Introduction
In Colombia, potato is the fourth most important crop; in colder climates, it is the main agricultural production system with a planted area of 125,660 ha (ICA, 2011; FEDE-PAPA, 2017). Worldwide, the development of genetically modified (GM) plants using proteins for insect resistance has occurred in several prominent crops, including potatoes (ISAAA, 2017). Their use has demonstrated advantages such as the reduced use of pesticides and protection of the crop throughout the farming season, while averting damages to the associated entomofauna and the natural environment. This, in turn, has improved production and the economies of farmers that have adopted this technology (Sauka et al., 2008; Klümper and Qaim, 2014).
The Guatemalan potato moth, Tecia solanivora (Lepidóptera: Gelechiidae), is one of the main agricultural pests in potato farming in Colombia (ICA, 2015). Due to the fact that it affects the tuber's quality, it can cause direct losses of up to 35% (Vargas et al., 2004). When this insect undergoes complete metamorphosis, the larval stage inflicts the most damage. After the larvae emerge and following the female's oviposition in the rifts of the plant stems or under the soil adhering to the potato tubers (Vargas et al., 2004; López-Pazos and Cerón, 2013), microorganisms enter the plant through the vertical and horizontal galleries created by the larvae as they feed. Food residues, excrement, and mold left by the larvae rot and become compacted, causing the tubers to acquire a dark tonality in the field or during storage. This decreases the quality and commercial value of the tubers, causing in some cases their rejection (Niño, 2004).
For over 100 years the entomopathogenic bacterium Bacillus thuringiensis (Bt) has been used for the biological control of insect pests. This microorganism produces various virulent factors that enable it to colonize its host. The most widely studied proteins from this bacterium are Cry proteins. These proteins are active against pest insects of the orders Lepidoptera, Coleoptera and Diptera, and others (Soberón and Bravo, 2007). Cry proteins have high specificity because their action mechanism depends on various stages, which include their solubilization and proteolytic activation in the insect. In addition, these proteins must be recognized by the protein receptors of the susceptible insect's intestinal membrane. Then, active oligomers must form to develop lithic pores that destroy the intestinal tissue's integrity, eventually causing the death of the host (Soberón and Bravo, 2007).
Important developments have been made on the biological activity of cry1Ba on T. solanivora as well as on the lethality of other Cry proteins. These developments make them a worthy alternative for consideration in biological plans for the control of this insect.
In order to obtain potato plants with resistance to the Guatemalan potato moth through the use of the cry1Ba1 gene, the gene must be included in an expression cassette. Therefore, in this research we designed five expression cassettes in silico, delving into certain elements inherent to the context of cassette design. The designed expression cassettes are preliminary for the development of future transgenic lines of the Pastusa Suprema potato. Using the tissue-specific promoter patatin B33 diminishes potential biosafety risks, since the plants would only express the transgene in the tuber. The tuber is the tissue affected by T. solanivora, avoiding exposure of the entomofauna associated with the crop or gene flow (Vanegas et al., 2010).
Research on GM crops for insect resistance has promoted the development for the expression of different cry genes. Having several options is valuable considering 1) biological scenarios that imply adaptation to diverse agronomic environments; 2) biosafety contexts for commercial release; 3) alternatives for possible cases of resistance development to a specific Cry protein of a GM crop in the field; and 4) marketing contexts such as those that include intellectual property rights, besides other developments, suggesting the need for viable alternatives for generating new events with other cry1 genes. The development of transgenic lines with gene stacking using a cry gene that is different from cry1Ac is an alternative in scenarios such as the appearance of resistant pest insects (Pitre et al., 2008; López-Pazos et al., 2010).
Although genetic engineering programs have been initiated in Colombia to control T. solanivora using the cry1Ac gene, our research demonstrates the possibility of obtaining transgenic potato plants evaluated with other genes; the cry1B gene is a good candidate for developing plants that are resistant to T. solanivora.
Materials and methods
Background studies
We conducted two studies using recombinant Cry proteins on T. solanivora larvae. In the first study, higher toxicity results were observed for Cry1Ac protoxin compared to the other proteins evaluated (20% mortality at a concentration of 4.37 μg cm-2) by using protoxins Cry1Aa, Cry1Ab, Cry1Ac, Cry1B, Cry1C, Cry1D, and Cry1E expressed by recombinant strains of Escherichia coli. These protoxins were purified and assessed on the first instar of T. solanivora larvae at a concentration of 4 μg cm-2 (corresponding to the LC50 of the positive control strain Bt kurstaki HD1). Cry-1Aa, Cry1Ab, Cry1B, Cry1C protoxins showed mortality percentages of 6.24%, 6.76%, 4.16%, and 5.72% respectively, whereas protoxins Cry1E and Cry1D were the least toxic with an average mortality of 3.12% (Martínez et al., 2003).
In the second study, the recombinant toxins Cry1Aa, Cry1Ac, Cry1B, and Cry1C were activated with trypsin and purified by ion exchange column chromatography before their biological activity was assessed on the first instar of the T. solanivora larvae. This experience yielded an LC50 of 0.103 μg cm-2 for Cry1Aa, 0.107 μg cm-2 for Cry1Ac, 0.085 μg cm-2 for Cry1B, and 0.112 μg cm-2 for Cry1C.
These data were subjected to an analysis of variance that found no significant differences between the recombinant proteins. The similar average mortalities demonstrated that T. solanivora was highly susceptible to Cry1 proteins. The proteins used in this work had a high level of purity (chromatography) and conferred greater lethality because the proteolysis of the protoxin had been carried out in vitro, favoring their recognition by the receptor. The use of the activated toxins produced a faster effect on the larvae. The Cry1B protein showed the best results in a subsequent trial evaluating a Cry1B-Cry1I hybrid protein in domain II (Pitre et al., 2008). This protein was obtained from a plasmid in E. coli, which encoded the hybrid protein that was constructed by replacing a section of domain II of the cry1B gene with the corresponding fragment of the cry1I gene. The protoxins produced were treated with trypsin and purified. The Cry1Ba-activated toxin evaluated individually has high activity towards T. solanivora with 60% mortality at a dose of 0.5 μg cm-2 (López-Pazos et al., 2010).
Valderrama et al. (2007) and Villanueva et al. (2014) examined the use of Cry proteins in potato crops by plant transgenesis. They found that the developed varieties have a high level of expression of the cry1Ac gene, and because they were expressed constitutively, they can pose biosafety issues with commercial release of the plant (Vanegas et al., 2010). Therefore, a study was carried out in which López and Chaparro-Giraldo (2007) propose an Agrobacterium tumefaciens transformation system of potato explants of the androsterile variety Pastusa Suprema. Androsterility is an important feature in the environmental biosafety of GM plants for their release in centers of origin or in crop areas of high biodiversity. In Colombia, Pastusa Suprema is the only potato variety with this characteristic obtained by conventional breeding processes. Torres et al. (2012) developed transgenic lines using the variety Pastusa Suprema which was transformed using the cry1Ac gene. These lines were transformed with the plasmid p1AcPRD, which contained the cry1Ac gene that was donated by the University of Ottawa under a material transfer agreement (MTA). These lines were subjected to a freedom to operate (FTO) analysis, in which it was concluded that the FTO was affected by the MTA, because it restricted its use to research only and did not allow commercialization (Hincapié and Chaparro-Giraldo, 2014).
Analysis of the biological activity of the Cry 1Ba1 protein
An exhaustive literature review of the available data evaluating the Cry1Ba protein on larvae of the Guatemalan potato moth led to a determination of the key parameters validating the selection of the cry1Ba1 gene as an option for potato transgenesis to confer resistance to T. solanivora, within the context of its toxic activity towards the insect.
In silico design of expression cassettes
We designed five expression cassettes in silico. We obtained the sequences from the nucleotide database of the National Center for Biotechnology Information (NCBI) as cry1Ba1 gene access number X06711.1, promoter B33 (NCBI A08215.1), promoter CaMV35S (NCBI AF234316.1) and T-nos (NCBI AET75772.1). We used the NCBI ORF Finder tool (http://www.ncbi.nlm.nih.gov/gorf/) to determine the cry1Ba1 gene open reading frame (ORF). We also used Visual Gene Developer 1.3 software (Jung and Mc-Dondald, 2011) to produce the codon use modification. We made modifications of codon usage to the cry1Ba1 gene sequences to ensure their closer resemblance to the potato gene sequences using the Kasuza database (http://www.kazusa.or.jp/codon/) considering splicing sites, polyad-enylation signals, and the removal of restriction sites that could interfere with transcription. Then we assembled the sequences with ApE-A plasmid Editor 2.0 software. We placed restriction enzyme recognition sequences present in the polylinker region of the pCAMBIA2300 vector that did not cut anywhere in the gene sequence at the ends of the construct. With this software, we verified that the inserted sites did not alter the reading frame. Generay Biotech Co. Ltd. (China) synthesized the cloning of the five expression cassettes designed in the pCAMBIA2300 vector.
Genetic transformation of Escherichia coli
The genetic transformation of the commercial strain DH5alpha of E. coli was carried out through electroporation, to clone each expression cassette. The bacterial strains were phenotypically selected by their growth in the presence of kanamycin, considering that the pCAMBIA2300 plasmid contains a resistance gene for this antibiotic; strains were confirmed using Polymerase Chain Reaction (PCR) assays. We performed plasmid DNA extraction using the GeneJET Plasmid Miniprep Kit (Termofisher, Waltham, MA, USA). The PCR reaction was carried out with 1X Buffer PCR, 0.5 uM dNTP MIX, 2.5 mM Mg, and 1 μM of the primers that amplified the expression cassette and specifically the cry1Ba1 gene, and 0.3 U Taq polymerase. The temperature cycle had the following stages: denaturation (95°C for 5 min), synthesis (95°C for 1 min, 57°C for 2 min, 72°C for 1 min, for 30 cycles), and elongation (72°C for 5 min).
Obtaining and characterizing Agrobacterium tumefaciens LBA4404
We transformed the strain LBA4404 of A. tumefaciens by electroporation with the plasmid pCAMBIA2300 containing each expression cassette. To this end, we incubated 25 μl of electrocompetent cells and 1 μl of vector (100 ng μl-1) in SOC medium (Triptein 2%, 8.56 mM NaCl, 2.5 mM KCl, 10 mM MgCl2 and 10 mM MgSO4 heptahydrate) for three hours at 28°C and planted in Luria Bertani growth (LB) medium with kanamycin (50 mg L-1) and rifampicin (50 mg L-1). These media were incubated at 28°C for 48 h. We selected bacterial strains by their growth in solid LB medium with kanamycin (50 mg L-1) and rifampicin (50 mg L-1) by PCR assays using primers that amplify the expression cassette and the cry1Ba1 gene.
Plant transformation
We carried out the phenotypic characterization of A. tumefaciens LBA4404, in vitro regeneration and transformation of 3 to 4-week-old potato seedlings following the protocols by Trujillo et al. (2001) and Jiménez et al. (2009). Regeneration occurred using internodal stem segments (5-6 mm length) with the expression cassette 5 (containing the CaMV35S promoter with the cry1Ba1 gene without C-terminal fragment and codon usage adjustment). The regeneration system allowed the formation of callus and the regeneration of the explants. The efficiency of the regeneration system was evaluated through the induction of callus, percentage of regeneration, and the number of regenerants per explant. The transformation of the seedlings proceeded based on a method proposed by López and Chaparro-Giraldo (2007) by taking groups of 40 explants and placing them in the co-culture medium, which consisted of liquid MS medium supplemented with vitamins and 20 mg L-1 of acetosyringone. The explants were placed in contact with the bacterial suspension for 8 min, using as a control a group of 40 explants not subjected to cocultivation and transferred directly to MS solid culture medium without the addition of hormones. We transferred the co-cultivated explants to Petri dishes with solid coculture medium and incubated them in the dark at a temperature of 20 ± 3°C for 18 h. Then, we transferred the explants to a regeneration medium supplemented with 250 mg L-1 of cefotaxime for 7 d, and transferred to the same medium supplemented with 50 mg L-1 of kanamycin as the selection concentration that has been used for explants of Pastusa Suprema (López and Chaparro-Giraldo, 2007; Torres et al., 2012) and other varieties (Andersson et al., 2003; Khan et al., 2006; Meiyalaghan et al., 2011; Molla et al., 2012).
Freedom to operate analysis
We performed a search for requests and conceded patents in the public access international database The Lens (https://www.lens.org/), using keywords relating to the elements of the designed cassettes. The analysis was centered on the claims of the patent requests. The Lens locates patents granted by the United States Patent Office and its European counterpart, as well as the records in the Patent Cooperation Treaty (PCT) that are in the World Intellectual Property Organization (WIPO) database. Later, we performed the search in the patent database of the Superintendencia de Industria y Comercio (SIC) (Colombian National Superintendence of Industry and Commerce).
Results and discussion
Selection of the different Cry1Ba1 sequences
We considered the mechanism of action of the Cry1 proteins in Lepidoptera in order to define the characteristics of the coding region for the Cry1Ba1 protein (Pigott and Ellar, 2007). The protoxins must be processed by insect proteases that cleave the protoxin polypeptide into specific sequences (Deist et al., 2014), and this is crucial for determining the mode of action of these proteins after ingestion of the crystal and the pH-dependent solubilization of the protoxin (for the orders Lepidoptera and Diptera this happens at basic pH, while, for the orders Coleoptera and Hemiptera, the pH is neutral to acidic). In the case of the Cry1A protein (~130-135 kDa), the cleavage results in an active toxin of 65 kDa, caused by trypsin (or similar to trypsin) and chymotrypsin-type proteases, where enzymatic digestion eliminates a sequence of ~600 amino acids at the C-terminus and the first 28 amino acids at the N-terminus. For the orders Lepidoptera and Diptera, the main intestinal proteases are of the serine type, while in Coleoptera, the main proteases are cysteine and aspartic acid proteases, although some use cathepsin G and also trypsin and chymotrypsin (serine protease). In the intestinal fluid of various insects, other proteases (elastase, pronase, thermolysin) have been identified and recognized for their possible interactions with Bt protoxins. The cleavage of the protoxin is a prerequisite for its insertion into the membrane. These interactions are complex, and the activation process in vivo is unclear and could vary because of differences in proteases in the intestines of insects (Miranda et al., 2001). The secondary structure of the toxin and the toxin receptors in the epithelial cells of the insect's midgut are important determinants of toxin specificity, and it is likely that the differential proteolysis of the protoxin by the intestinal juice of the different larvae may affect toxicity; this has been demonstrated in different species (Bah et al., 2004).
Considering the importance of the proteolytic activation of the Cry1Ba1 protoxin, determining the structure of the respective active toxin was vital. Exactly where each type of protease performs the cleavage depends on the type of Cry protein and the specific proteinase population of each insect (Pigott and Ellar, 2007). Since the mechanism of action of Cry1 proteins in T. solanivora is not completely understood, in this research we designed five expression cassettes that contemplate possible scenarios of the mechanism of action of the Cry1Ba1 protein in this species. To this end, we identified the functional domains of the Cry1Ba1 protein according to the NCBI database of conserved domains (https://www.ncbi.nlm.nih.gov/Structure/cdd/wrpsb.cgi), which corresponds to A) N-terminal I domain-amino acid 89, B) domain II-amino acid 267, and C) domain III-amino acid 489 (Fig. 1). This analysis contemplates putative processing by proteases that have been identified in other Cry proteins.
In silico design
The in silico design of expression cassettes permits changes in the nucleotide sequences that can improve the expression of the gene. The codon modifications are one of the most critical factors for achieving adequate levels of expression (Christey et al., 2006).
We describe the five expression cassettes designed (Fig. 2) in this work as follows: 1) Contains the cry1Ba1 gene without the C-terminal fragment and codon usage adjustment, it is formed by the first 1947 bp of the cry1Ba1 gene; this construct contains the B33 promoter; 2) Contains the cry1Ba1 gene without C-terminal fragment and codon usage adjustment in the first 87 aa, is composed of 1947 bp; 3) Contains the cry1Ba1 gene coding for protoxin with codon usage adjustment, conformed by 3687pb; 4) Contains the cry1Ba1 gene encoding the active toxin cleaved at the N-terminus and C-terminal with codon usage adjustment, conformed by 1647 bp that start at nucleotide 267 and ends at nucleotide 1908 of the cry1Ba1 gene; 5) Contains the cry1Ba1 gene without the C-terminal fragment and codon usage adjustment, it is made up by the first 1947 bp of the cry1Ba1 gene, it contains the constitutive promoter CaMV35S. The first four constructs contain the B33 promoter that has a high degree of specificity for expression in the tuber (Fig. 2).
All the designed expression cassettes contain the T-nos terminator. The sequence of the cry1Ba1 gene is of bacterial origin and has a lower content of guanine and cytosine (GC) than that reported for plant genomes (Li et al., 2016).
The modifications of codon usage allowed changing the content of GC to 42%, which is a value closer to the potato genome (42.4%). Cryptic splicing sequences and premature polyadenylation sites of the ORF were eliminated in each of the cassettes.
Phenotypic and molecular characterization of recombinant strains
After the transformation of bacteria with expression cassettes 1, 2 and 5 to establish the characterization protocol presented below, we planted by exhaustion in the respective selection medium. As a phenotypic characteristic, the non-transformed colonies showed no growth and the colonies positive for PCR were subcultured in a selection medium for subsequent cryopreservation at -80°C. We performed a PCR assay on the positive colonies transformed with expression cassettes 1 and 2 using the forward primer for the B33 promoter and the reverse primer for the T-nos terminator. We considered the colonies that had an amplicon of 3917 bp positive. We considered positive the PCR assay using the primer pair consisting of the forward primer for the promoter CaMV35S and reverse primer for the terminator T-nos with an ampli-con of 2602 bp. We confirmed the A. tumefaciens and E. coli colonies that showed growth by a PCR assay for a region of the crylBal gene, and subcultured in selection medium. The PCR result was considered positive when 200 bp amplicons were obtained (Fig. 3).
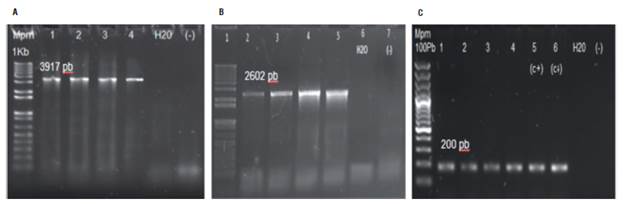
FIGURE 3 Molecular characterization of the recombinant strains of E. coli and A. tumefaciens. A) Amplification of expression cassettes 1 and 2, with the B33 promoter; B) Amplification cassette 5 with the CaMV 35S promoter; C) cry1Ba1 gene from the cassettes, negative controls (plasmid pCAMBIA 2300).
Transformation tests
We used the application of the transformation protocol to determine the infective capacity of A. tumefaciens LBA4404 on internodal segments of potato, leading to the subsequent introduction of the T-DNA with the gene of interest and its insertion in the genome of the plant. We evaluated the growth of regenerants in the presence of kanamycin with cassette 5 containing the nptII gene as a plant selection marker. The control group of regenerated explants without transformation with the expression cassette exhibited callus formation in the second week with a percentage of callus induction of 96%. That is, approximately 38 internodal explants of the 40 cultivated in regeneration medium, and a percentage of regeneration of 90%; approximately 36 explants regenerated with numerous shoots per explant of 4 ± 1. The untransformed explants cultivated in regeneration medium with selection antibiotic began to show oxidation at week one; during week two they presented oxidation and chlorosis in medium with kanamycin (50 mg L-l). Twenty percent of the cocultivated explants initiated the process of callogenesis, and by the second week they began to lose viability and exhibited complete oxidation. Eighty percent of the explants did not display callogenesis or regeneration; this could be associated with the effects on the plant genome at the time of coculture, physical damage, and the presence of the antibiotic. The explants transformed with the recombinant strain LBA4404 showed a behavior similar to that of the previous group, with the difference being that from the fourth week on, 10% persisted in the selection medium and produced regenerants. The resistance presented by the explants in the selection medium could be associated with the insertion of the nptII gene transferred by the recombinant strain LBA4404 containing the expression cassette 5 (Fig. 4).
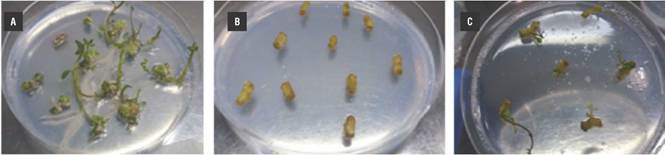
FIGURE 4 Application of the transformation protocol in potato explants using the recombinant strain of A. tumefaciens LBA4404. A) Untransformed explants in regeneration medium without kanamycin, showing processes of callogenesis and regeneration; B) Untransformed explants in regeneration medium with 50 mg L-1 of kanamycin, no regeneration was observed; C) Explants transformed with the strain LBA4404 in regeneration medium with kanamycin (50 mg L-1), regenerants were evident.
Employing a regeneration medium containing 50 mg L-1 of kanamycin as a selection agent has proven useful for the preliminary selection of possible transformants when using potato explants; they avoid selection pressure and loss of possible transformed explants by oxidation, chlorosis or tissue necrosis when higher concentrations of the antibiotic are used. This is demonstrated by some authors that have used concentrations of 100 mg L-1 (Andersson et al., 2003; Khan et al., 2006; López and Chaparro, 2007; Meiyalaghan et al., 2011; Molla et al., 2012; Torres et al., 2012).
Freedom to operate analysis
We identified patents relating to each of the genetic elements of the constructs beginning from the deconstruction of the product. The patent EP2482639A2 is within the patents relating to the cry1B gene, which is part of the application by PCT number WO2011041256A2 (Syngenta Participations AG). This protects nucleic acid molecules, which encode the Cry1Ba protein as well as modifications in its amino acid sequences, and the insertion of this with promoters such as patatin. Patent US20100235951A1 (Bayer Bioscience) includes plant cells or plants comprising a cry1C chimeric gene with cry1B or cry1D. The patent US8772577B2 (Pioneer Hi-Bred International, Inc.) protects a sequence that possesses 88% identity with the cry1Ba gene. In the search of the Colombian national database, we found no granted patents or patent requests for the cry1Ba1 gene. We found patent applications involving cry1B sequences, such as WO2011060009A2, whose claims protect a sequence possessing 88% identity with the cry1Ba, cry1Bc and cry1Bb genes. Another patent application is US20120324606A1 that includes plants that express cry1Ab sequences and cry1Be, has been requested in Colombia with the number CO6561804A2. However, this does not correspond to the variant of the gene used in this work (Cry1Ba1). Similarly, patent application US 20110277184A1 claims the cry1Bf sequence, a protein with an identity of at least 95% in plants. During the search for patents requested and approved in Colombia, we found patent CO6561804A2. This patent was requested nationally on June 16, 2012; it was denied because patenting of genes is not allowed in Colombia.
Concerning the promoter B33, patent EP0375092B1 was granted but it expired in 2009. The patent protected the use of this promoter in the production of potato plants. Patent US8334429 concerning the strain of A. tumefaciens was found (Pioneer Hi-Bred International, Inc.).
The patent US 20090075358A1 frames within its claims the general structure of the vector pCAMBIA. We found no patents protecting the use of the T-nos terminator sequence. Therefore, to date when the review was carried out, no intellectual property rights relating to the selected sequences in the design of the expression cassettes were registered in Colombia.
Conclusions
We selected the cry1Ba1 gene as a viable option for obtaining GM potato lines, according to the biological context of the mechanism of action of Cry1 proteins.
The in silico design of expression cassettes enabled the attainment of modified versions of the cry1Ba1 gene for transformation of potato plants, with modifications that allowed the desired levels of expression and lethal effects on the target insects. These studies should be carried out on the transgenic lines that we obtained.
To date, the freedom to operate analysis of the expression cassettes in this work, suggested that their use does not affect the rights of third parties in Colombia.