Introduction
Biochar is a solid, porous, carbon-enriched compound, produced by high temperature biomass thermal degradation under an inert atmosphere (absence of oxygen) through a thermochemical process known as pyrolysis (Itskos et al., 2016; Basu, 2018; Baskar et al., 2019). This process consists of simultaneous, successive and serial reactions that include dehydration, depolymerization, isomerization, aromatization, decarboxylation, and carbonization (Lee et al., 2019; Xiao et al., 2020). These stages are intertwined (Kan et al., 2016) and cover a complex set of reactions that involve the formation of radical groups (Odinga et al., 2020). Although it is widely considered as an independent biomass conversion technology, pyrolysis actually constitutes the first stage of the gasification and combustion processes (Levin et al., 2016). The main stages of pyrolysis have been generally described as: i) initial evaporation of free moisture, ii) primary decomposition, and iii) secondary reactions (oil cracking and repolymerization) (Uddin et al., 2018; Chen et al., 2019).
The complexity of the pyrolysis process is strongly related to the conditions of manufacturing such as the maximum process temperature, the particle size, the residence time (Zhang et al., 2019), the temperature/heating time relationship, pressure, etc. (Itskos et al., 2016; Leng & Huang, 2018; Szwaja et al., 2018; Sato et al., 2019). During this process, large chains of polymers and inorganic compounds, mainly made up of carbon, hydrogen and oxygen, decompose into smaller molecules in the form of gases, condensable vapors (tars and oils) and solid carbon (Kan et al., 2016). The diversity in composition of the biomass, depending on its origin, added to the conditions described above, will determine the speed and degree of decomposition of each of the components (Tabakaev et al., 2019) as well as their performance and quality (Intani et al., 2016).
Through direct and indirect effects, biochar can improve the physicochemical properties and quality of the soil, increase the productivity of crops, or help mitigate climate change (Cheng et al., 2018; Diatta et al., 2020). That is why biochar has managed to position itself as a key input within the wide range of alternative practices for more sustainable development, thanks to its versatility and potential in different fields such as agriculture or the environment. Some effects derived from the application of biochar to the soil are a reduction of greenhouse gas emissions and the promotion of carbon sequestration in the soil, making possible the rehabilitation of degraded soils (Bis et al., 2018; Gupta et al., 2020). Additionally, biochar reduces dependence on chemical fertilizers in crops by improving the physical, chemical and biological properties of the soil (pH, surface area, cation exchange capacity (CEC), particle density, humidity, and conductivity) (Burrell et al., 2016; Zwart, 2020), reducing the leaching of nutrients, or making a direct or indirect contribution of nutrients that exerts a promoting effect on plant growth and increasing crop yield (Ouyang et al., 2014; Sun et al., 2019). Biochar not only improves the physicochemical properties of the soil, but it can also have a positive impact on its biological properties, such as an increase in the abundance and diversity of beneficial soil microorganisms, since it provides a micro habitat that serves as protection against other pathogenic microorganisms (Liao et al., 2016; Ajema, 2018).
However, it is important to note that the type and magnitude of the effect on soil properties are associated not only with the chemical and physical properties of biochar (Weber & Quicker, 2018), but they can also vary remarkably depending on the type of soil where it is added. Therefore, the characteristics of the biochar and the type of soil are two important factors to consider before its use.
In this review, we provide detailed information about the different technologies, process conditions, and starting materials employed for biochar production and their influence on the final physico-chemical characteristics and practical applications of biochar. We also analyze the impact of biochar use in various applications, in areas such as the mitigation of climate change and agriculture, as well as the international regulations that govern biochar use.
A brief history of biochar
The origin of biochar goes back to the black earths, anthropogenic dark earths (ADE), or Terra Preta de Indio, as they were known by the indigenous populations that inhabited the Brazilian Amazon region (De Oliveira et al., 2020). These lands exhibit completely different properties to most soils in that region (Glaser & Birk, 2012). These soils are widely distributed throughout the region and are characterized by having a high content of nutrients such as carbon (C), phosphorus (P), calcium (Ca), magnesium (Mg), zinc (Zn), manganese (Mn) and organic matter (Barbosa et al., 2020). Its organic component does not come strictly from the accumulation of vegetal cover, but rather it has originated as a result of ancestral human practices as a method for the use of plant and animal bone remains, cooking coal, and waste from fires that were made to replace forests with farming lands (Costa et al., 2017; Macedo et al., 2017). The use of coal for promoting agronomic productivity has been known for centuries (Ogawa & Okimori, 2010), since it retains its stability and properties for hundreds of years. Also, it has proven to be an excellent soil amendment (Ye et al., 2020).
The term biochar was used for the first time in 1999 to describe an activated carbon prepared from sorghum grain and deployed in a process known as reverse-burn gasification (the ChemChar process) (Bapat et al., 1999). Later, it was incorporated into scientific literature on bioenergy to describe charcoal prepared from various crop residues to be used as fuel. The idea of using biochar as an alternative to mitigate climate change was conceived by different authors since 1993, long before the term was born (Paustian et al., 2016). However, it was not until the beginning of the 21st century that Johannes Lehmann associated biochar with the concept of climate change as a way to reduce greenhouse gas emissions (GHG); thus, biochar started to be considered as a soil amendment (Lehmann et al., 2006; Hyland et al., 2010).
Influence of pyrolysis process parameters and type of biomass on biochar characteristics
Biochar can be produced from a variety of biological, raw materials such as crop residues, decaying organic matter of animal origin (livestock manure, pigs, poultry, etc.) (Tag et al., 2016; Pariyar et al., 2020; Tomczyk et al., 2020). Regarding crop residues, the raw biomass is constituted mainly by carbohydrates formed from photosynthesis; the structures and compounds are very diverse depending on the type of plant. These characteristics also influence and significantly determine biochar properties (Li et al., 2020). The yield, ash contents, development and size of the pore, surface area, and even pH are just some of the characteristics that change considerably between the different types of starting material (Tan et al., 2017; Jafri et al., 2018).
Several types of biomass have been used to produce biochar with different purposes (Tab. 1).
TABLE 1 Different raw materials used to produce biochar and some areas of study and potential application.
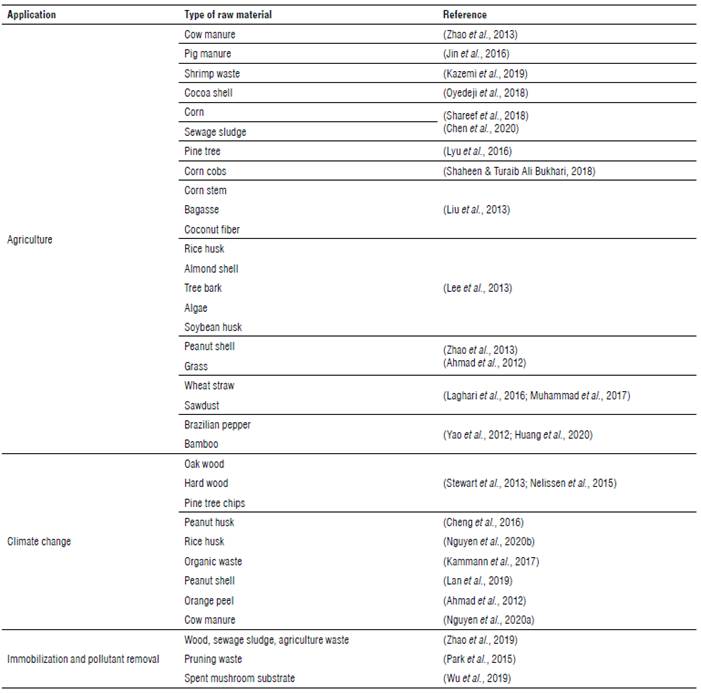
Lignocellulose, the principal component of plant biomass, is mainly constituted of three organic polymers: cellulose (40-60% w/w), hemicellulose (20-40% w/w) and lignin (10-25% w/w) (Yang et al., 2007). Each one of these polymers react differently during the heat treatment (Weber & Quicker, 2018), due to their different thermal stabilities that influence not only the required treatment temperature, but also the yield regardless of the chosen conditions (Tag et al., 2016; Uddin et al., 2018).
A large number of studies have shown that there are several interactions between the main components of biomass (Yu et al., 2017); therefore, it is not possible to predict the pyrolysis results simply based on the individual thermal behavior of the three components (Yaashikaa et al., 2019). The interaction between hemicellulose and lignin promotes the production of phenols, while it hinders the generation of hydrocarbons (Cao et al., 2018). Lignin also interacts significantly with cellulose by limiting the polymerization of levoglucosan which reduces the formation of biochar, while the interaction between cellulose and hemicellulose has less effect on the formation and distribution of pyrolysis products (Kan et al., 2016).
Low heating rates and long residence times are usually used to produce biochar. At higher temperatures, the carbon content in the final material increases. However, for vegetal residues high temperatures are not ideal due to the increase in ash contents (Rafiq et al., 2016). As temperature increases, water evaporation rises. Volatile compounds are then released, which, in turn, enlarges the relative fixed carbon ratio of the solid compared to the raw biomass (Jouhara et al., 2018). At the same time, there are variations in the carbon, hydrogen, and oxygen contents that lead to some physicochemical changes such as polarity reduction, aromaticity increase, and biochar hydrophobicity (Lehmann et al, 2009; Gray et al, 2014) 500°C, and 620°C. The surface area as well as pore volume also increase slightly due to the release of volatiles during carbonization. This process reconfigures the structure of lignocellulose compounds, giving rise to the formation of structures shaped as canals (Sigmund et al., 2017).
The thermal decomposition of the organic components in the biomass begins between 350-550°C with the formation of two structural fractions composed by the stacking of crystalline sheets of poly-aromatic graphene that grow laterally, and by an amorphous fraction of randomly organized aromatic structures (Weber & Quicker, 2018). Both of these fractions are associated with ring shaped carbon bonds of benzene types with oxygen or hydrogen (Kwiatkowski & Kalderis, 2020), that give rise to layers, forming the structure of a lattice or slit that originates multiple spaces corresponding to the pores. These bonds between aromatic structures of C-O and C-H determine the stability of biochar and are used as a parameter to measure the degree of aromaticity (Choudhary et al., 2019). They also contain oxidized and aliphatic carbon structures that are easily degradable.
The dominant process is carbonization, that occurs at between the 600-800°C, and causes the removal of most non-carbonaceous atoms such as calcium (Ca), magnesium (Mg), and potassium (K) (Zhang et al., 2017; Chatterjee et al., 2020). Hydrogen (H), oxygen (O), phosphorus (P), and sulfur (S) are mainly located within aromatic rings as het-eroatoms. The presence of heteroatoms contributes greatly to the reactivity of biochar and the chemical heterogeneity of its surface (Cheng & Li, 2018).
As a result, biochar with low H/C ratios, that corresponds to a higher degree of carbonization, contain fewer functional groups and more aromatic structures than biochar produced at low temperatures. O/C ratios are much higher than those present in mineral coal that also originates from biomass, but that is formed by geological processes in periods that include geological scales (Bakshi et al., 2020).
The pH of biochar also depends on the production parameters and type of biomass, which results in products with a wide range of pH values (Tag et al., 2016). When biochar is produced at temperatures below 400°C, it reaches a pH below 7, but at temperatures above 500°C, the pH can vary from 7 to more alkaline (Tomczyk et al., 2020).
Biochar production technologies
Within the diverse thermochemical processes that transform biomass into biochar, four methods are used: slow pyrolysis, fast pyrolysis, flash pyrolysis, and gasification (Novak et al., 2010; Ippolito et al, 2012).
Slow pyrolysis, or carbonization, is characterized by long residence times (hours to days) at relatively low temperatures (~300-700°C) and materials with different particle sizes (5-50 mm) (Claoston et al., 2014). In this type of pyrolysis, the thermal decomposition of biomass occurs at a very low heating rate (temperature/time), using enough time to maximize the solid yields thanks to repolymerization reactions. The previous method is used mainly to produce the solid fraction or coal, although it can also be gasified to obtain hydrogen-rich gas.
Fast pyrolysis generally involves high heating rates (>10-200°C sec-1) and short residence times (0.5-10 sec) (Claoston et al., 2014). It is used to obtain a high yield of liquid products, such as bio-oil, in relation to the dry biomass base, which can be as high as 50-70% by weight.
Finally, the pyrolysis process known as flash is characterized by very high heating rates (103-104°C sec-1) and much shorter residence times (<0.5 sec), resulting in very high bio-oil yields that can reach up to 75-80% by weight (Gilbe et al., 2008; Gao et al., 2011; Enders et al., 2012). In comparison to residual biomass, these materials offer greater chemical and biological decomposition resistance (Zimmerman, 2010) as well as a greater number of aromatic structures (Baldock & Smernik, 2002).
Biochar quality standards
In order to guarantee the safety of the use of biochar as a soil amendment to the market and especially consumers, the members of the International Biochar Initiative (IBI) in conjunction with research centers, scientists, farmers, and producers worldwide, developed guidelines and policies that provide the rules for the characterization of biochar products. All the standards are based on a series of guiding principles and the follow-up of guidelines consistent with the best practices for the development of standards such as the International Standards Organization (ISO), ASTM International, and the Institute of Electrical and Electronics Engineers (IEEE). Compliance with these standards by manufacturers is not mandatory. However, the IBI has implemented a certification program to expand the biochar industry since 2013. Through this program the producer can certify that the product complies with quality standards and that it is safe for use in soils. In addition, the IBI Certified TM quality seal is awarded, which is recognized worldwide (International Biochar Initiative, 2015).
At the same time, Europe has the European Biochar Certificate (EBC) that applies a system for the sustainable production of biochar based on the latest and most innovative research and practices. These practices aim to guarantee the production control and quality of biochar with processes that have scientific and legal support and that are economically viable and practically applicable. Unlike the IBI standards, the European certificate is mandatory for all producers (European Biochar Foundation, 2016). Currently, there are two documents approved by the members of the IBI and EBC that contain the technical programs, policies, and guidelines: IBI Biochar standards (version 2.0) and European biochar certificate guidelines (version 6.4E).
Biochar in agriculture
Great interest has been generated on biochar and its application to soils due to the similarity between biochar particles and those found in Terra Preta de Indio (Lehmann et al., 2011). Several studies have shown that the physical and chemical properties of biochar favor adsorption thanks to its high specific surface area compared to other organic amendments, thus improving the availability of nutrients in the soil (Bonanomi et al., 2017; Yadav et al, 2019).
The pH is one of the most important soil parameters because of its great influence on biological, chemical, physical, and geological processes, and because it is related to soil fertility (Neina, 2019). Changes in pH trigger a series of modifications in the soil environment, affecting the availability of nutrients for plant growth and microbial activity, or accelerating biogeochemical processes in the rhizosphere (Ducey et al., 2015). The addition of biochar as a soil amendment has become a common practice, as it improves soil quality by reducing soil acidity. Due to its porous matrix structure, it can also improve soil structure by forming micro aggregates, increase water retention, improve solubilization, nutrient retention and transport, enhance the soil quality index and reduce nutrient leaching (Martinsen et al., 2014; Wang et al., 2014; Pratiwi et al., 2016; Jeffery et al, 2017; Oladele, 2019).
Some properties of biochar, such as high surface charge density, large surface area, internal porosity, and the presence of polar and non-polar surface sites play an important role in the liming effect (Shetty & Prakash, 2020). The effect of pH on acidic soils is probably due to their alkalinity and high buffering capacity (Juriga & Simansky, 2019). The basic cations (e.g., K, Ca, Mg and Si) that are contained in biochar as carbonates or oxides can reduce the acidity by increasing the reaction of the exchangeable basic cations. This process is carried out through the functional groups on the surface of biochar as COO- and O- with the H+ or Al+3 ions in the soil, which would cause an increase in the soil pH (Hansen et al., 2016; Dai et al., 2017; Zong et al, 2018).
Another physicochemical property of the soil associated with its fertility is cation exchange capacity (CEC), which refers to the capacity of soils to retain cations interchangeably through adsorption (Duraes et al., 2018; Zhang et al., 2018). The CEC of biochar is mainly a consequence of the temperature conditions at which it is produced (Tan et al., 2017; Leng & Huang, 2018). The carboxylic groups formed in the bridges of the aromatic nuclei of biochar are responsible for the increase of the CEC and reactivity (Zhang et al., 2018). After biochar is added to the soil, the charges of its functional groups are generally positive, but over time the functional groups on the surface oxidize and generate more negative charges relative to the positive ones, increasing the CEC. Adsorption of highly oxidized organic matter on biochar surfaces is another process that affects CEC (Tomczyk et al., 2020). When biochar is exposed to oxygen and water, more functional groups can be generated on the surface through oxidation, thus increased CEC is attained (Hue, 2020). However, CEC is highly variable depending on the surface chemistry of biochar and tends to change once it is incorporated due to interactions with the environment (Hailegnaw et al, 2019).
These processes can directly or indirectly influence the improvement of other physical and chemical soil properties (Karimi et al., 2020). A positive effect of biochar on the productivity of the crops has been shown (Jin et al., 2016; He et al., 2017; Masud et al., 2020). The most significant changes have been observed in acid soil (Diatta et al., 2020), which suggests that its yield effect is similar to that produced by liming, accompanied by better water retention (Ahmed et al., 2016). The water retention capacity is mainly due to two factors: the large internal surface and the high number of residual pores in the biochar, where water is retained by capillarity, improving soil aggregation and structure. This increases the general porosity of the soil and the water content, leading to a decrease in the mobility of water, reducing water stress in plants (Batista et al, 2018).
Biochar improves the structure, porosity and aggregation of the soil, facilitating tillage (Du et al., 2018) and favoring the availability of nutrients (Karimi et al., 2020). Nutrient composition and availability from biochar depend on biochar raw material and pyrolysis conditions (Purakayastha et al., 2019). In addition, various factors affect the nutrient status in biochar-treated soils including the soil and feedstock type, pyrolysis temperature, and addition rate (Yu et al., 2019). Biochar can directly provide higher nutrient content such as P, K, Ca, Mg (Gao et al., 2017). Also, it can influence soil microbial activity due to changes in labile carbon and soil properties. The activity of soil microbes can significantly affect soil organic matter decomposition and nutrient cycling (Yu et al., 2019). The soil microbial biomass and enzyme activities have major impacts on the soil nutrient status and crop productivity. Another promoted benefit of biochar application to soil is reduced nutrient leaching (Zhao et al., 2019; Karimi et al., 2020).
Therefore, biochar does not act by means of a single effect. It influences different, interconnected soil physicochemical and biological properties. A highly variable response has been shown in the soil biota, reporting increases, decreases (Kolton et al., 2011; Li et al., 2018), or no significant effects (Lehmann et al., 2011). These interactions between microorganisms and biochar are also controlled by multiple environmental factors such as types and rates of biochar amendment, soil type, land use, and vegetation types (Gorovtsov et al., 2020).
Biochar as a matrix carrier for plant growth-promoting microorganisms
Peat is the most widely used inoculum carrier in the world; however, its availability is limited (Sahu & Brahmaprakash, 2016). Exploration of other matrix alternatives generated by more sustainable processes and that compete biologically and economically with the materials currently used could be beneficial to the biofertilizer industry (Herrmann & Lesueur, 2013; Flores-Félix et al., 2019; Saeid & Chojnacka, 2019). Biochar has the potential to be used as an alternative vehicle in the development of new biofertilizers. Its use as a carrier of plant growth-promoting microorganisms could favor both the proliferation of the microorganism of interest immobilized in it and the abundance of indigenous microorganisms (Ajema, 2018). However, it is important to consider that the raw material, the pyrolysis temperature, the degree of oxidation, and the size of the pores obtained during the production of biochar affect the characteristics of the final product and, therefore, its effect on microorganisms. The effects of inoculated biochar also vary according to the environmental conditions that directly affect the viability of microorganisms (Palansooriya et al, 2019).
The effects of biochar incorporation on soil microorganisms can be direct through the contribution of labile organic matter that provides a source of energy and nutrients. They can also be indirect, through changes in some soil properties, such as pH or porosity (Gorovtsov et al., 2020). The porous structure of biochar can act as a habitat for microorganisms. It can also increase the soil surface, which is able to retain water and nutrients favoring the growth of soil biota and rhizosphere microbiome (Jenkins et al., 2017; Husna et al., 2019).
The addition of biochar can increase mineralization through its contribution of labile organic matter. In general, biochar obtained through slow pyrolysis contains a greater amount of labile organic matter and tends to cause a greater increase in soil biota than more recalcitrant biochar (Cross & Sohi, 2011; Hardy et al, 2019).
Hale et al. (2014) show that survival but not abundance of Enterobacter cloacae (UW5), a well-studied bacterium that produces indole acetic acid (IAA) in a tryptophan-dependent manner, increases in soils amended with any of five different types of biocarbon (palm leaves, pine wood, coconut shell, pistachio shell, and fruit pits) when compared to peat and vermiculite. Ghazi (2017) evaluates the effect of Rhizobium-based formulations developed with biochar from rice husks on bean seeds, which were compared with peat and vermiculite materials. The biochar-based treatment records a maximum carrier survival population after 180 d of inoculation. In addition, a slight decrease in pH was recorded at the end of the storage period.
Biochar derived from acacia wood and inoculated with Azospirillum lipoferum (AZ 204) was tested at different dose levels (0, 5, 10, 15, 20, 25 t ha-1) and its effect on maize growth was evaluated. A significant increase was observed in Azospirillum and other diazotroph populations in the rhizosphere after the application of biochar at all the stages of crop growth. Furthermore, a significant increase of native mycorrhizal colonization was observed in response to biochar-Azospirillum application (Saranya et al., 2011).
Additionally, combinations of bacteria have been evaluated in the formulation of biofertilizers based on biochar, with the aim of finding sets of microorganisms that enhance plant growth through an interactive and harmonious work. The microbial consortium formed by endophytic bacteria (Brevibacillus, Enterobacter, Kytococcus, Pantoea, Pseudomonas, Serratia, and Stenotrophomonas) and fungi (Cutaneotrichosporon, Mucor, and Wickerhamomyces) isolated from rapeseed (Brassica napus) and barley (Hor-deum vulgare) were inoculated into a biochar derived from processed wood waste to evaluate its potential as a carrier of plant growth promoting microorganisms (PGPM) and its growth effect on barley seedlings. A synergistic effect of the microbial consortium with the biochar on root growth was observed. A decrease in urease activity in the soil was also found, without causing a negative effect on seedling development during the study (Vecstaudza et al, 2017).
Biochar has been recognized as a carrier matrix not only for microorganisms with a plant growth-promoting effect, but also as an alternative for the immobilization of microorganisms used in bioremediation. For example, biochar was used as a carrier of bacterial consortia in soils contaminated with cypermethrin, an insecticide for agricultural use (Liu et al, 2017).
Biochar can also play an important role as a carrier of bacteria used in biological control. In sugarcane, this strategy showed positive results for the control of the pathogen Macrophomina phaseolina by the antagonistic rhizobacterium Paenibacillus illinoisensis RH-3 (Shahjahan et al., 2018) . That study demonstrated that biochar derived from sugarcane bagasse is a better carrier of P. illinoisensis RH-3, compared to peat and lignite. The neutral pH of biochar together with its porosity and high availability of nutrients seemed to be determinants of its positive effect (Shahjahan et al., 2018).
All research on the potential of biochar as a carrier of microbial inoculum supports its use as an alternative to classical chemical fertilization. However, further studies are needed to elucidate the synergistic effects of biochar and soil microorganisms on plant growth promotion, their mechanisms of action, and the interactions between microorganisms and their microhabitats. Finally, such effects must also be validated under field conditions.
Biochar in climate change mitigation
Biochar was first recognized for its potential as a tool to counteract climate change in 2009 (Lehmann et al., 2009). Its porous structure and high internal surface area give it the capacity to absorb soluble organic compounds, gases, some inorganic compounds, and different pollutants thanks to the diversity of functional groups on its surface (Mendes et al., 2018). Biochar has been evaluated for the removal of heavy metals in contaminated waters such as lead (Pb), copper (Cu), zinc (Zn) and cadmium (Cd) (Shim et al., 2015; Wang et al., 2019; Zhao et al., 2019). The type of biomass and the temperature of the process are very influential for the absorption capacity of biochar. For example, materials produced at high temperatures result in materials with a larger surface area, higher carbon content and microporosity, leading to increased efficiency in removing compounds such as trichloroethylene (TCE), benzene (C6H6) and nitrobenzene (C6H5NO2) (Zhou et al., 2010; Ahmad et al, 2012).
The application of biochar to soils has been proposed as a strategy to reduce the concentration of CO2 in the atmosphere, as it would serve as a long-term carbon sink. Its recalcitrant nature, i.e., its resistance to degradation due to all the carbon present in its structure, gives it the ability to remain in soils for hundreds or thousands of years (Woolf et al., 2010) and to reduce the rate at which carbon fixed by photosynthesis returns to the atmosphere. It has also been suggested that carbon retention can be much greater when soil carbon stocks increase (Ding et al., 2018; Ventura et al., 2019) . However, some studies have found that many of the practices used to sequester carbon in the soil are often offset by increased greenhouse gas emissions (Shen et al., 2014; Zhang et al., 2019) and that soils show a low potential for carbon accumulation (Smith, 2016; Sharma, 2018; Gupta et al., 2020). However, among the possible strategies to remove CO2 from the atmosphere, biochar stands out in this area and currently represents the most promising alternative.
Biochar application can also reduce the emissions of nitrous oxide (N2O) and sometimes, the flow CO2 in soil, generating an additional tool to mitigate their effects (Woolf et al., 2010). However, the results have not always been positive in terms of CO2 reductions (Spokas et al., 2010).
Regarding the potential of biochar to reduce nitrous oxide (N2O) emissions, several hypotheses have been put forward on how biochar could interact with denitrification, by directly stimulating or suppressing the amount of N that passes into the gaseous form. These hypotheses relate this effect to several different biochar properties, soil type, and environmental conditions such as temperature and precipitation (Dicke et al., 2015; Lan et al., 2019). Although to date the impact of biochar on denitrification has not yielded conclusive results (Cayuela et al., 2014; Ameloot et al., 2016; Kammann et al, 2017), it appears that the magnitude and mechanisms associated with biochar inhibition of N2O depend on the dose and application frequency (Liu et al., 2020).
Biochar-induced changes in the composition or activity of the microbial community not only affect nutrient cycles and plant growth but also the soil organic matter cycle (Ouyang et al., 2014; Hardy et al., 2019; Gorovtsov et al., 2020). While research under controlled conditions has shown that biochar can affect N2O emissions from the soil (Taghizadeh-Toosi et al., 2011; Schimmelpfennig et al., 2014; Kammann et al., 2017), laboratory results cannot be extrapolated to what is expected in the field. In field trials, often no statistical differences are observed between biochar and control treatments. Despite the extensive literature published on the subject in recent years, it is still very unpredictable whether a type of biochar will be effective in mitigating N2O emissions in a field with crops. Factors such as application rate, biochar characteristics, soil type, environmental conditions, less homogeneous particle distribution, and greater soil (and plant) heterogeneity in the fields result in a high variability in N2O flows (Huppi et al., 2015). Therefore, most research efforts are now directed towards achieving the greatest reductions in N2O emissions by selecting the most efficient biochars and analyzing the mechanisms involved.
Conclusions
The addition of biochar to the soil provides different benefits derived from changes in the physical, chemical, and biological characteristics of the soil, which in turn, promote plant growth and productivity by increasing the availability of nutrients and pH. The porosity of biochar, along with water and nutrient retention, can promote the establishment and increase the abundance and diversity of microbiota which, in turn, promote plant growth.
The potential of biochar for carbon sequestration and its ability to reduce greenhouse gas emissions make it a very attractive alternative to counteract the adverse effects of climate change. However, further research is needed in this field to elucidate its long-term effects.
The wide availability of biochar as well as the varied responses in crops that will depend not only on the crop species but also on the type of soil, make it difficult to establish standard parameters regarding the dose and form of biochar application. For this reason, it is necessary to expand research in this field, since the wide availability of raw materials and the different technologies available for pyrolysis offer a wide range of opportunities for the production, marketing, and profitable use of biochar in Colombian agriculture.