Introduction
Quinoa (Chenopodium quinoa Willd.) is considered a crop with great potential because of its high agronomic characteristics and nutritional value, and especially for its inclusion in children and elderly people's diets (Valcárcel-Yamany and Silva, 2012). According to Escuredo et al. (2014), this plant has the capacity to produce grains of high quality and protein content. Additionally, it contains amino acids such as lysine, threonine and methionine, which are considered as essential.
These nutritional characteristics are the result of environmental conditions, such as temperature, light intensity, relative humidity and precipitation. These conditions are key factors in the quality and number of grains per panicle (Morales et al., 2017), as well as in the phenological and physiological performance of the plants related to the adaptive capacity to diverse environmental conditions (Winkel et al., 2016). The plant has adaptive advantages that allow it to express a great productive potential. Quinoa (Chenopodium quinoa) has undergone diverse evolutionary processes, but it has been bred from crosses with Chenopodium carnosulum to acquire resistance to salinity problems, Chenopodium petiolare to obtain adaptability to droughts, and Chenopodium pallidicaule to receive tolerance to frosts (Jarvis et al., 2017).
The quinoa plant has the capacity to develop alternative metabolic plasticity (Bazile et al, 2014). This change is induced by conditions such as temperature, light intensity, nutritional status, relative humidity, and water availability (Morales et al., 2017). In addition, it is able to undergo phenological, morphological, and physiological changes known as phenotypic plasticity.
On the one hand, the soil conditions, weather, and nutrient availability are important factors in the morpho-agronomic performance of the crop. However, the physical, chemical, and microbiological characteristics are specific to each place and mark plant development and the composition, quality and quantity of the quinoa grain (Veloza et al., 2016).
Moreover, in Colombia, the most cultivated varieties of quinoa are Piartal and Tunkahuan which come from Ecuador, SL47 from Nariño, White from Jerico and White from Soraca and Boyaca (Ardila et al., 2006). These quinoa genotypes have an average productivity of1.5 and 2.6 t ha-1 depending on the variety the fertilization plan (Delgado et al, 2009) and the environmental conditions (García-Parra et al., 2017).
Furthermore, the quinoa is planted at a small scale in the provinces of Nariño, Cundinamarca, Cauca and Boyaca. In Boyaca, there are reports of crops in the central zone (2,538-3,031 m a.s.l.) grown from a mix of seeds that affects crop productivity and grain quality (Veloza et al., 2016).
In that order, it is necessary to evaluate the physiological performance and the composition of the quinoa grain in three types of fertilization and under the environmental conditions of the municipality of Oicata.
Materials and methods
The research was carried out from June 2016 to May 2017 in the municipality of Oicata (Boyaca, Colombia) with coordinates 5°22'48" N and 73°30'09" W and an elevation of 2,875 m a.s.l. The average temperature was 12°C, with 74.1% relative humidity and an average precipitation of 1018.9 mm per year (Tab. 1). The soil in which the experiment was established had Andic Dystrustepts and Vertic Haplustalf association (IGAC and UPTC, 2005).
TABLE 1 Climatic conditions at the experimental plot.

*Pluviometer data of Oicata at an altitude of 2,645 m a.s.l. Code 24030450 IDEAM.
**Data supplied by the weather station from IDEAM (Universidad Pedagogica y Tecnologica de Colombia).
White quinoa seeds from Soraca were used as plant material, which were stored by the Research Group Agricultura, Organizaciones y Frutos (AOF) for six months. Some of the fertilizers used were: Paz del Rio Fertilizer (PRF, Escoria Thomas, Colombia), Urea (U) and an organic fertilizer from The Agro-Ecological Farm Victoria (AEFV, Colombia).
The experiment area for the research was of460 m2, where four fertilization treatments with four replicates were established. These trials were performed based on the result of the soil analysis carried out by the Chemistry Laboratory of Soil, Water and Plants from Agrosavia (Tab. 2). The treatment T0 was the control, T1 was for the application of 6 kg of AEFV fertilizer, T2 was for the application of 3 kg of AEFV fertilizer plus 100 g of U and 50 g of PRF, and T3 was for the application of 200 g of U and 100 g of PRF (Tab. 3).
TABLE 3 Chemical characteristics of fertilizers.
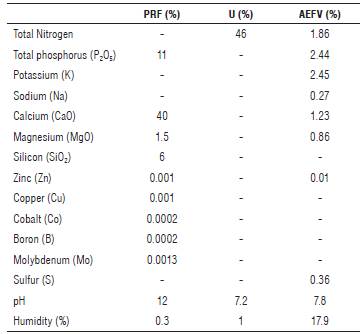
PRF: Paz del Rio fertilizer; U: Urea; AEFV: Agro-Ecological Farm Victoria fertilizer.
The response variables were: number of leaves, height of plants (rigid flex meter), days to reach six true leaves, and days to 50% flowering. In addition, days to milky grain and days to pasty grain state, chlorophyll (SPAD 502 plus, Konica-Minolta, Japan), dry and fresh weights of the plant (Drying oven HSY-75, 24 h at 104°C), grain productivity, protein amount (Kjeldahl Technique, NTC370), neutral detergent fiber in grain (Gravimetric determination, Van Soest AOAC 2002.4) and acid detergent fiber in grain (Gravimetric determination, Van Soest H2SO4) were measured every 15 d.
Statistical design
A completely randomized design with four replicates and 16 separate experimental units was established. The data obtained in the experiment were tabulated using Excel' program; a test of homogeneity of variety with the Bartlett method and a normality test with the Shapiro-Wilk method were performed. An analysis of variance (ANOVA) and a Tukey test of comparison of means with a 0.05 significance level were performed using the program R version 3.3.0.
Results and discussion
Number of leaves
As shown in Figure 1, significant changes, such as an increase in the number of leaves, were observed after day 75. However, after the start of the flowering stage (105 d), the plant showed a loss of leaves to its senescence. The highest average was found in the T3 treatment (90.75), which presented significant differences compared to the others, whereas T0 had the lowest average (32.75). All the treatments displayed a sigmoid performance. This performance was expressed in the exponentially development of the plant regarding the time (Taiz and Zeiger, 2007) taking into account that the plant induces, in the vegetative stages, the greatest number of foliar buds capable of capturing and housing the assimilates that will influence the reproductive stages and the formation of grains (Atencio et al., 2014).
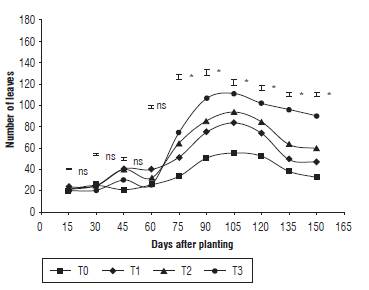
FIGURE 1 Number of quinoa leaves under different fertilization protocols in Oicata - Boyaca. T0: absolute control, T1: organic fertilizer, T2: organic fertilizer + urea + Paz del Rio fertilizer, and T3: urea + Paz del Rio fertilizer according to the Tukey test (P≤0.05). ns: not significant, *: significant for the day of sampling.
Although the number of leaves is a determining variable in the production of quinoa, it is not a key factor to crop yield. Similarly, the application of elements such as nitrogen stimulated the excessive development of fodder, which could affect the grain productivity (Kakabouki et al., 2018). Correspondingly, García et al. (2017) stated that the application of an increasing dose of N-P-K in quinoa enhanced leaf area but not productivity. Nevertheless, the opposite occurs when organicmineral fertilizer is used.
This kind of amendment facilitates the absorption of important elements for energetic, metabolic and enzymatic activities of the plant due to the microorganism content (Ramzani et al., 2017).
Plant height
Statistical differences (P<0.05) in height were recorded from day 75 for the T3 treatment. This contrast resulted in the highest average during the days 75, 90, 105, 135 and 150. The final average was 172.25 cm, compared to the absolute control treatment T0, which resulted in 93.25 cm (Fig. 2). Following day 120, the T2 treatment increased its growth due to the fertilization plan that was provided to each treatment. This increase could be due to the fact that nitrogen can stimulate plant elongation and cellular division, which allows the synthesis of auxin and cytokinins that have an effect on stem growth (Basra et al., 2014).
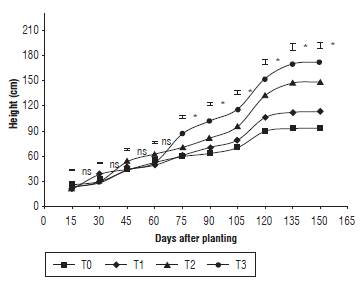
FIGURE 2 Height of quinoa plants under different fertilization protocols in Oicata - Boyaca. T0: control, T1: organic fertilizer, T2: organic fertilizer + urea + Paz del Rio fertilizer, and T3: urea + Paz del Rio fertilizer according to the Tukey test (P≤0.05). ns: not significant, *: significant for the day of sampling.
As a result, when more nitrogen is applied and there is more precipitation, an increase of the stem elongation is evident. According to Fghire et al. (2015), the continuous cell division and elongation increase the permeability of the root meristems and facilitates the absorption of structural minerals such as calcium, considered as fundamental in tissue rigidness and an active agent in the manifestation of plant hormones that stimulate the elongation and cellular division.
Chlorophyll content
This variable presented important statistical differences among days 90, 105, 120 and 130. T3 showed the highest average (66.4 SPAD units) in the closest phase to the flowering, at the moment in which the plant displayed the highest number of leaves. However, at 150 d, the treatments did not show significant differences (Fig. 3). This could be due to the fact that nitrogen application and chlorophyll content are directly proportional to each other (Liu et al., 2015), along with the application urea in treatments T2 and T3.
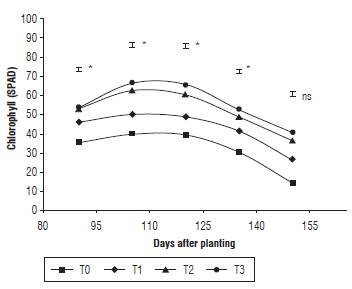
FIGURE 3 Chlorophyll content of quinoa under different fertilization protocols in Oicata - Boyaca. T0: Absolute control, T1: organic fertilizer, T2: organic fertilizer + urea + Paz del Rio fertilizer, and T3: urea + Paz del Rio fertilizer according to the Tukey test (P≤0.05). ns: not significant, *: significant for the day of sampling.
In addition, nitrogen determines the content of assimilates existing in the leaves, and consequently, the color of the leaf through which the chlorophyll meters act on the wave capture. Then, the high nitrogen applications result in high chlorophyll contents (Fghire et al., 2015), as observed in the experiment. For this reason, it is important to measure the chlorophyll content to evaluate the nutritional status of plants at foliar level (Rincón and Ligarreto, 2010).
Therefore, it is evident that the highest chlorophyll content is equivalent to vegetative phases and it is reduced in the reproductive phases (Fig. 3). According to García-Parra et al. (2018), the plant captures the highest amount of mineral elements in these phases, while in the filling and maturation phases, the nutrients are finally accumulated in the seeds.
Fresh weight
Each one of the evaluated organs presented significant statistical differences (P<0.05), which evidences that the T2 treatment had greater fresh weight in roots and leaves. In contrast, T3 displayed the highest average in the weight of the stem and panicle (Fig. 4). These results were obtained as a response to the implementation of mineral organic fertilizers (Garcia-Parra et al., 2017). Moreover, the high precipitations during the final phase of the vegetative stages and at the beginning of the reproductive stages generated the dilution of the phosphate fertilizer, which is indispensable for the development of diverse energetic activities. Similarly, the contribution of calcium is fundamental in the expression of plant hormones, which stimulate the development of leaves, stems and roots.
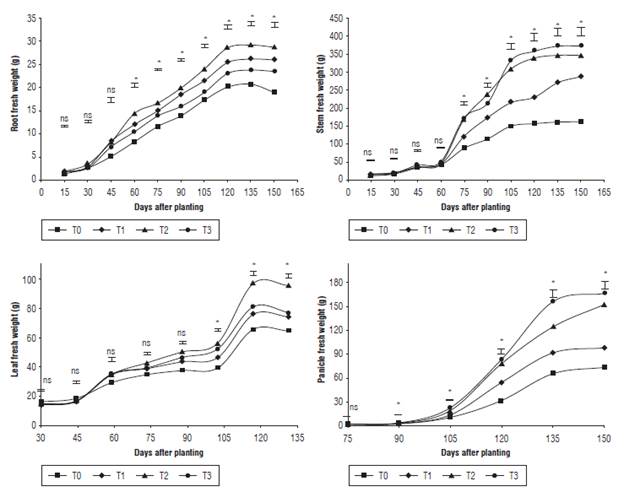
FIGURE 4 Fresh weight of stems, roots, leaves and panicles of quinoa under different fertilization protocols in Oicata - Boyaca. T0: control, T1: organic fertilizer, T2: organic fertilizer + urea + Paz del Rio fertilizer, and T3: urea + Paz del Rio fertilizer according to the Tukey test (P≤0.05). ns: not significant, *: significant for the day of sampling.
The fresh weight of plants is an indicator of efficiency in the uptake of nutrients and water (Torres et al., 2000), which can be influenced by environmental conditions. Thus, the biggest part of fresh biomass is found in the panicles followed by the stems, because the panicles support the leaves, glomerulus, and seeds, and are the duct for important substances in the nutrition of quinoa (Al-Naggar et al., 2017).
Dry weights
The plants treated with different fertilization protocols under the edaphoclimatic conditions of the municipality of Oicata presented significant statistical differences (P<0.05) regarding the dry weight of the roots, stems, leaves, and panicles. These results showed that the treatments T2 and T3 had the highest average during the entire test (Fig. 5) because of the implementation of the organic material that acts as a retainer of moisture (García, 2006). This facilitates the dilution of urea, Paz del Rio fertilizer, and minerals that are present in the AEF fertilizer, all expressed as assimilates in dry matter. In the case of T3, the increment occurred because of the application of soluble fertilizer and the increase of precipitation during the experiment.
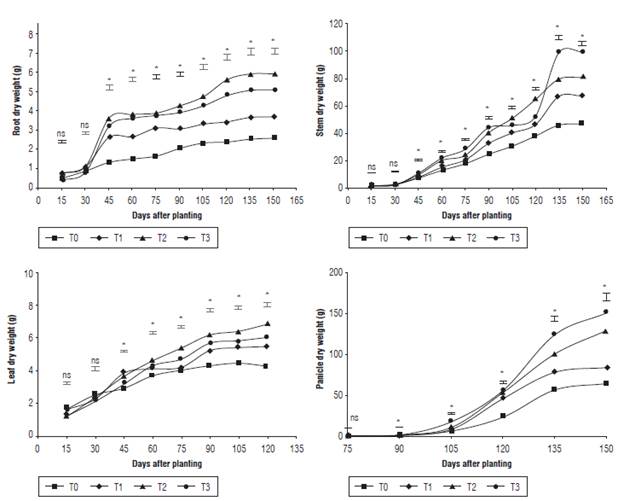
FIGURE 5 Dry weights of quinoa stems, roots, leaves and panicles under different fertilization protocols in Oicata - Boyaca. T0: Absolute control, T1: organic fertilizer, T2: organic fertilizer + urea + Paz del Rio fertilizer, and T3: urea + Paz del Rio fertilizer according to the Tukey test (P≤0.05). ns: not significant, *: significant for the day of sampling.
Furthermore, the dry matter is an indicator of the capacity that plants have to absorb nutrients (Magolbo et al., 2015). Aliro et al. (2011) stated that in the vegetative stages the highest dry matter accumulation occurs in leaves, while in grain formation the elements, minerals, and photo-assimilated compounds are transported from the source to the sink organs.
Nevertheless, the high indexes of dry weight do not certainly indicate either the productivity of the plant or the quality of its composition. It is due to the fact that quinoa expresses diverse potentials according to its variety and origin; the nutrients absorbed not only reach sink organs like seeds, but they are also stored in source organs as reserve, which are determining in the dry weight of leaves, roots and stems (Jayme-Oliveira et al., 2017).
Phenological performance
According to Table 4, the differentiation of the phenological performance started at flowering stage when treatments T1 and T2 took less time to get into their reproductive stages. However, after reaching the stages of milky and pasty grain, T0 presented a shorter productive cycle compared to T1, T2 and T3. This effect was due to the availability of nitrogen, which stimulated the longevity of the plant tissue, generating a constant production of new cells that are expressed in longer productive cycles.
TABLE 4 Phenological performance of quinoa according to the treatments.

Different letters in the same column indicate significant differences according to the Tukey test (P≤0.05). T0: absolute control; T1: organic fertilizer; T2: organic fertilizer + urea + Paz del Rio fertilizer, and T3: urea + Paz del Rio fertilizer.
The phenological response by the crop occurs through determining factors such as the soil and weather (Vargas et al., 2015). For this reason, quinoa plants allow to display longer productive cycles when fertilization plans are carried out with N-P-K in excess (Gómez and Aguilar, 2016).
Moreover, and regarding the climatic factors, the activity of the Rubisco activase (enzyme) declines as temperatures increase above the thermal optimum of photosynthesis. This loss of activity causes Rubisco deactivation, which in turn is proposed to reduce photosynthetic capacity at elevated temperatures (Raines 2011).
In addition, elements such as nitrogen determine how long the quinoa plant can be harvested (Geren, 2015). This growth effect is triggered when this element stimulates plant hormones that generate the production of foliar buds, which are indispensable in the photosynthesis process. It also composes the chlorophyll molecule that is found between 40-52 SPAD units in the vegetative phases (García-Parra et al., 2017).
Another determining factor is the edapho-climatic conditions during the establishment and development of the crop. The metabolic and phenotypical plasticity processes adapt to the water levels, sun radiation and relative humidity, which are all variables that intervene in the metabolic activities of the plant. These variables have allowed the plant to adapt to diverse regions of the world (Tabaglio et al., 2015).
Production and composition of the grain
Grain production did not show significant statistical differences (P<0.05). However, T1 showed a greater productivity per square meter, while protein, neutral detergent fiber (NDF) and acidic detergent fiber (ADF) had the best results in T2, T1 and T3, respectively (Tab. 5). The protein of T1 could be higher because it does not contribute to the available nitrogen despite of the organic fertilizer supply; the population of diazotrophic bacterium existing in this kind of fertilizer influences its availability in the plant (Parra-Cota et al., 2014). Additionally, a balance in elements like phosphorus, magnesium and other microelements contribute to the synthesis of protein (Marschner, 2012).
TABLE 5 Production and composition of the quinoa grain.

NDF: Neutral Detergent Fiber. ADF: Acid Detergent Fiber. Different letters in the same column indicate significant differences according to the Tukey test (P≤0.05). TO: absolute control, T1: organic fertilizer, T2: organic fertilizer + urea + Paz del Rio fertilizer, and T3: Urea + Paz del Rio Fertilizer.
On the one hand, quinoa production is determined by factors such as the availability of elements in the soil. For this reason, even though no significant differences were observed in the grain productivity, treatments T3 and T2 outweigh the amount reported by Delgado et al. (2009). Moreover, the amount of protein obtained is between the ranges established by Jacobsen (2003), which are between 13 and 18%. These ranges are higher in comparison to cereals such as rice (7.5%) and corn (13.4%) (Elsohaimy et al, 2015).
Regarding NDF content, the amount was higher in T1 seeds. However, the values for every treatment are higher in comparison to what was obtained by Peiretti et al. (2013), who reported values of 12.75%. The amount of ADF in the test was low compared to studies by Simranpreet et al. (2017), who reported 77.73%. These results were obtained because the composition and presence of fiber are influenced by the maturity stage of the seed (Reguera et al., 2018). The results in this test are related to the fact that when plants started the grain phenological stage, precipitations caused the elongation of the panicle that formed new seeds. This prolonged the phenological period of the plant to seven months; in other words, two more months than the phenological average of the variety in previous tests.
It is noteworthy that reports of ADF and NDF are very diverse in different tests reported in around the world. This could be happening because of the variety, maturity stage of the grain and fertilization, as referenced by Peiretti et al. (2013), whose results were 12.75% of ADF and 5.49% of NDF. On the other hand, Marmouzi et al. (2015) reported ADF of72.03% and ADF of27.06%, similar to the results of Simranpreet et al. (2017), with values of 77.73% and 27.4% for NDF and ADF, respectively.
Conclusions
Quinoa under the high Andean tropic conditions has a better reaction and performance reflected on grain productivity and quality when it is amended with organic and mineral fertilizers. Nonetheless, from a physiological activity perspective, the plant responded better to mineral fertilization. Even though the organic fertilizer did not contribute to the nitrogen available to the plant, the population of diazotrophic bacteria native to this environment is efficient in the contribution of nitrogen for plant development.