Introduction
The production of food without generating high levels of environmental pollution is one of the great challenges of humanity. The agricultural sector, especially livestock, contributes 18% of the total emission of greenhouse gases (GHG) into the atmosphere; at the same time, it is responsible for 37% of methane (CH4) emissions and 65% of nitrous oxide (N2O) (Steinfeld et al, 2006; FAO, 2013). Within the objectives of sustainable development of the Paris Agreement, countries have committed to reduce emissions from the different productive sectors (CMNUCC, 2017).
In Honduras, the agricultural sector has a significant influence on GHG emissions. From 1990 to 2014, synthetic fertilization (8.3%), manure applied to the soil (4%), and pastures (22.7%) were the agricultural activities that most influenced the GHG emission from soils in livestock systems (FAO, 2017). The National Climate Change Strategy of Honduras (ENCC) promotes the use of technologies, laws, training and generation of information in the industrial and agro-environmental sector; however, preventing increases in GHG emissions from the soil is complex and difficult, in a reality of low agricultural technology, extreme poverty, continuous deforestation, soil degradation, and excessive use of agrochemicals in agroecosystems (ENCC, 2022).
Grazing is the main livestock production system in the region and has been identified as a source of N2O due to deposition of animal waste in pastures, use of nitrogenous fertilizers (Pastrana et al, 2011), harvest residues, and compaction of soils, among others (Millard et al., 2004). It is estimated that between 75 and 90% of the nitrogen (N) consumed by grazing livestock is excreted through urine and manure (Luo et al, 2010). Considering that N2O has 298 greater global warming potential (IPCC, 2007) compared to CO2, its impact has importance for global warming.
The GHG emissions from the soil, especially N2O, depend on the biological cycle of N, soil management, and the particular edaphoclimatic conditions of each region (Uchida et al., 2011; Sosa, 2013). Therefore, it is appropriate to generate mitigation, soil conservation and economically competitive strategies for the producer.
Various authors have made valuable contributions to understanding the N-soil-atmosphere relationship. Silva et al. (2013) found that degraded pastures emit higher N2O compared to silvopastoral systems. Ibrahim et al. (2010) recorded that silvopastoral systems are a mitigating alternative to emission, comparable to natural forest. Bastidas et al. (2020) found that increases in synthetic nitrogen fertilization improved the agronomic performance of four pastures, including Urochloa humidicola, however, the emission of N2O increased. Organic amendments, such as biochar, generate positive effects in mitigating nitrous oxide as well as improving chemical properties of the soil (Jiang et al., 2020).
In order to increase knowledge about the dynamics of this gas and the factors that model its expression in livestock systems, this research sought to quantify the N2O flux from the soil planted with Urochloa brizantha and fertilized with bokashi and urea.
Materials and methods
Characterization of the experimental area
This study was carried out between October 2016 and April 2017 in pastures of Brachiaria of the Regional University Center of the Atlantic Coast (CURLA) of the National Autonomous University of Honduras (UNAH) located in the municipality of La Ceiba, department of Atlântida, Honduras at 15°47 '20" N and 87°51 '15" W, altitude of 26 m. The climate conditions of the experiment site were: average temperature of 26.2°C, relative air humidity of 95%, and average annual rainfall of 3,230 mm (Climatemps, 2017) distributed in the rainy season, from mid-September to February, when approximately 70% of the precipitation falls. The Oxisol (Soil Taxonomy, 2014) was analyzed (Tab. 1) at a depth of 0-20 cm before establishing the experiment.
TABLE 1 Initial physicochemical properties of the soil with pastures.
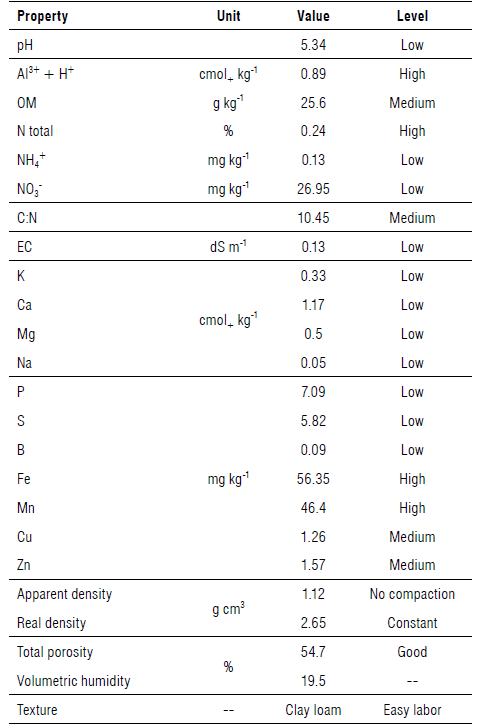
pH: aqueous solution 1:1; EC: saturated extract, conductance; Al+H: extraction with KCl; macro and microelements: plasma Mehlich-3; texture: pipette; bulk density: bevelled cylinder; real density: pycnometer; porosity and moisture: mathematical formula. The soil analysis was carried out at the Western Hemisphere Analytical Laboratory (WHAL) of the Standard Fruit company and the CURLA soil laboratory, Atlantis, Honduras, 2017.
To analyze the chemical properties of the soil, international criteria were used (Molina & Meléndez, 2002). The high levels of exchangeable acidity, Fe, Mn, low pH and the amount of organic matter (Tab. 1) should be noted, as they can interfere with the availability and absorption of mineral nutrients as well as with biological activity, and, therefore, with crop yield. The physical properties were suitable for tillage (texture), facilitating root development (apparent density and total porosity) and moisture retention.
Experiment description
The experimental units consisted of field plots with an area of 2 m2, in which Urochloa brinzantha (Hochst. ex A. Rich.) R. D. var. brizantha, established approximately 6 years ago, was planted sown at a rate of seed 2.5 kg ha-1 and fertilized with different N sources (treatments) under a randomized complete block design with four replicates (Tab. 2).
TABLE 2 Treatments established to analyze the flux of N2O from the soil planted with pastures and nitrogen fertilization.
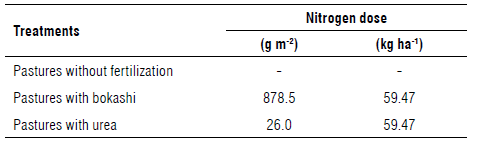
Fertilization with organic and synthetic N sources was carried out twice, at the beginning of the rainy season (November 2016) and in the dry season (April 2017). In the treatments with the chemical synthesis fertilization, this was done by applying the urea fertilizer at a dose of 26 g m-2, according to the 59.47 kg ha-1 of N required by the crop (Hernández, 2009). For the treatments with addition of organic compost bokashi type, the calculated and applied dose was 878.5 g m-2, in order to provide the same amount of N as the conventional fertilizer, according to chemical analysis performed on bokashi (total N=1.36 %, pH=7.45, C=16.75 and C:N=12.36) in the WHAL laboratory (Honduras).
The materials used to make the bokashi were 200 kg of chicken manure, 50 kg of rice husks, 200 kg of forest land, 150 kg of cow dung, 22.65 kg of charcoal, 9.06 kg of rice semolina, 0.9 kg of yeast, 1.89 L of molasses, 1.35 kg of calcium carbonate, 2.26 kg of ash, 2.26 kg of rock meal, and moisture was regulated by fist test. All the ingredients were mixed randomly and homogeneously. After 21 d, the product was harvested, turning it once every 24 h until day 14, letting it rest for the third week, monitoring its humidity and temperature regularly to ensure quality. Then it was sieved and packed in 25 or 50 kg bags.
In order to be as homogeneous as possible, the area of the base of the chamber was taken into account for the application of the appropriate amount of treatment in the quadrant; 0.234 g of the conventional treatment and 7.9 g of the organic fertilizer were applied inside the base of the chamber, and the rest outside of it.
The harvest of the pastures was done monthly, making cuts manually at an approximate height of 10 cm above the soil. In the 1 m2 area, where the chamber was established, disturbing the soil was avoided as much as possible during the samplings. In a parallel area where the chamber was established, soil samples were obtained from the 1 m2 of the soil surface at a depth of 10 cm to measure chemical properties, such as C and N contents, and physical properties. such as moisture, which affect the dynamics of nitrous oxide.
Evaluated variables
In the dry and rainy season of the year, variables related to the soil were quantified: ammonium, nitrate and total N were determined by Mehlich-3 plasma detection, and total carbon by LOI (Loss of ignition method) (Jones, 1999); volumetric moisture (Jaramillo, 2002) was determined taking compound samples made up of five subsamples from each useful plot of the treatments.
To analyze the productivity of the pasture, the pasture was harvested from 1 m2 of each experimental unit fresh 30 d after cut, at a height of 10 cm, simulating grazing. A 250 g sample was then taken and dried in an oven for 3 d at 70oC to determine the percentage of plant dry matter (DM).
For the analysis of GHG fluxes from the soil and the atmosphere, the Closed-Chamber Technique (CCT) was used (Pastrana et al., 2011; Klein and Harvey, 2012; Sosa, 2013). The CCT is a standard at the international level and is in the process of being validated for the Central American region.
The chambers were made of a PVC tube, with a diameter of 24.0 cm and 38.0 cm in height, with an acrylic cover and a circular design; in the latter, with two holes: one with a rubber septum used to introduce the thermometer and the other for sampling, without internal ventilation. The chambers were lined with an insulating membrane of aluminized polyethylene foam 9 mm thick, to avoid direct sunlight and heating of the chambers.
To install the chambers in the 12 experimental units under field conditions, the PVC ring/base was placed at a depth of 10 cm in the soil, placing it 1 d before sampling, to restore soil conditions when disturbed. The chambers were then mounted on the rings and hermetically sealed with an elastic band.
Prior to taking the samples in the treatments, the calibration curve was carried out in a range of12 h (6:00 am - 6:00 pm), with samples every 2 h for 2 d. to calculate the average gas emission and the schedule where this most often occurs.
Once the calibration was done, the sampling time was defined at 10:00 am similar to Arguedas et al. (2019) in Costa Rica. For the collection of the samples in each treatment, the internal air of the chamber was mixed by pumping the air syringes. The sample was then transferred to a 20 ml vacuum "vial" tube, to be later transported to the laboratory. Sampling lasts for 1 h, taking three samples per chamber at three different times: T0: when installing the chamber; T20 = 20 min; and T40 = 40 min. Additionally, for each day of sampling, two air samples are taken outside the chamber, at the same height of the sampling, to be used as a "blank" in the laboratory.
Sampling frequency: two months of sampling were scheduled in the rainy season, between December 2016 and January 2017, and one month in the dry season, between March and April 2017. Table 3 shows the schedule carried out according to the sampling days:
Analysis of the samples
The samples were sent to the INTA-CR Gas Chromatograph, located in Alto de Ochomogo, Cartago (Costa Rica) for subsequent study and estimation of the N2O flux and the emission factor, according to the methodology described by Venterea et al. (2012) described below:
Once the GHG concentration has been quantified (in parts per million), the N2O flux was calculated as a function of area and time. To calculate the flux of N2O, the following ideal gas equation was used:
where:
Pa=pressure in Pascal
V=chamber volume in m3
MW=molecular weight of N2O in g/mol ppm=weight of N2O at the collection T of the sample
R=Units in Pa*m3/mol*K
T=Temperature in degrees Kelvin
The linear flux was calculated using the equation:
where:
g T40 = g N2O of T40
g T20 = g N2O of T20
g T0 = g N2O of T0
Area = Chamber area, cm2
Time = Time to collect the gas sample, 0, 20 or 40 min.
Results and discussion
Accumulated flux of N2O under nitrogen fertilization
The analysis of variance recorded significant differences for the nitrous oxide flux in the treatments evaluated in the pastures (Fig. 1), differentiated by the highest emission due to chemical synthesis fertilizer, followed by bokashi and finally, by the pasture without fertilization. Soil moisture is one of the factors that modulates the emission or sink action of N2O; however, the existing variability in the soil and the contributions of rain generated conditions that masked the effect of the treatments, shown and explained later in Table 4. The contribution of N to the system soil is a key factor in the nitrification and denitrification processes, which release the highest percentage of N2O into the atmosphere. Millard et al. (2004) and Uchida et al. (2011) consider that the application of nitrogenous fertilizers significantly promotes the emissions of this gas from the soil. For their part, Harty et al. (2016) and Montenegro (2020) verify the promoting effect of urea on the emission of N2O, however, the stimulus represents 0.5 to 1% of the N applied. The entry of N into the system, regardless of the source, accelerates the mineralization of the nutrient, producing inorganic fractions that, accompanied by aeration and present humidity, generate N2O.
Dynamics of N20 emitted from the soil according to the climatic season
The emission of N20 was variable throughout the rainy season (209 mm/month) evaluated on each day sampled (Fig. 2). The trend was similar among the treatments analyzed, with the highest emission at the beginning of the month, after application of the fertilizers that correspond to each treatment. Emissions decreased in the following days, with another peak on day 17, coincident with a rainfall event of 180 mm on two consecutive days; possibly, the rainfall generated anaerobic conditions in the soil with clay loam texture.
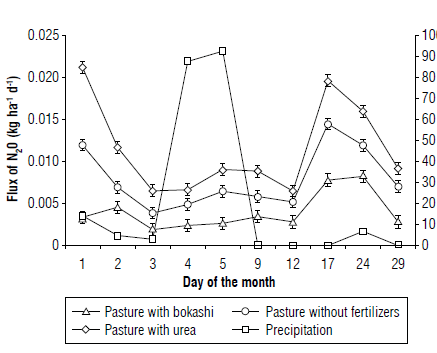
FIGURE 2 Accumulated flux of N20 In the rainy season under nitrogen fertilization on first sampling. Error bars Is standard error.
Anaerobic conditions favor the production of the analyzed GHG. Rowlings et al. (2015) and Oertel et al. (2016) consider the role played by soil moisture in gas emission to be fundamental; when the pore spaces are filled with water, values exceed 55%. In this sense, biological denitrification predominates in conditions of low oxygen concentration, allowing the action of anaerobic heterotrophic soil bacteria that use nitrates as electron acceptors instead of 02 in their respiration processes, and organic carbon as electron donor, with final products of N02, NO, N20, and N2 (McNeill & Unkovich, 2007).
After the first month of measurement of the gas emission, the pastures were cut to a height of 10 cm, simulating grazing. For this second month of measurement (Fig. 3), the incidence of rainfall was lower than the previous one, with 4.2 mm accumulated. The daily flux of N20 was low until day 9, when emission increased between treatments, coinciding with the increase in rainfall, and then gradually decreased. It is possible that the lower contribution of water to the soil affects the lower emission of N20 (Nogueira et al., 2015), at which the soil mineralization process focuses more on nitrification in dry periods, when the volumes of water are lower compared to the rainy season. A similar assessment is reported by Montenegro (2020), stating that the variations observed in N20 emissions are explained by the effect of the seasons of the year, which affect the fluctuation of water levels in soil pores. In this research, when rainfall increased, N20 emissions increased y low oxygenation conditions in the soil promote GHG production.
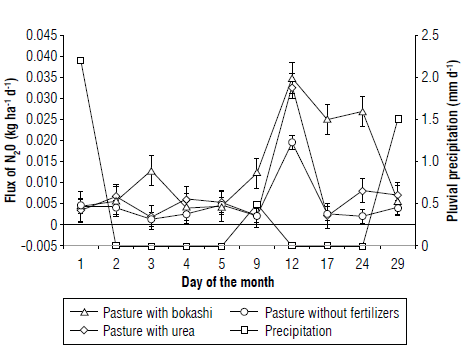
FIGURE 3 Accumulated flux of N20 In the rainy season under nitrogen fertilization on second sampling. Error bars is standard error.
For the only sampling of gas emission in the dry season (Fig. 4), very marked fluctuations were recorded between treatments. After the second fertilization before sampling, the high values seen from day 1 in the rainy season were not obtained. During the month of evaluation, with no precipitation (0 mm rainfall) recorded, nitrification predominated, supported by the supply of N in fertilization, whose respective availability of ammonium and nitrate stimulates the activity of nitrifying bacteria (Madigan et al., 2003; Sosa, 2013). A higher emission of N20 was recorded from d 4; it is possible that the lack of water limits the production of the gas. However, Mcneill and Unkovich (2007) stated that nitrifying bacteria can release ammonium and nitrates, both under aerobic and anaerobic conditions, concentrating the activity on the soil surface, which contains more humus.
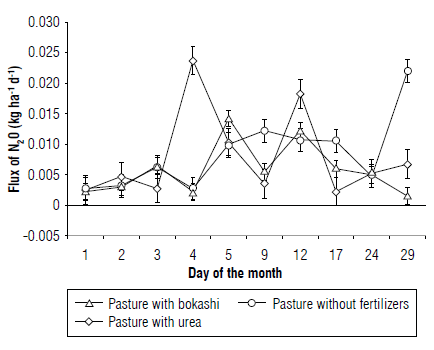
FIGURE 4 Accumulated flux of N20 In the dry season under nitrogen fertilization at first sampling. Error bars Is standard error.
Nitrogen fractions such as ammonium and nitrate, carbon, C:N ratio, soil moisture, and plant dry matter were analyzed; the ANAVA found no significant differences between treatments, blocks, and samplings (Tab. 4). There are multiple reports on variations of these edaphic parameters (Uchida et al, 2011; Nogueira et al., 2015; Rowlings et al., 2015; Montenegro, 2020), product of their interaction with the stimuli formulated and executed in studies; however, in the present research, the soil and climatic conditions masked the response of the variables analyzed.
TABLE 4 Biomass production and contents of C and N in the soil In the livestock system.
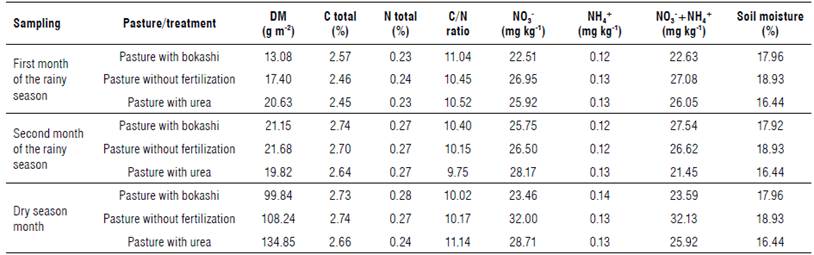
DM: plant dry matter.
It is worth mentioning that, although there was no statistical difference, the fractions of N (N03 - orNH4 +) and C regulate the emission of GHG. In this sense, the availability of inorganic N (NCV + NH4 +) from the soil in the treatments was similar throughout the study, registering a mineralization range of 0.8 to 1.35%; this process is mediated by ammonifying and nitrifying bacteria, obtaining similar values of ammonium and nitrate in the experimental units. Even so, nitrate concentrations were higher than the concentrations of ammonium, regardless of the season, indicating that nitrification prevailed, possibly generating more N20. Butterbach-Balh et al. (2013) provided a panorama of relationships between microorganisms, plant species, climate, and soil types that regulate the emission of N20.
Conclusions
Nitrogen fertilization in the pastures stimulated the emission of N20; the organic amendment, bokashi, generated less flow from the soil with respect to urea, and, therefore, a possible mitigation strategy.
The flux of nitrous oxide varied during each month of the dry and rainy seasons, with soil moisture and nitrogen availability as the modulating factors of the greatest magnitudes of the emission. The variables related to the soil (total C, total N, ammonium, nitrate and humidity) are very dynamic over time, making it impossible to show the effect of the treatments.