomato and 0.4 and 0.8 mg β-carotene/100 g of tomato for the Rio Grande de México variety (Mechlouch et al., 2012). These differences are attributed to carotenoid content, which varies depending on the origin, conditions of the cultivation and the variety. Previous tomato studies have also reported values similar to those found in the Chonto variety, presenting ranges between 35 and 45 mg β-carotene/100 g of dry tomato material (Dos Santos et al., 2012; Kerkhofs et al., 2005)
TABLE 3: Effect of different dehydration temperatures on the antioxidant component content in tomatoes. The values are reported as the mean ± standard deviation of the samples; the boldface letter indicates the clusters obtained in the Tukey’s test to discover significant differences.
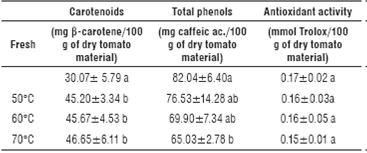
Additionally, the carotenoid content was favored by the different thermal processes, such as dehydration, concentration, etc.; with some exceptions (e.g. boiling, baking, and frying), which decreased carotenoids with respect to fresh tomatoes (Sahlin et al., 2004). However, studies on dehydrated tomatoes have found a significant increase in carotenoids with respect to fresh content (Arslan and Özcan, 2011; Das Purkayastha et al., 2013; Giovanelli et al., 2002; Takeoka et al., 2001). Correlating the obtained data with some studies produced values between 45 and 60 mg/100g of dry tomato material (Kerkhofs et al., 2005), carotenoid behavior during drying is similar to the ‘Chonto’ tomato used in this study. The thermal process significantly increased the content of bioavailable carotenoids. This increase was principally due to the increased freeing of phytochemicals of the food matrix, which made easier to extract these components. Thermal processing of the tomato can improve the bioavailability of lycopene, the principal carotene in the fruit, breaking the cells of the walls (Shi and Xue, 2009).
Total phenols
Although there is a tendency to decrease phenolic components, significant changes were not seen until 70° (P>0.05). The reviewed studies based on fresh material showed a difference in polyphenols, however, these data are not comparable due to the fact that the water content of fresh tomatoes and dehydrated tomatoes is different. These studies showed that the phenols have a tendency to increase (Chang et al., 2006; Mechlouch et al., 2012). However, when observing studies with results based on dry material, a downward trend in polyphenols during the drying can be observed (Kerkhofs et al., 2005). The thermal treatments freed phenolic components, provoking the destruction of some cellular components; freeing oxidative enzymes that can destroy these antioxidant components in fruits and vegetables (Gümüs, 2015).
Antioxidant activity
The results show a decreasing tend, but there was not a significant change in antioxidant activity for any temperature (P>0.05) (Tab. 3), confirming that drying generally does not affect the antioxidant activity in the tomato. The majority of the studies showed an antioxidant activity below than reported (Kotíková et al., 2011; Toor and Savage, 2005; Zanfini et al., 2010), inferring that the Chonto tomato variety has a high antioxidant activity. However, some articles have reported an antioxidant activity higher than ‘Chonto’ and with a tendency to increase with dehydration (Mechlouch et al., 2012). This could be due to the presence of other antioxidant components which the Chonto variety has in lower proportions (Kerkhofs et al., 2005). The stability of the antioxidant activity of tomatoes is mainly due to the synergistic effect that the two principal groups of bioactive components have, i.e. the carotenes and phenols, because when there is a positive effect on the carotene content and a negative effect on the phenols, the antioxidant activity is balanced (Shi and Xue, 2009), conserving, in this case, the antioxidant activity without any change.
Microestructural analysis
Figure 3 shows the microphotographs of the dehydrated tomato pulp at different temperatures. At 50°C, a structure corresponding to the mesocarp of the tomato was observed with smoother curves, indicating a lack of structural damage. However, at 60 and 70°C, stronger bends were seen, indicating a hardening of the endocarp and mesocarp, producing more fracturability at those temperatures. The texture tests indicated that the temperature that most effected fracturability was 60°C and this was confirmed in the microphotographs because at this temperature the bends in the mesocarp were higher in number and were more pronounced. This was mainly due to the protoplasmic membrane being affected by the loss of differential permeability, the loss of turgency pressure in the cells and the denaturalization of proteins during the drying (Askari et al., 2009).
Figure 4 shows observations, at different drying temperatures, of the seeds, with the same development as the pulp, but in this case the bends are more pronounced at 70°C, to the point that one can observe small particles at 70°C, which could be detachments of the seed coat given the fact that high temperatures break tissues of the episperm. This results because the principal function within the seed is the absorption of water and the protection of the internal part and the evaporation of water through heated air seriously affect the seed coat, which becomes porous and exposes the internal structure of the seed (Heuvelink, 2005).
Conclusions
The drying process prompts changes in the quality characteristics of fruit. It is recommended that tomato samples have to be dried at a temperature no higher than 60°C in order to avoid browning reactions and the degradation of pigments which affect the color of the fruit and cause browning. The texture characteristics take on a more important role due to the fact that, at high temperatures, the product acquires fracturability, which is an important factor in the acceptance of dehydrated products, as seen in the microphotographs that demonstrate the huge difference in the structures with increasing temperatures. The total carotenoid content was favored by the increase in temperature, thereby conserving the antioxidant capacity of the tomatoes.