Introduction
Nitrogen (N), an essential element for all living organisms, is the primary nutrient limiting crop production (Xin et al., 2014). Legume crops are less affected by N deficiency in soils due to their capacity for establishing simbiosis with N2-fixing bacteria collectively known as rhizobia that directly fix the atmospheric N2 in forms that can be assimilated by the plant (Kuypers et al., 2018). Symbiotic N2-fixation (SNF) capacity varies among legume species.
Compared to other legumes, the common bean (Phaseolus vulgaris L.) has lower SNF capacity (Polania et al., 2016).
The common bean is the most important legume crop for human consumption and the main source of protein for people in African and Central and South American countries (Broughton et al., 2003). Strategies to improve SNF capacity of the common bean include the selection of improved SNF common bean varieties as well as rhizobia strains efficient for different soil and environmental conditions (Farid et al., 2017; Oliveira et al., 2017), co-inoculation with rhizobia plus growth promoting bacteria (Hungria et al., 2013; Jesus et al., 2018), and the improvement of inoculants by the addition of biostimulating compounds (Lara-Acosta et al., 2019).
Oligosaccharins are biostimulating compounds widely used in agriculture; these compounds include chitin, chi-tosans, and oligogalacturonides (OGs). The OGs are linear oligosaccharin molecules of about 2 to 20 a-1,4-D-galact-uronic acid residues that are released upon fragmentation of pectic polysaccharides from the plant primary cell wall (Ridley et al., 2001). Oligogalacturonides are considered plant endogenous elicitors or bioregulators that may regulate the amount and action of plant hormones, thus modulating different developmental processes (Savatin et al., 2011). The positive effect of OGs has been reported for different crops (Camejo et al., 2011; Cabrera et al., 2013), but their effect on SNF-legume crops remains poorly analyzed. Recent work from our group aimed to analyze the effect of an OGs mix in Rhizobium-inoculated common bean plants (Lara-Acosta et al., 2019). This work demonstrated that the application of a mix of OGs, either onto the seeds when planting or by foliar spray in inoculated plants promoted nodulation and plant growth. For this reason, we hypothesized that an OGs mix may have a positive effect on the SNF process of common bean plants. Therefore, the objective of this work was to evaluate the effect of the application of an OGs mix on the common bean-rhizobia symbiosis. The Rhizobium tropici CIAT 899 strain used as inoculum is broadly used in commercial inoculants for application on the common bean in South America and Africa because it displays intrinsic resistance to abiotic and biotic stresses (Ormeño-Orrillo et al., 2012).
Materials and methods
The common bean Cuban (Mesoamerican) black-seeded Cuba Cueto-25-9-N cultivar was used in this research. The seeds, supplied by the Instituto de Investigaciones Fundamentales en Agricultura Tropical "Alejandro de Humboldt", Cuba (INIFAT), were surface sterilized (Ramírez et al., 2013). Subsequently, seeds were germinated over moistened sterile paper towels at 30°C for 2-3 d in darkness. The strain Rhizobium tropici CIAT 899, initially isolated from a common-bean nodule in Colombia (Martínez-Romero et al., 1991), was used as inoculum. The seedlings were planted in pots with sterile vermiculite and inoculated with 1 ml per plant of Rhizobium tropici CIAT 899 (1 x 1010 colony-forming units (CFU) ml-1) saturated liquid culture.
Inoculated seedlings were subjected to OGs treatment that consisted of a mixture of OGs with a degree of polymerization of 2 to 8 galacturonic acid residues obtained by enzymatic digestion with Pectinex enzyme (Sigma-Aldrich, Mexico City, Mexico) of commercial citric pectin (Cabrera et al., 2003) and characterized by MS analysis (MALDI) in a Q-Tof Premier mass spectrometer (Waters Corporation, MA, USA). An OGs mix was applied either to the seed (10 mg L-1, 1 ml per seed) at the time of sowing and inoculation or by foliar spray at a low (5 mg L-1, 1.5 ml per plant) or high (100 mg L-1, 1.5 ml per plant) concentrations onto plants with two trefoils. The OGs mix concentrations per treatment were selected based on data from Lara-Acosta et al. (2019) since these concentrations obtained the best results on increased root growth and nodulation from those tested in seed applications and foliar sprays.
Control and OGs mix-treated inoculated plants were grown in growth chambers under controlled environmental conditions (26-29°C, 16 h photoperiod, 4,800 LUX, and 60% relative humidity) and were watered with a N-free nutrient solution as reported by Summerfield et al. (1977). For the fertilized (non-symbiotic) condition, a full nutrient Sum-merfield solution was used for watering. Control and OGs mix-treated SNF common bean plants were harvested at 18 d post-inoculation (DPI) for comparative phenotypic analysis. The different parameters were determined from 10 plants (biological replicates) from the control and each of the OGs mix treatments.
Shoot and root lengths (cm) were determined from freshly harvested plants. Excess vermiculite was carefully detached from the roots by rinsing with tap water to avoid breakage of the root. Nodules were detached from the root and counted. Subsequently, roots, shoots, and nodules from each plant were dried in an oven (Laboratory Equipment BG, Mexico City, Mexico) at 60°C for 3 d and then weighed on an analytical scale (Sartorius GmbH, Göttingen, Germany) to calculate the dry weight (DW, g). Nitrogenase activity was determined by the acetylene reduction assay (ARA) (Hardy et al., 1968) in detached nodulated roots. Specific activity of nitrogenase was expressed as μmol C2H4 h-1 g-1 nodule DW per plant.
Nodule transcript levels of the genes coding for ammonium assimilation enzymes glutamine synthetase (GS) and NADH-dependent glutamate synthase (NADH-GOGAT) were analyzed by quantitative reverse transcriptase poly-merase chain reaction (qRT-PCR). Total RNA was isolated from 200 mg of frozen nodules using Trizol reagent (Life Technologies Corporation, Frederic, MD, USA) following the manufacturer's instructions. Three replicate RNA samples were isolated for each treatment. cDNA was synthesized using the RevertAid H Minus First Strand cDNA Synthesis Kit (Thermo Scientific, Waltham, MA, USA) from 1.5 mg total RNA, according to manufacturer's instructions. Resulting cDNAs were then diluted and used to perform qRT-PCR assays using SYBR Green PCR Master mix (Applied Biosystems, Foster City, CA, USA). Reactions were analyzed in a real-time thermocycler (7300 Real-Time PCR System, Applied Biosystems, Foster City, CA, USA) with settings of 50°C for 2 min, 95°C for 10 min, and 40 cycles of 95°C for 15 s and 60°C for 60 s. The primers used for qRT-PCR amplification of GS1 (Phvul.001G229500.1) and GOGAT (Phvul.001G076400.19) cDNAs were designed from the coding sequence of each gene obtained from the Phaseolus vulgaris v2.1 genome sequence available in the Phytozome data base (https://phytosome.jgi.doe.gov/pz/portal.html). The sequences of primers were the following: for NADH-GOGAT: Forward 5'-ACCAGGAGGTTGTGGATTTT-3' and Reverse 5'-TTTTTGCTTTCCTTCCTTCG-3', and for GS Forward 5'-GGAGCATATCGCTGCTTATGG-3' and Reverse 5'-AGTTTCGTGTCGTCCTGTCAGA-3'.
The relative expression level for each sample was calculated using the comparative Ct method and normalized with the geometrical mean of three housekeeping genes: ubiquitin (UBC, Phvul.006G110100.1), malate dehydrogenase (MDH, Phvul.007G273500.1) and heat-shock protein (HSP, Phvul.001G039700.1) (Hernández et al., 2007).
The SNF effectiveness for inoculated plants (control or each OGs treatment) was calculated using the following formula:
The plant DW was determined by adding the weights of dried roots, shoots, and nodules from each plant. Ten inoculated plants from each treatment and 10 fertilized (full nutrient) plants were analyzed.
Statistical analysis for parameters growth, nodulation, nitrogenase activity and the gene expression was performed using the Student's t-test (P<0.05). The SNF effectiveness data were analyzed by ANOVA using SPSS v22 (IBM Corp., USA). Differences between means were evaluated using the Tukey's honestly significant difference test (P<0.05).
The experiment was carried out under a completely randomized design with 10 replicates and was repeated on two occasions. In the article, only the results of one experimental repetition are shown given the similarity in the behavior of the results.
Results and discussion
Figure 1 shows data of growth and nodulation from 18 DPI inoculated common bean plants subjected to the different OGs mix treatments compared to untreated control plants. The values for shoot/root growth showed positive effects on inoculated plants subjected to foliar sprays of an OGs mix. Shoot length increased 37% and 35% and root length increased 25% and 15%, with foliar sprays of an OGs mix at low or high concentrations, respectively. However, shoot/ root growth in plants from OGs mix-treated seeds was similar to that from control plants (Fig. 1A-B). The OGs mix-treated plants showed similar shoot biomass compared to control plants (Fig. 1C). Regarding root biomass, a positive effect was observed in plants subjected to the two types of OGs mix treatments, showing increases of 54%, 45% and 55% in root DW after seed or foliar sprays of an OGs mix at low or high concentrations, respectively (Fig. 1D).
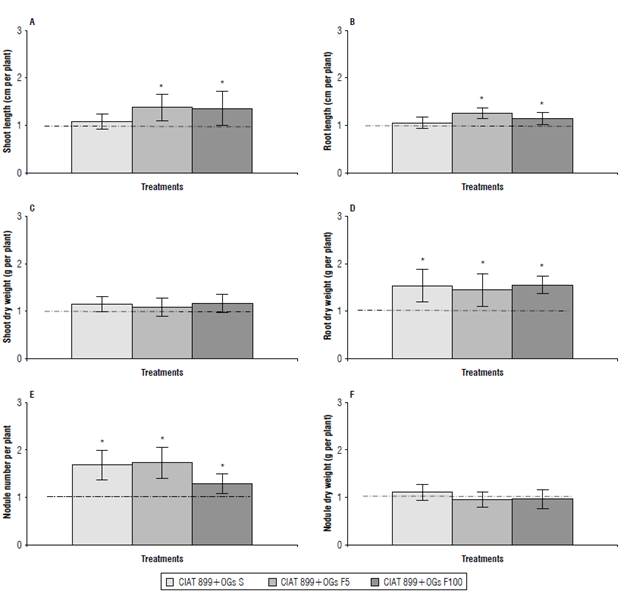
FIGURE 1 Effect of a mix of oligogalacturonides (OGs) on A) shoot length, B) root length, C) shoot dry weight, D) root dry weight, E) nodule number, and F) nodule dry weight of Cuba Cueto-25-9-N-common bean plants. The OGs mix treatments applied to Rhizobium tropici CIAT 899-inoculated plants were: CIAT 899+OGs S: seed application (10 mg L-1), CIAT 899+OGs F5: foliar spray at 5 mg L-1 and CIAT 899+OGs F100: foliar spray at 100 mg L-1. Parameters were evaluated in 18 d post-Inoculation plants. Values were normalized to the value from the control condition (inoculated plants without OGs mix addition) that was set to 1 as Indicated by a dashed line. Values represent the average ± SD from 10 different plants per treatment. Student's t-test was used to analyze the difference in each parameter compared to the control treatment. Columns marked with an asterisk (*) represent significantly different means according to the statistical analysis (P<0.05).
As observed in previous studies (Lara-Acosta et al., 2019), the OGs mix-treated plants showed an increase in nodule number of 68%, 73% and 29% in plants subjected to seed or foliar sprays of an OGs mix at low or high concentrations, respectively (Fig. 1E). However, OGs-treated plants showed similar nodule biomass compared to control plants (Fig. 1F).
The observed effects of the OGs mix in root and shoot growth of common bean plants may be correlated with altered auxin synthesis and concentration or location in common bean plants (Izquierdo et al., 2016), although the mechanism underlaying this effect has not been described yet. Auxin is the major hormone governing lateral root formation and primary root growth in plants (Bensmihen, 2015). The increased nodule number determined for SNF common bean plants treated with an OGs mix could be related to auxin signaling. The bacterial infection and nodule organogenesis in the Rhizobium-legume symbiosis is finely regulated by different phytohormones, including auxin (Bensmihen, 2015). The role of auxins in nodule initiation is due to its effects on both lateral/hairy root and nodule development (Kohlen et al., 2018). Barraza et al. (2018) reported that common bean plants with increased synthesis and concentration of indole-3-acetic acid have increased the number of nodules per plant.
Bacteroid nitrogen fixation and nodule cell ammonia assimilation are important aspects of nodule function. Thus, we assessed nodule function of OGs mix-treated plants by evaluating the nitrogenase activity and the expression level of genes coding for the GS and NADH-GOGAT enzymes that catalyze initial ammonia assimilation (Temple et ah, 1998). The highest values of nitrogenase activity were observed in the plants from seeds treated with an OGs mix and plants with foliar sprays of an OGs mix at a high concentration with an increase of 19% and 27%, respectively (Fig. 2A).
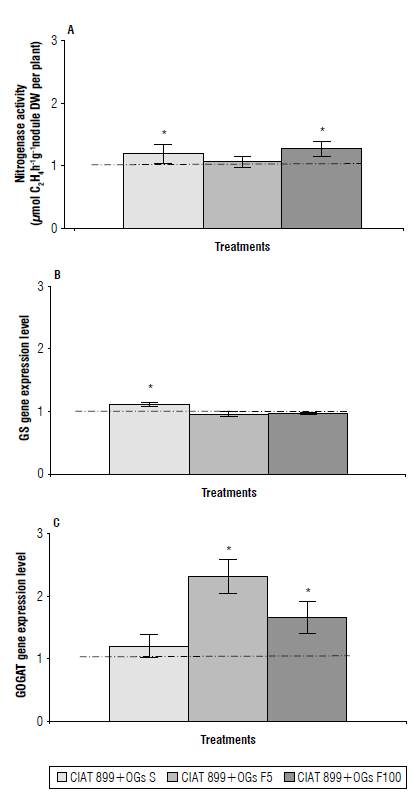
FIGURE 2 Effect of a mix of oligogalacturonides (OGs) on A) nitroge-nase activity, B) GS gene expression level, and C) NADH-GOGAT gene expression level of symbiotic N2-flxation (SNF) Cuba Cueto-25-9-N-common bean plants. The OGs mix treatments applied to Rhizobium tropici CIAT 899-inoculated plants were: CIAT 899+OGs S: seed application (10mg L-1), CIAT 899+OGs F5: foliar spray at 5 mg L-1 and CIAT 899+OGs F100: foliar spray at 100 mg L-1. Parameters were evaluated from 18 d post-inoculation plants. GS - glutamlne synthetase. NADH-GOGAT - NADH-dependent glutamate synthase. Values were normalized to the value from the control condition (Inoculated plants without OGs mix addition), that was set to 1 as indicated by a dashed line. Values represent the average ± SD from 10 different plants per treatment. Student's t-test was used to analyze the difference In each parameter as compared to the control treatment. Columns marked with an asterisk (*) represent significantly different means according to the statistical analysis (P<0.05).
Although a higher nitrogenase activity could be related to increased nodulation, it can also be related to the positive effect of the OGs mix on photosynthetic activity since sucrose derived from photosynthesis is the main carbon source for nodule function (Roy et al., 2020). OGs treatments increase stomatal density in banana leaves, thus favoring photosynthetic activity through improving gas exchange and decreasing water loss by transpiration (Izquierdo et al, 2016).
The GS gene expression level was only increased (11%) in plants from OGs mix-treated seeds (Fig. 2B). However, NADH-GOGAT gene expression level showed increases of 130% and 65% in plants with foliar sprays of the OGs mix at low and high concentrations, respectively (Fig. 2C). Nodule NADH-GOGAT is considered the limiting step of the GS/ NADH-GOGAT ammonia assimilation cycle (Temple et al, 1998; Roy et al., 2020). Nodules from inoculated alfalfa plants silenced for NADH-GOGAT gene expression show a strikingly altered symbiotic phenotype including reduced nitrogenase activity (Cordoba et al, 2003).
Based on the data from Figure 1, which indicate the positive effects of an OGs mix on Rhizobium-inoculated common bean plants, we evaluated their SNF effectiveness by comparing plant biomass of N-fertilized plants (DW = 0.998 ± 0.08 g) with biomass of inoculated plants treated with an OGs mix and control-plants. While inoculated common bean plants showed 50% SNF effectiveness, the OGs mix-treated plants showed increased SNF effectiveness to 60% (Fig. 3).
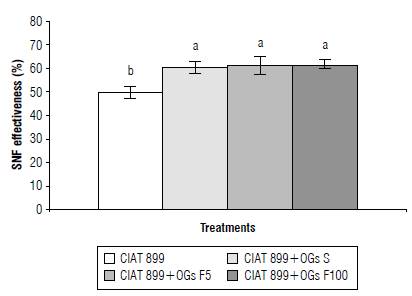
FIGURE 3 Effect of a mix of oligogalacturonides (OGs) on symbiotic N2-fixatlon (SNF) effectiveness of Cuba Cueto-25-9-N-common bean plants Inoculated with Rhizobium tropici CIAT 899. The SNF effectiveness, or the dry weight (DW) from Inoculated plants compared to DW from fertilized plants, was determined at 18 d post-Inoculation. CIAT 899: control R. tropici-inoculated plants. The OGs mix treatments applied to Rhizobium tropici CIAT 899-inoculated plants were: CIAT 899+OGs S: seed application (10 mg L-1), CIAT 899 + OGs F5: foliar spray at 5 mg L-1 and CIAT 899 + OGs F100: foliar spray at 100 mg L-1. Values represent the average ± SD from 10 different plants per treatment. Different lowercase letters Indicate statistically different groups according to the Tukey test (P<0.05).
The increase in effectiveness of SNF in inoculated common bean plants and treated with an OGs mix is interpreted as the result of the positive effects of OGs on nodulation, nitrogen fixation, and nodule function. A greater number of nodules guarantees a greater number of sites to fix atmospheric nitrogen (Haag et al., 2013). In addition, the higher nitrogenase activity increases the availability of NH4 + for the synthesis of glutamate due to the action of the enzymes glutamine synthetase and glutamate synthase (Chalk et al., 2017), which will be used by the plant for its growth.
Conclusions
The application of a mix of OGs with a polymerization degree from 2 to 8 galacturonic acid residues shows a positive effect on Rhizobium tropici CIAT 899-inoculated common bean plants. The OGs treatment can constitute an ecologically and economically suitable alternative for improving SNF effectiveness in the common bean. The positive effect of an OGs mix on root growth, nodulation and nodule function could guarantee an adequate growth and development that would translate into grain yield increase of this legume.