Introduction
The potato has been recognized as a key product for providing food security for the growing human population, particularly in developing countries (Devaux et al., 2020). This food crop stands out among others because it is an accessible source of nutrients (Wijesinha-Bettoni & Mouillé, 2019) that grows in a wide range of environmental conditions (International Potato Center, 2017), and its commercial value is resilient to price volatility at the global level due to its local production and distribution (Campos & Ortiz, 2019). Therefore, potato crops are expected to strengthen a sustainable capacity to supply sufficient human nutrition. Nevertheless, there are important challenges that must be overcome to ensure environmental sustainability. One of the biggest issues in potato production is to optimize the use of fertilizers to minimize the negative impacts of current fertilization practices on the environment (Tilman, 1999). Potato is a species with high nutritional demand per kg of produced dry mass. A commercial crop of diploid potato extracts, in a productive cycle, 124 kg ha-1 of nitrogen (N), 25.4 kg ha-1 of phosphorus (P) and 258.2 kg ha-1 of potassium (K) (Suarez &Torres, 2014). The demand for nutrients implies high fertilization doses to sustain high crop yields (Rajiv & Kawar, 2016). Consequently, potatoes are one of the crops with the highest application doses using 243 kg ha-1 of fertilizers (FAO, 2006) for a production of 370 million t over more than 17 million ha around the world (FAOSTAT, 2019). Locally in Colombia, applications doses in commercial diploid potato are around 833.3 kg ha-1, and the nutrient doses applied of added fertilizers are around to 125 kg ha-1 N, 54.6 kg ha-1 P, and 104 kg ha-1 K (Alvarado & Ramirez, 2018), exceeding those reported by FAO (2006).
Despite the increase in yields per unit area achieved with the application of chemical fertilizers (FAO, 1981), only 3035% of the N, 18-20% of the P and 35-40% of K present in the chemical mixtures are actually absorbed and used by the plants in agricultural crops (Subramanian et al., 2015), meaning that more than half of the applied fertilizers are quickly lost into the environment through runoff, leaching, and/or volatilization (Huang et al., 2017). The non-absorbed N and P are the main drivers of serious environmental problems such as water pollution, eutrophication-caused reductions in biodiversity (Whitters et al., 2014; Diatta et al., 2020), global warming (Bouwnman et al., 2002), and reductions in the ozone layer (Molina-Herrera et al., 2016). Unless there is a rise in fertilizer efficiency, a significant increase in NPK fertilizer application is expected by 2050 due to the increasing demand for food (Drescher et al., 2011) triggering a greater negative impact on the environment.
One of the strategies for facing this issue is to optimize the use of fertilizers in potato crops by implementing controlled release fertilizers. This technology maintains constant slow rates of nutrient release into the soil allowing synchronization between the onset of nutrient uptake by the plants and availability of nutrients (Naz & Sulaiman, 2016). Meanwhile, this technology reduces leaching by rain or irrigation water, mitigating eutrophication and the release of greenhouse gases into the atmosphere (Cong et al., 2010). To achieve controlled release, fertilizers are encapsulated in mineral and organic polymers known as coating agents (Azeem et al., 2014; Ali & Danafar, 2015; Guilherme et al., 2015). However, the materials used in the coating agents are often non-biodegradable, costly, toxic, and inconsistent in their release patterns and rates (Azeem et al., 2014; Naz et al., 2016). Recently, a carrageenin based hydrogel (CBH) has been proposed as a new encapsulating agent for fertilizer granules. This has extra advantages: its main component is carrageenan, a sulfated polysaccharide from the wall of red algae Hypnea musciformis (Wulfen) J.V. Lamouroux (Rozo et al., 2019); the alga is abundant in the coasts and widely distributed throughout the tropics and warm temperate seas in the eastern and western Atlantic. This alga is found in Southeast Asia (Ang et al., 2014), the Philippines (Lastimoso & Santianez, 2021), Asia (Titlyanova et al., 2016), the southern China (Phang et al., 2016), and the Caribbean (Camacho & Montana-Fernández, 2012). In addition, this natural hydrogel is relatively simple in structure and chemical composition, porous, semi-permeable, easy and inexpensive to synthetize, biodegradable and nontoxic (Blakemore, 2016; Hilliou, 2021; Guo et al., 2022). Fertilizers coated with urea and acrylamides have existed since the 1960s. However, since 1996 alginate and chitosan biopolymers were developed with a different synthesis technology than the one used for the CBH (Fertahi et al., 2021). Chitosan has shown good results in corn (Kumaraswamy et al., 2021). The use of kappa carrageenan as a coating material is still very new and there are still no studies evaluating this material as a coating in the field.
The CBH is a natural material that seems to have high potential as a coating to optimize fertilizers, ensuring the same yields while minimizing negative impacts in the environment; however, its efficiency at the field scale remains unexplored. Although it is known that the charges in its structure may have a natural potential to retain cations, it has not yet been explored whether, once the ions are retained, they are released from the hydrogel and absorbed by the plant. Knowledge of the integrity of the gel under the environmental conditions of the crop is absent but it is known that the encapsulating fertilizers with the CBH significantly reduced the N-NH4+ and K+ in leachates from laboratory soil column experiments carried out with a soil from an Andean potato crop (Santamaría et al., 2019). The CBH did not have a major impact on the P leaching because in these soils this element is not leached. In general, potato crops in Colombia are in soils with high iron and aluminium content and a pH ranging between 4.5 and 6.0 (FAO, 2019), inducing the P in the fertilizer to absorb by the Fe/Al oxides (Hanyabui et al., 2020); therefore, P leaching is low. Furthermore, CBH encapsulation did not have a negative impact on the growth and quality of potato (Solanum tuberosum L., Phureja Group, cv. Criolla Colombia), since the encapsulated fertilizer was as effective as the non-encapsulated in green house experiments with plants cultivated in pots with soil (Santamaría et al., 2019). These results look promising; however, in order to propose the CBH as an environmentally friendly alternative to be implemented in potato crops, an evaluation of its efficacy in field experiments is necessary. The objective of this study was to test whether the CBH coating around granulated fertilizer could reduce NH4 + and K+ leaching without impacting plant growth, specific gravity, and yield of a commercial potato crop.
Materials and methods
Study area
This study was conducted in a Solanum tuberosum L. crop at the San Isidro farm in the municipality of Sibaté (4°25'42"N; 74°17'58.4"W), located at 2720 m a.s.l. (Cundinamarca, Colombia) that supplies local markets of the Cundinamarca region. The production cycle took place between August and December of 2018, a period with an average temperature of 12.7oC and a maximum/minimum of 15.3/7.1oC. Collected soil samples were analyzed at the CIAT analytical laboratory for the physicochemical properties of the top (0 to 15 cm) soil layer (Tab. 1). Based on the levels of nutrient availability, the soil had a deficit in the amount of assimilable nitrogen (16.8 kg ha-1), since 1 ha of the cv. Criolla Colombia potato extracts on average 124 kg of nitrogen (Suarez &Torres, 2014). Both phosphorus and potassium are present in optimal concentrations with respect to the concentration requirements of the crop (Suarez & Torres, 2014).
Experimental design
Three treatments were compared in a randomized block design with three replicates for a total of nine experimental plots of 15 m2 each. Each plot was planted with two rows spaced 1 m apart and 25 plants per row with 0.3 m between plants in a row for a population of 33,333 plants ha-1. The treatment of the plot T1 was fertilized with the nonencap-sulated fertilizer and the treatment plots T2 were fertilized with the CBH hydrogel encapsulated fertilizer. A control treatment T3 was established with no fertilizer added to the plots. We used cv. Criolla Colombia (S. tuberosum L., Phureja Group), because this is the most cultivated diploid variety in Cundinamarca, with 120 d of cultivation cycle at 2600 m a.s.l (Ñústez & Rodriguez, 2020) and because of its excellent nutritional attributes (Thomas et al., 2021) and high required fertilizer applications (Alvarado & Ramirez, 2018), demand the design of innovative management strategies to reduce environmental impact. Also, the Phureja group is diploid and displays superior performance of agronomic traits, unlike Solanum tuberosum L. that is autotetraploid (Tai & Xiong, 2003) and has restrictions in the advancement of genetic improvement (Camadro & Mendiburu, 1988).
Fertilization treatments
Field preparation and management practices followed those used at the San Isidro farm. The land was dredge plowed and the furrows were constructed using a manual hoe. Fertilizer treatments with and without coating, consisted of 25 g of a granulated fertilizer (Vecol 15-15-15-11 with N 15%, P205 15%, K2O 15%, S 11% (Phosagro, Rusia) that contains only ammoniacal N) applied once per plant at the time of sowing. This amount corresponded to 833.3 kg ha-1. In T2, the 25 g of fertilizer were distributed in 11 capsules of the CBH hydrogel. The capsules had a cylindrical shape of 15.4 cm3 and contained 2.27 g of fertilizer (14% w/v). The encapsulated fertilizer was prepared as described in Santamaria et al. (2019). For both treatments, the 25 g of fertilizer were placed at 10 cm around the seed (Fig. 1). Finally, fertilizer and seed were covered with a layer of about 5 cm of soil. Selected seed tubers, about 5 cm in diameter, of the cv. Criolla Colombia were obtained from farmers of the region.
Rainfall was recorded daily using a conventional rain gauge. Precipitation registered a total of 481 mm during the production cycle. This precipitation corresponded to the second rainy peak at this locality. Supplemental irrigation was not performed.
Soil leachates
To collect leachates containing the nutrients not absorbed by the roots, three suction lysimeters were installed (SSAT Model, Irrometer Company, Inc., USA) the planting day in each experimental plot. Lysimeters were installed at 40 cm depth to ensure that the sampling of soil draining water not absorbed by the plants. At this depth, the leachates have moved beyond the reach of the roots that are typically 30 cm long in the cv. Criolla Colombia (C. Ñústez, personal communication, February 15, 2019). Lysimeters were placed next to the plants. Leachate volumes were sampled weekly up until 50 d after sowing (das). This time interval included the phenological growth stages of germination (BBCH 0), leaf development (1), formation of lateral shoots (2), longitudinal growth (BBCH 3), development of harvestable vegetative parts (BBCH 4), and appearance of floral organs (BBCH 5) based on the BBCH scale of phenological growth stages (Hack et al., 1993). Their content of NH4+ and K+ was quantified in the Analytical Services Laboratory of the International Center for Tropical Agriculture (CIAT). The NH4 + was analyzed by the automated spectrophotometry method using a Skalar Sanplus Analyzer SA 3000/5000 Mode 5000-01 (Skalar Analytical B.V, Netherlands) and atomic absortion spectrophotometry (Unicam Solar 969 spectrophotometer, Unicam company, England) was used to quantify K+.
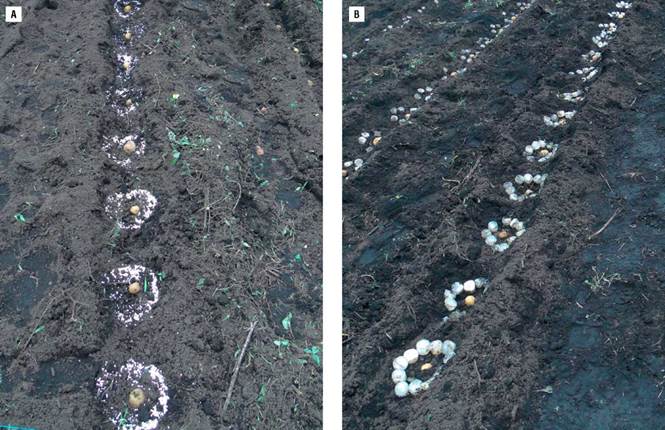
FIGURE 1 Fertilizer distribution around the potato seed. A) Treatment 1=noncoated fertilizer and B) treatment 2=CBH coated fertilizer.
Plant material samples and measured growth variables
To compare plant growth among the treatments, destructive samplings were conducted on three plants from each experimental plot fortnightly from 14 d after emergence (dae), until 8 d before harvest, which took place 126 d after planting. We quantified the total plant dry mass (PDM) (g), tuber dry mass (TDM) (g), foliar area (FA) (cm2), and chlorophyll content (SPAD units). In all cases, the result was expressed as the average of the nine sampled plants.
The PDM was the sum of the dry weight of leaves, stems, flowers, and tubers on each plant. Dry weight of plant organs was determined after dehydration of the fresh samples in an oven at 70oC until they reached constant weight. Tuber dry mass content was determined by chopping all the tubers from the same plant and drying this material in an oven at 70oC until a constant weight was reached. Leaf area was quantified using a digital camera (Canon EOS-Rebel T3) and following the methodology of Campillo et al. (2008). The relative content of chlorophyll was measured with a portable chlorophyll meter (SPAD-502 plus, Konica Minolta, Inc., Japan) on the third or fourth fully expanding leaf of the upper third in three plants per experimental plot. Three measurements per leaf were averaged.
At the time of harvest, we conducted destructive sampling to quantify tuber weight and size. Tubers from 30 different plants from each plot were classified according to the local commercial category bases on tuber diameters: > 4 cm, between 2 and 4 cm and < 2 cm. The tuber yield per plot was expressed in t ha-1. The specific gravity, a commercial quality indicator, was determined for 10 tubers (> 4 cm diameter) randomly taken from each treatment after harvest, using both the weight in water and weight in air method (Bonierbale et al., 2010).
Data analysis
The assumptions of normality were verified by the Shapiro-Wilk and Kolmogorov-Smirnov tests. The homogeneity of variance was verified by the Levene test. If any variable did not meet the assumptions, a Box-Cox transformation was performed, and an analysis of variance was performed again.
Concentrations of N-NH4 + and K+ in leachates did not meet the assumptions after transformation. Therefore, we tested if the treatment and the das explained the concentrations of N-NH4 + and K+ in the leachates using generalized linear models (GLM, R core packages Stats version 3.6.2) followed by model selection. We conducted two independent models, one for each nutrient. The response variable was the concentration (mg L-1), while the treatment and das were the fixed effects. We used a GLM from a Gaussian family, since a preliminary analysis, (Supplementary material 1 [S1]) showed our data deviated from the linear regression assumptions only slightly. To increase the robustness of our analysis, we used the corrected Akaike's information criterion (AICC) and dredge automated model selection tool (dredge, R package MuMIn; Bartón, 2020), instead of minimum squares to determine the significance of each predictor. We report the model averaged coefficients and 95% confidence intervals (considered significant if they did not overlap with 0) of the resulting models. Models with AAICC < 2 were not considered different, so the simpler model was preferred for that case. All statistical analyses were performed in R 3.6.3 (R Core Team, 2018).
Regarding plant growth and yield evaluation, a main effects analysis of variance (ANOVA) was applied to determine differences among treatments. The data from each sampling date were analyzed separately to determine the treatment effect on a phenological crop stage. Average values were compared by the Tukey's HSD post hoc analysis.
These statistical analyzes were conducted with the software STATISTICA (version 7: StatSoft Inc., Tulsa, USA), with a significance level of α=0.05.
Results and discussion
Nutrient leaching
Overall, the greatest effect of the CBH on nutrient leaching occurred from the second week after sowing (Fig. 2 A, B). The highest leachate concentrations of N-NH4 + occurred in T1 (1.05±0.51 mg L-1, mean ±standard deviation [SD]) at 15 das (Fig. 2A), a time at which the plants had not yet developed a root system to take the nutrients supplied through the fertilizer. The N-NH4 + in the leachates collected in T2 at 15 das (0.16±0.03 mg L-1), was lower than in T1 and showed similar N-NH4 + values to those in leachates from the control treatment T3 (0.12±0.005 mg L-1). Likewise, at 22 das the N-NH4 + in the leachates from T2 (0.12±0.01 mg L-1) was lower than in T1 (0.423±0.145 mg L-1), although the difference between T1 and T2 decreased. At 29 das, an important reduction in the N-NH4 + concentration was observed in leachates from T1 (0.28±0.13 mg L-1) with closer but still higher values to those observed in T2 (0.13±0.01 mg L-1) and T3 (0.10±0.002 mg L-1). After 36 d, high N values were observed in the leachates from T1, but leachates from T2 and the control remained as in the previous sampling dates. Regarding the K+ ion (Fig. 2B), its concentration in the leachates from T2 (4.18±0.75 mg L-1) was lower than in T1 (6.39±1.09 mg L-1) from 15 d.
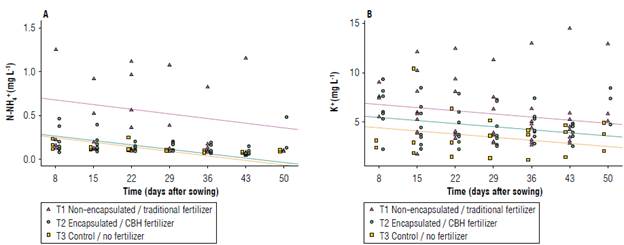
FIGURE 2 Nutrient concentration in soil leachates over time. The first seven weeks of the crop cycle in the San Isidro Farm are shown. A) Generalized linear models-GLM for N-NH4 + and B). generalized linear models-GLM for K+. Number of analyzed leachate samples per treatment 1 and 2 in each sampling date=9.
In previous studies, the mathematical models that follow the ions leaving the gel as a function of time describe a gradual release (Rozo et al., 2019). Results for AIC model selection criteria (S2) showed that only the variable treatment had an effect on the concentration of N-NH4 + and K+ in the soil. Results of the linear models are shown in Table 2. The estimate for T1 was different from the control, indicating that the application of uncoated fertilizer increased the ammonia and potassium ions in leachates. Conversely, T2 was no different from the control for any of the two nutrients, indicating that the coating reduced nutrient leaching to the same level as the control, reducing the environmental risk of using fertilizers.
TABLE 2 Results of the generalized linear models for the concentrations of the N-NH4 + and K+.
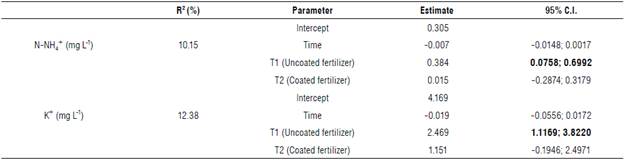
The estimates for each parameter are shown. The intercept corresponds to ion concentration in the control (no fertilizer) at the beginning of the experiment (d=0). The parameter "Time" indicates the change in concentration according to the d, which is constant for all treatments (no interactions). The percentage of variance explained is shown as the R2 in %. The effect of each treatment (coated or uncoated) was determined as the difference between their intercept and the control given by a 95% confidence interval (C.I.) around the estimate. The intervals that indicate a significant difference from the control (those that do not overlap with 0) are highlighted in bold.
It is important to highlight the large variation for N-NH4 + concentration in the leachates from plots under T1 (Fig. 2A). This may be the result of non-homogeneous fertilizer distribution in the crop soil plus the lateral flow of the water produced by the soil heterogeneity and the land slope that together may change the expected downward vertical transport of the infiltration water towards horizontal transport of the water (Hardie et al., 2012; Kim & Mohanty, 2016). Ideally, diffused nutrients from the fertilizer placed around the tuber seed would be expected to be mobilized primarily vertically into deeper layers of the soil profile by water infiltration. As a result, the fraction of the volume of the water infiltration sucked by the lysimeters placed in Tl plots would be expected to contain a high concentration of nutrients in all leachate samples. Nevertheless, the redistribution of the infiltration that resulted from the preferential water flow could avoid the infiltration water to move vertically into deeper layers of the soil profile towards the lysimeter (Bundt et al., 2001; Starr & Timlin, 2001; Zhang et al., 2018). Therefore, some lysimeters in the Tl plots probably collected infiltrated water coming from soil areas not directly influenced by the fertilizer, yielding lower contents of N-NH4 + for some of the leachate samples. On the other hand, the variation in nutrient content of leachates collected from T2 was small and similar to that of the control, indicating that plots under T2 had a homogeneous low N-NH4 + concentration across the soil surface as a result of the control that CBH exerts on the exit rate of N-NH4 + from the fertilizer.
The CBH is more efficient regulating the N-NH4 + than the K+ leaching. When the mean ion concentration in T2 is subtracted from Tl, the difference is higher for K+ than for N-NH4 +, suggesting a greater effect of the CBH on К retention. However, there was a wider range of K+ concentration values in leachates sampled in T2 (from 22 d until the last sampling date) than the one registered for N-NH4 + in T2 (Fig. 2B), pointing out that the CBH exerts less control over the diffusion of this ion from the fertilizer granule and reiterating previous laboratory results. This occurs because the presence in the hydrogel network of carboxyl and sulfate negative charges had a greater influence on the retention of the ammonium ion as a result of a greater hydration radius in NH4 + than in K+ (Guilherme et al., 2015; Xu, 2019). Anyway, less variation was observed for K+ in T2 than in Tl due to the presence of the CBH. Greater retention of potassium in the CBH could be achieved by using carrageenan with a higher content of sulfates.
Subsequent field trials with an experimental design including a greater number of replicates and plots are necessary. This is essential to measure the amount of fertilizer that can be saved using the CBH encapsulated fertilizer.
Crop growth and yield
From the beginning of tuberization detected at 42 d after emerging (dae), the PDM, FA, and TDM tended to have lower values in the plots treated with the CBH encapsulated fertilizer, but no significant differences were found among Tl and T2 for most sampling dates (Fig. 3).
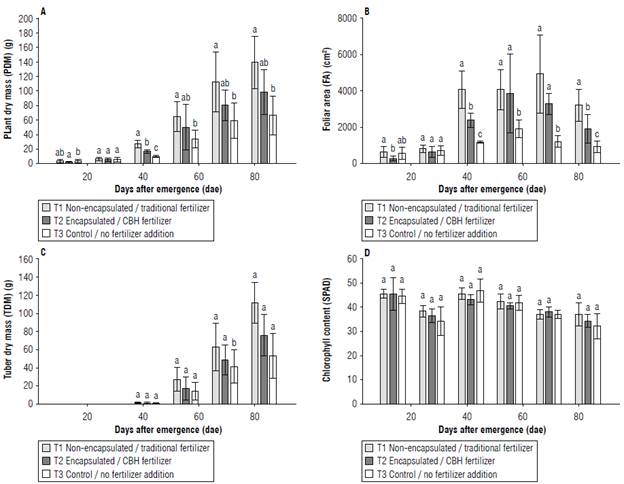
FIGURE 3 Plant dry mass A), foliar area B), tuber dry mass C) and chlorophyll content D) (mean ± standard deviation [SD]); n=9. Different letters above the bars indicate differences between treatments at P<0.05 (Tukey HSD P-values). Bars=SD.
No significant differences were observed among treatments for chlorophyll content (Fig. 3D) and specific gravity that showed an average value of 1.081 (±0.003) for Tl, 1.078 (±0.010 SD) for T2 and 1.106 (±0.025) for T3 (Fig. 4A). Potatoes in T2 had lower mean yields than T1 in all categories of tuber diameter, although there were no significant differences between treatments (Fig. 4B). The lowest yields were recorded in T3.
The lower PDM and TDM trend in plants under T2 warns of the possibility that the current CBH formulation might decrease the crop yield below that achieved by using the non-CBH encapsulated fertilizer. The CBH could be retaining nutrients around the fertilizer, harming the development of the leaf area, and diminishing the interception of photosynthetically active radiation, hence affecting the total biomass production (Allen & Scott, 1980; Santos Cas-tellanos et al., 2010; Gómez et al., 2017). Nonetheless, more field trials are needed to confirm a negative effect of the CBH on biomass production. Despite this trend towards a lower FA and PDM in T2, the chlorophyll content and the specific gravity were not affected. The chlorophyll ranged between 34.47 and 46.9 SPAD units between the 14 and 84 dae, coinciding with values previously reported by Ariza et al. (2020) for the cv. Criolla Colombia (42 SPAD at 70 das) under optimal irrigation and fertilization conditions. Therefore, in this study, normal chlorophyll levels were maintained with all treatments so nitrogen may not be a limiting factor in any of the treatments for chlorophyll synthesis. The specific gravity, an important quality indicator for the industrial processing of potato and directly correlated with the dry matter and starch content (Lulai & Orr, 1979), showed values (Tl=1.081±0.003 and T2=1.078 ± 0.01) recommended for most processed products (Kirk-man, 2007) like those reported for the cv. Criolla Colombia in previous studies (Rozo & Ñústez, 2011). The total tuber yield reported for cv. Criolla Colombia ranged between 11.15 and 20.48 t ha-1 in six localities in Antioquia department (Rodriguez et al., 2009). The tuber yield result in this experiment is within this reported range, except for ТЗ, a result that was expected due to lack of fertilizer.
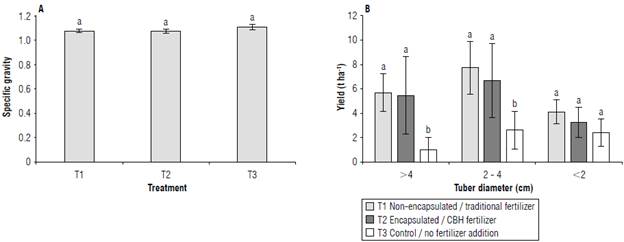
FIGURE 4 Specific gravity A) and yield B) (mean ± standard deviation [SD]); n per treatment for the yield data=3 blocks (30 plants per block) and n for the specific gravity=10 plants per treatment. The same letters above the bars indicate no differences between treatments (Tukey HSD, P>0.05). Bars=SD.
Even so, contrary to the expected, a slight trend towards a lower yield was observed when CBH is used. This is not desirable because this would translate into a lower profit at the time of harvest. It is necessary to verify whether this effect is a consequence of the CBH nutrient retention that would make it necessary to modify the hydrogel formula to ensure the right flow of nutrients at the beginning of tuber development. It might also be an effect of the field trial size and the number of samples collected for yield estimation. Therefore, new trials should include larger crops and larger samplings.
Conclusions
This study confirmed the controlling effect of the CBH on the exit of nutrients from the soil solution at the field scale and during a rainy season, ensuring the retention of the nutrients added in the fertilizer on the soil surface and preventing them from easily leaching, especially at the beginning of the productive cycle when the seed potato tubers have not emerged and do not have roots to absorb the nutrients. At the same time, it was confirmed that the CBH is less efficient controlling the exit of K+. The encapsulated CBH fertilizer allowed the same yield for crops at a field scale as the traditional fertilizers, with the additional advantage of reducing the negative environmental impact of leachates.