Introduction
Radish (Raphanus sativus L.) originates from the Mediterranean region and belongs to the Brassicaceae family, being one of the oldest cultivated vegetables. The globular root is edible; it contains medicinal properties as stimulant of the digestive system and natural expectorant. It also contains vitamins A, C, B1, B2, B6, potassium, folic acid and calcium, has antioxidant activity, low amounts of calories and a high amount of dietary fiber (Camargo et al., 2007). The radish is a short cycle crop enabling space to other vegetables. Likewise, it can be grown in pots where the space of cultivation is limited. The cultivars of greater acceptance produce roots of bright scarlet coloration and white pulp. Currently, the culture has gained greater prominence among small and medium horticulturists due to the crop size rusticity and its short cycle, been harvested 25 to 35 d after sowing (Filgueira, 2008).
Nitrogen is the second most required nutrient by vegetables. This element is mainly absorbed in form of nitrate or ammonium, and constitutes amino acids, proteins and enzymes. In this way, it exerts influence on the growth of plant species and on the production of reserve substances and maturation. Ammoniacal nitrogen can bring some benefits to plants, because it acts as an important intermediary in many metabolic reactions (Britto and Kronzucker, 2002), has lower energy expenditure for its metabolism, and dispenses the reduction phases, which are required to the absorption of NO3 - (Hachiya et al., 2012). However, elevated concentrations of NH4 + lead to several issues: induces toxicity in plants, may lead to chlorosis in leaves, reduction of photosynthesis, lower plant performance, lower nutrient level, cation and metabolites mismatches, as well as a decrease of amino acids and organic acids (Britto and Kronzucker, 2002).
To reduce ammonium toxicity in plants, the use of beneficial elements such as silicon (Si) is recommended. Its effect is related to the mitigation of stresses (Liang et al., 2007; Bybordi, 2010) by increasing the activity of antioxidant enzymes (Gao et al., 2014), the physical integrity of the membranes (Kochanová et al., 2014) and the photosynthetic activity (Vaculíková et al., 2014), resulting in increased plant growth. In canola plants (Brassica napus L.) under salt conditions, Bybordi (2010) observed a beneficial relationship of Si and ammonium, where the ammonium increased the production of fresh matter and foliar area in the presence of the beneficial element, evidencing the beneficial effect of the Si interaction with high ammonium concentrations.
In recent studies (Barreto et al., 2016) on the strengthening of silicon in the nutrient solution in tomato plants under excess of ammonium, researchers have found an increase in the accumulation and the efficiency of use of nitrogen in the leaf area and the dry biomass of roots. However, there are not studies on ammoniacal toxicity in radish crop and its relationship with silicon nutrition.
Therefore, hypothetically ammoniacal toxicity in radish cultivation affects the physiology of the plant and its damage is greater in the root than in the aerial part, where silicon would beneficts plans against ammonium toxicity.
The present study had the objective to evaluate the dry matter production, accumulation of nitrogen and silicon in the roots and the aerial part as well as the physiological variables in radish culture, as a function of ammonium concentrations in the nutrient solution in the absence and presence of silicon.
Material and methods
The study was conducted in a greenhouse at the Faculty of Agricultural and Veterinary Sciences, Sao Paulo State University (UNESP) in Jaboticabal, SP, Brazil, at the geographic coordinates of 21°15'22" S and 48°18'58'' W, with radish (Raphanus sativus var. Crimson Giant) cultivation being grown in pots from July to September, 2015. This variety is characterized by developing red and white colors, large 3-4 inch (7.5-10 cm), globe shaped crimson roots with white pulp. Plants were grown under controlled environmental conditions in a growth chamber with a light/dark regime of 13/11 h, temperature regime of 35/25°C, and relative humidity of about 50%. A completely randomized design was adopted, with four replicates, in a 5x2 factorial scheme, corresponding to the absence and presence of Si (2 mmol L-1) and five ammonium concentrations (1.0, 7.5, 15, 22.5, or 30 mmol L-1). For the ammonium composition, the nitrogen source was NH4Cl. Si was supplied as H4 SiO4 (Si = 28.5 g L-1). The experimental unit was composed of plastic pots with a capacity of 3.8 L. Seeds were sown in plastic pots placing eight seeds at each pot in a depth of 2 cm. After 5 d of emergency, thinning was made leaving four plants in each pot, allowing adequate distance for root growth. Substrate mixture of (1:1, v/v) medium vermiculite (90 to 100% of the particles between 1.19 and 1.50 mm) and superfine (90 to 100% of the particles between 0.21 and 0.30 mm) was used, allowing for adequate porosity and aeration (Sarasketa et al., 2014; Barreto et al., 2016).
Initially 100 mL of distilled water by pot during the first 7 d were used to guarantee the required moisture for the seedlings germination. Starting from this time 200 mL of solution nutritive per every pot proposed by Hoagland and Arnon (1950) were used, with a modification in the iron source mainly alternating of Fe-EDTA for Fe-EDDHMA.
The nitrogen source used to compose the treatments was ammonium chloride, which maintained the nutrient solution pH value between 5.5 and 6.0.
After 30 d of the germination, the vegetative cycle of this species was completed and the plants showed clear affectations by the toxicity of NH4+ which marked the beginning of the evaluations, starting with the green color index, measurements were performed in the second pair of fully developed true leaves, using a chlorophyllometer (Opti-Sciences®, CCM-200, and Chlorophyll Meter, Hudson, NH, USA). The remaining physiological variables evaluated were photosynthesis, stomatal conductance (mmol m-2 s-1) and transpiration (mmol H2O s-1 m-2). Measurements were practiced on the second pair of fully expanded leaves from the top to the bottom, between 08: 00 and 09: 00 am. An Infrared gas analyzer was used (LICOR, Inc., LI-6400, Lincoln, NE, USA). The leaf area (cm2), was measured in all leaves of the plants from a digital meter (Li-Cor, model L1-3000®, Lincoln, NE, USA).
All the plant material was ground in a Willey mill, where N levels were determined as ammoniacal form, following the methodology described by Bataglia et al. (1983), and the silicon levels, according to Kraska and Breitenbech (2010). Depending on the levels of this nutrient and the dry matter, the accumulation in the roots and aerial part (mg per plant) was calculated.
Statistical analysis
The data were subjected to analysis of variance by the F test, the means were compared by the Tukey test (P≤0.05). In all variables, a polynomial regression analysis was applied, depending on the ammonium concentrations, where SISVAR 3.01 software was used for (Ferreira, 2011).
Results and discussion
The increase of nitrogen concentration in the nutrient solution raised the accumulation of this element in the aerial part of the plants reaching the maximum value at a concentration of 22.7 mmol L-1 with 5.74 mg per plant (Fig. 1A). Otherwise, the highest accumulation in the root also was related with the studied factors, obtaining the maximum value to the concentration of 15 mmol L-1 which represents 1.60 mg per plant (Fig. 1B). The balance of N sources in the nutrient solution regarding the N accumulation is widely known in the literature (Zanin et al., 2015). This effect, probably occurs due to the increase of the ammonium concentration up to non-toxic levels in the cell's cytoplasm, not causing toxicity to the radish plants since they can adopt different strategies which include the regulation of the ammonium transporters and the increased uptake of this ion (Setien et al., 2014).
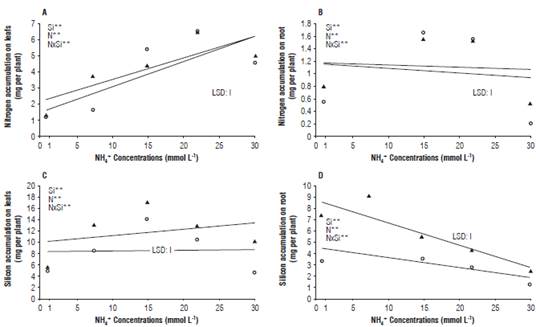
FIGURE 1 Effect of ammonium concentrations in the absence and presence of silicon on the accumulation of ammonium nitrogen and silicon in radish plants. Nitrogen accumulations in the shoots (A) and the root (B), silicon accumulation in the shoot (C) and the root (D). LSD, *, ** and ns = least significant difference, significant at P≤0.05, P≤0.01 for the F-test.
However, a higher proportion of NH4 + decreased the accumulation of total N in the plants, a fact found in this research and reported by other authors in crops considered sensitive, such as Arabidopsis thaliana (Sarasketa et al., 2014) and Cucumis sativus (Roosta et al., 2009). Moreover the excess of ammonium in the nutritive solution caused a high accumulation of this cation in the chloroplasts being able to block the metabolism of the enzymatic complex GS-GOGAT, responsible for the assimilation of nitrogen by plants (Bittsánszky et al., 2015). While in the presence of silicon, there was greater accumulation of this element in the plants followed by a progressive increase in the aerial part, until the concentration of ammonium reached 17 mmol L-1. Corresponding to its highest level and representing 16.10 mg per plants (Fig. 1C). This could be influenced by the higher absorption of silicon by the plants, increasing the absorption of silicon cations (Chen et al., 2011). A different behavior was observed in figure 1D, where silicon accumulation was lower with increasing ammonium concentrations in roots, being higher with the presence of this element in the nutrient solution and at the same time been influenced because the ammoniacal N, causes different damages to the root system, such as reducing growth, turning plant to brown colors, and necrosis (Bianco et al., 2015). These authors suggested that the excess of ammonium also produces greater growth of vegetative buds compared to reproductive buds.
Otherwise, the presence of silicon, in excess of ammonium, increased the index of green color of the leaves, decreasing in the treatments without silicon and in the smaller and greater doses. Reaching the maximum point with concentration of 29.53 mmol L-1 (Fig. 2A). This may be because of the higher uptake of Si by the plants under stress conditions, which can increase the physical integrity of the membranes on different abiotic stresses (Kochanová et al., 2014). These results can also be explained by the fact that Si promotes protective effects on photosynthetic mechanisms and nutrient balance (Mateos Naranjo et al., 2013). The photosynthesis rate decreased when ammonium concentration increased, especially without using Si in the nutrient solution (Fig. 2B). Therefore, the use of Si in the nutrient solution alleviated the decrease of photosynthesis due to ammonium toxicity. The increase of the ammonium concentration decreased the transpiration (Fig. 2C) and the stomatal conductance of the radish plants (Fig. 2D), especially with the incorporation of Si in the nutrient solution. The addition of Si could protect the photosynthetic pigments in leaves, alleviating the damage to chloroplast ultrastructure, and increasing the expression of genes associated with photosynthesis (Song et al., 2014). The silicon, after being absorbed as monossilicic acid, is accumulated in the abaxial region of the leaves, making them upright and with greater light absorption and increasing the enzymatic activities responsible for the reduction and assimilation of nitrogen (Feng et al., 2010). In addition, silicon can form a double silicate layer on the leaf epidermis controlling the stomata conductance, which could favor the photosynthetic efficiency of the plants (Kochanová et al., 2014).
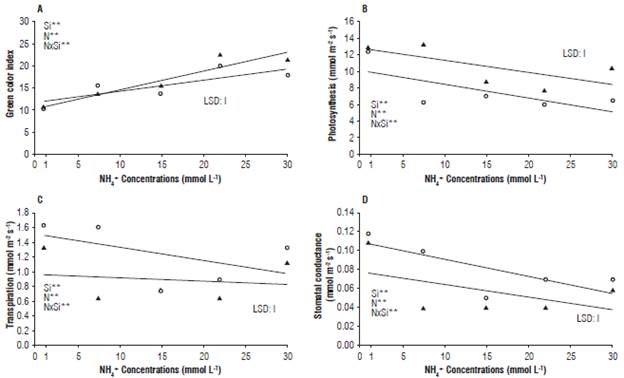
FIGURE 2 Effect of the concentrations of ammonium in the presence and absence of silicon, on four physiological variables in radish plants. Green color index (A), photosynthesis (B), Transpiration (C) and Stomatal conductance (D). LSD, *, ** and ns = least significant difference, significant at P≤0.05, P≤0.01 and non-significant level, respectively, for the F-test.
While for the foliar area, there was interaction between the factors, Si concentration and NH4 + concentrations followed a quadratic adjustment, where the maximum value of this variable was reached in the concentration of 12.1 mmol L-1 of ammonium in the presence of Si (Fig. 3A). However, the ammonium excess in the nutrient solution decreased the leaf area, independently of the presence or absence of Si, which might be influenced by the toxic effect and accumulation of that cation in the chloroplasts, blocking the metabolism of the GS-GOGAT enzymatic complex, responsible for the assimilation of N by plants (Bittsánszky et al., 2015).
The accumulation of ammonium in the aerial part was also influenced by the silicon, where in its absence the maximum point was reached at 16.3 mmol L-1, representing 1.12 g per plant, while he presence of this element in the nutrient solution led the maximum point at 16.5 mmol L-1 of ammonium, which means 1.5 g per plant of dry matter, respectively (Fig. 3B). With the absence and presence of silicon, it was found that its appropriate dose was achieved in the range of 10 and 9 mmol L-1 (90% of the maximum production) to 16.5 and 16.3 mmol L-1 (maximum production) and the toxic dose was achieved betwen (10% decrease in maximum shoot dry matter yield) 23.0 and 21.5 mmol L-1, respectively. Moreover, the critical level of ammonium toxicity was higher with the presence of Si in relation to its absence in the nutrient solution indicating a greater tolerance to the ammonium toxicity of the plants.
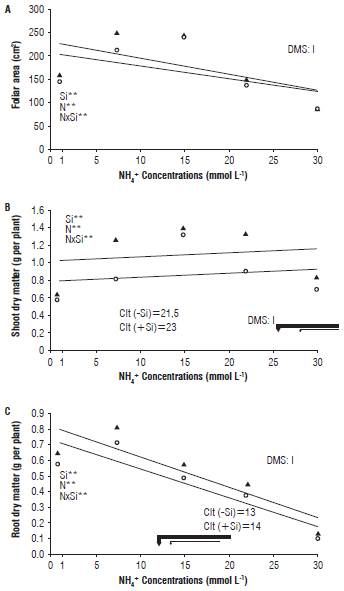
FIGURE 3 Effect of the concentrations of ammonium in the presence and absence of silicon in radish plants. Leaf area (A), shoot dry matter (B) and root (C). LSD, *, ** = least significant difference, significant at P≤0.05; P≤0.01 for the F-test.
Critical toxicity levels, corresponding to the presence or not of silicon in the nutrient solution, resulted in a decrease of shoot dry matter, inducing symptoms in older leaves, which initially showed chlorosis at the edges, which was progressively extended to the rest of the plant (being more notorious when the NH4 + concentration increased). After the critical or tolerance levels of the plant were reached, necrosis of a large part of the leaf area was observed.
In duckweed plants Lemna minor, the ammonium toxicity affected the aerial part , changing leaves of a green color to yellow, and also the roots becoming short (Huang et al., 2013). A recent study in the cultivation of Solanum lycopersicum verified a linear decrease of dry matter with the use of ammonium concentrations higher than 1 mmol L-1 in the nutrient solution (Barreto et al., 2016). In other research on different species, the effect of NH4 + toxicity can also be verified, and its critical concentration of toxicity in plants such as Lemna minor, with 3.3 mmol L-1 of NH4 + (Huang et al., 2013), and Solanum tuberosum, with 4.1 mmol L-1 of NH4 + (Zebarth et al., 2012). Root dry matter presented interaction between factors, influenced by the presence of silicon in the nutrient solution, reaching its maximum point in the concentration of 6.18 mmol L-1 representing 0.72 g per plant (Fig. 3C).
The appropriate NH4 + levels were determined following the same criteria as shoots dry matter. Based on this criterion we determined, for this crop, the adequate dose according to this variable and when Si presence is in the range of 1.00 mmol L-1 to 6.18 mmol L-1 and the critical level of toxicity was 14 mmol L-1, representing a reduction of more than 10% of the dry matter of the roots. However, in the absence of silicon, the maximum point was reached at the concentration of 5.28 mmol L-1 representing 0.64 g per plant when the critical level of toxicity was 13 mmol L-1, with a lower tolerance of the plants to the toxicity of ammonium related to the treatment with silicon presence in the nutritive solution. The ammonium toxicity is detrimental to the plants, as this cation is directly related to root development, which is mostly affected by the exposure of this ion in relation to the other plant organs with severe physiological and morphological changes (Zanin et al., 2015).
Conclusions
Ammonia toxicity in radish cultivation decreased photosynthesis, transpiration and stomatal conductance, with a greater decrease in the dry matter accumulation of the root when related to the aerial part this effect was reduced with the incorporation of silicon in the nutritious solution in the doses used for this research.