Introduction
Tomato (Solanum lycopersicum L.) is the vegetable crop with the highest demand and greatest economic value in the world. Tomato trade and production have particular importance in tropical, subtropical, and temperate regions of the world, for both fresh and processing markets (Gayosso-Barragán et al., 2019). Italy plays an important role in tomato cultivation worldwide, with 13% of the world's production and 53% of Europe's production (ISMEA, 2022). In Italy, as in other areas of the world, the tomato crop is undergoing increased attack by a holoparasitic plant belonging to the Orobanchaceae family, the Phelipanche ramosa (L.) Pomel (formerly classified as Orobanche ramosa L.), and commonly known as a branched broomrape. The economic damage that it causes to infested crops is worrisome.
Phelipanche seed germination occurs after a preconditioning period (moist and suitable temperatures for several days) and in response to metabolites exuded by the host plant roots (Sato et al., 2003). Following germination, this holoparasitic plant remains concealed underground for its life cycle, thus inaccessible to conventional agricultural control methods, such as tillage and contact herbicides. Following germination, the seedlings attach to the host roots by the production haustoria that penetrate the host tissues until they reach the vascular system for uptake of water, nutrients, assimilates, growing at the expense of the host plant's resources (Joel et al., 2007). Once connected to a host plant, broomrape grows rapidly, forming a tubercle (a storage organ for nutrients and water extracted from the host) underground.
Multiple shoots (up to 20) develop from the tubercle and emerge above the soil surface, then reach from 15 to 30 cm in height. After several weeks of underground development, the holoparasitic plant emerges above the soil surface, having already done most of the damage to the host, and develops flowering stems, which produce seeds within a short period of time. Most of the seeds in the soil will not germinate immediately, instead forming a seed bank for the next cropping seasons. They can remain viable in the soil for more than 10 years; thus, if host crops are frequently cultivated, the seed bank in the soil increases tremendously, leading to the failure of cultivating host crops (Fernández-Aparicio et al., 2008, 2016). The main difficulties in the control of Phelipanche seed bank arise from their immense number, diminutive size, extreme longevity, and ease of dispersal. These cause rapid increase in the parasite soil seed banks, even when the original infestation area is very limited. The amount of seed in the soil can be reduced only when the introduction of new seeds is lower than those produced that do not germinate or die naturally or by the action of pathogens or predators (Grundy et al., 2003; Van Mourik et al., 2003; Rubiales et al.,2009; Van Mouriket al., 2011). For this reason, understanding the fate of seeds in the seed bank can be an important component of overall weed control. When weed seeds enter the seed bank, several factors influence their duration. Seeds can sense the surrounding environment in the seed bank and use these stimuli to become dormant or initiate germination (Hossain & Begum, 2016).
The control of P. ramosa in the field is important not only to reduce direct damage to the host crop but also to limit the production of seeds, which will replenish the local seed bank and at the same time increase the risk of the seed dispersal to uninfested areas.
To control this parasite, there are several methods (preventive, physical, chemical, and biological) that help to avoid germination, infection, or effective reproduction of the weed (Rubiales et al., 2009; Habimana et al., 2014). For the reduction of the seed bank in the soil, various environmentally friendly approaches are possible (Disciglio et al., 2016, 2018; Lops et al., 2021), including those related to soil management and the resistant varieties.
Soil management practices can directly influence the seed environment in the soil and can, thus, be used to manage the seed longevity and germination behavior. The soil management must aim at reducing the seed bank, while minimizing the production of new seeds and their dispersal to new sites. In this regard, it is widely recognized that the primary tillage influences distribution of weed seed in the soil tillage layer (Skuodienè et al., 2013). Inversion plowing results in burial of a large proportion of seed in the tillage layer, placing them at a depth from which they cannot germinate, although they do remain viable in the deep soil for a long period of time (Roberts & Feast, 1972; Mohler & Galford, 1997; Benvenuti et al., 2001). Deep plowing has been suggested to bring seeds of parasitic weeds to a soil depth with less oxygen availability and, therefore, a reduction in their germination capacity (Van Delft et al., 2000; Eizenberg et al., 2007). Furthermore, some research findings suggest that, in newly infested fields, soil inversion or the adoption of no-till seeding may reduce the replenishment of the seed reserves in soil (Hamad et al., 2014).
Mohammed-Ahmed (1995) found that the largest number of P. ramosa attachment on roots of tomato were from the seeds buried at 3-4 cm compared to shallower -or deeper-buried seeds. Also, the experiments carried out on tomato in pots (Eizenberg et al., 2007) showed that deep-plowing delayed parasite emergence and prevented flower initiation and seed set.
The use of resistant varieties is another sustainable method to control Phelipanche (Rubiales et al., 2003; Abbes et al., 2007; Disciglio et al., 2016; Bai et al., 2020). In addition, it is a useful component of an integrated approach (Haussmann et al., 2000), because it is easy to combine with other measures such as soil fertility amendments, land preparation or soil tillage. Tomato crops are usually susceptible to the destructive Phelipanche weed, but some cultivars are potentially resistant. The selection of resistant cultivars is among the most promising approaches to deal with this problem (Bai et al., 2020; Albanova et al., 2023). In the host, response and resistance to parasitic plants are of molecular nature, which are still not completely understood (Mutuku et al., 2021). The mechanisms of resistance to parasitic plants vary depending on the host species and cultivars (Mutuku et al., 2021; Jhu & Sinha, 2022; Albanova et al., 2023). Some mechanisms underlying the resistance have been described Pérez-de-Luque et al., 2008; 2009; Pérez-de-Luque & Rubiales, 2009). Some host varieties exude stimulants of the seed germination at low levels (Labrousse et al., 2001) or excrete chemical inhibitors of germination (Serghini et al., 2001), while others exhibit strengthening and lignification of root cell walls (Jhu et al., 2022) that could reduce the holoparasitic infection. Other varieties are able to tolerate the infection with lower production losses due to lags between the development phases of the parasite compared to the productive phases of the host (Grenz & Sauerborn, 2005; Fernández-Aparicio et al., 2012) or due to the biochemical changes (low levels of amino acids in the phloem) that induce low performance of the parasite when it attacks the roots. This limits the absorption strength of the parasite and yield losses of host due to its parasitism (Abbes et al., 2009).
One underestimated aspect is the cross-resistance/tolerance to multiple stresses as well as the interaction between them (Jhu et al., 2022). In the case of tolerant varieties, their cultivation can continue to increase the seed bank of the parasite.
The number and dry weight of parasitic plants per host plant and the number of emerged shoots per surface unit can be used as indicators to monitor the Phelipanche infection (Rubiales et al., 2006). Some authors (Cubero, 1991; Rubiales et al., 2003; Abbes et al., 2011) indicate that the best indicator is the number of parasite shoots per host plant.
This study assessed two crop production practices in naturally infested fields: 1) the effect of a 50 cm soil plowing depth compared to the 30 cm depth generally used by farmers to control seed germination of P. ramosa, and 2) the resistance level of two commercially available tomato varieties to P. ramosa infection.
Materials and methods
Site description and experimental setup
The study was conducted on tomato crops in the Foggia Province (Apulia Region, Southern Italy) on heavily naturally infested soils (Typic Chromoxerert, fine, thermic, according to the Soil Taxonomy-USDA-NRCS,1999; silty-clay Vertisol of alluvial origin (1.20 m depth).
The tomato seedlings (with 4-5 leaves and a height of 100-150 mm) were obtained from a nursery and manually transplanted to the field in double rows at 0.4 m apart which were spaced at 2 m intervals, with the plants positioned 0.3 m apart within each single row. This provided a theoretical plant density of 3.3 plants/m2. Drip irrigation with the lines between each pair of plant rows was used. The water volume for each irrigation was 100-300 m3 ha-1 depending on crop growth stage, with a watering interval of 3-4 days. Weed and pest control and the other agricultural management employed were those commonly adopted by local farmers (the weed control is carried out mechanically). For fertilization at pre-transplanting, 35 kg ha-1 N and 70 kg ha-1 P2O5 were applied. Throughout the tomato crop cycles, 75 kg ha-1 N and 100 kg ha-1 P2O5 were added with fertir-rigation. Regarding pest and disease management, tomato pinworm was controlled by chlorantraniliprole 18.5% SC (Coragen®SC) at a rate of 0.3 ml L-1 (during the flowering and fruit setting stages). Fruit borers and Helicoverpa spp. were controlled by emamectin benzoate 0.5 g L-1 SC + dichlorvos 76% EC at 1 ml L-1. Early blight was controlled by spraying metalaxyl 8% + mancozeb 63%WP at 2 g L-1 of water, and late blight of tomato was controlled by spraying cymoxanil 8% + mancozeb 64% WP fungicide (Curzate M8) at 2 g L-1 (applied in the growth phase of the plants).
P. ramosa infestation was monitored as: (I) number of emerged branched holoparasitic shoots (hereafter referred to as "shoots"); (II) attachment of tubercles on tomato roots. The shoots that emerged above the soil were counted at various days after transplanting (DAT). At tomato-fruit harvest, the numbers and dry weight of mature holo-parasitic shoots and tubercles were recorded as reported by Qasem and Kasraw (1995).
The tomato fruits were harvested at full-maturity stage in August (a transplanted crop has a cycle of 100-120 d). At the tomato harvest, the marketable yield and the major qualitative parameters were evaluated as described by Disciglio et al. (2018). In particular, the following major qualitative and quantitative yield parameters were determined on 10 fruits for each plot: mean fresh weight (g), dry-matter content (% fruit fresh matter); soluble solids content (°Brix), pH, and titratable acidity (g citric acid per 100 ml fresh fruit juice) (AOAC, 1990) The color parameters were measured using a spectrophotometer (CM-700d; Minolta Camera Co. Ltd., Osaka, Japan) as the CIELAB coordinates (i.e., L*, a*, b*) on four randomly selected areas of the fruit surface. Only the a*/b* ratio has been reported, which represents an index that describes the color differences of tomato fruit (Francis & Clydesdale, 1975; Favati et al., 2009).
The monthly climatic parameters were recorded daily at the nearest meteorological stations of the Consorzio per la Bonifica della Capitanata, located at Foggia (Tavernola countryside). The site of the research was in a typical semi-arid zone, characterized by a Mediterranean climate, which is classified as an accentuated thermomediterranean climate (UNESCO/FAO, 1963), with temperatures that may fall below 0°C in the winter and exceed 40°C in the summer. Rainfall is unevenly distributed throughout the year and is mostly concentrated in the winter months, with a long-term annual average of 559 mm (Ventrella et al., 2012).
In Table 1, the main climatic data (May-September) for the two experimental seasons (2014 and 2018) are reported. Differences occurred in air temperature and rainfall between the two years of tomato cultivation. The maximum and minimum temperatures were relatively higher in 2018 than in 2014, whereas this last season was slightly drier than that of 2018 (Tab. 1).
Effect of the plowing depth
To assess the effect of two plowing depths on the infestation of P. ramosa on tomato for processing crop (cv. DRES F1 produced by Clause Vegetable Seeds Company), an experiment was performed in 2014 in the countryside of Foggia (41°65' N, 12°48' E, altitude 54 m a.s.l.) at two different fields (field A and B, about 2 km apart) of different private farms ("Ortuso" and "Pazienza" properties). The soils were silty-clay Vertisol of alluvial origin (1.20 m depth) (Typic Chromoxerert, fine, thermic, according to the USDA Soil Taxonomy (USDA-NRCS, 1999), whose physical-chemical characteristics were reported in Lops et al. (2021). In particular, the soil texture in field A was clayey-loamy, while that in field B the soil was of medium texture.
The effect of 50 cm plowing depth was compared to the 30 cm depth (control) normally used by the farmers. The two plowings were carried out in spring, just before the tomato transplant; the soil was then refined with a disc harrow. The tool used to reverse the soil at both depths was the moldboard plow.
In both fields, the tomato plants were transplanted on May 5, 2014, to the soil cultivated in the previous year with wheat. Each experiment was laid in a randomized block design, with three replications, using the plowing depth plot of 100 m2 (10 X 10 m), which included five tomato twin rows (330 plants).
P. ramosa infestation was measured by counting the emerging shoots during the tomato growing cycles, at 56, 78 and 92 DAT on three sampling areas of 4 m2 for each plot. At tomato harvest (on August 5, 2014), the numbers and dry weight of mature holoparasitic shoots and tubercles and fruit yield from 12 tomato plants (~4 m2 of sampling area where the parasite infestation was detected) were recorded.
P. ramosa flowering stems
Only in field A, at tomato harvest, a sample of 100 P. ramosa holoparasitic shoots was chosen at random in the field, the number of stem spears per branched plant was counted, and the frequency distribution of classes of the 2-amplitude interval was determined.
Effect of tomato resistance
The resistance of two commercially tomato cultivars (SV5197 and SV8840), produced by Bayer Vegetables Italia Company, to the P. ramosa infection was evaluated in 2018, in the countryside of Rignano (Foggia province, 41°40' N, 15°35' E, altitude 50 m a.s.l.).
The two varieties belonging to the pear-shaped fruit and round-shaped fruit typology, respectively, are widely grown in the agricultural areas where the experiment was carried out.
The transplant of the tomato cultivars was carried out on May 10, 2018. The experiment was arranged according to a completely randomized design, with three replicates, using the tomato cultivars treatments as plots of 60 m2 (6 X 10 m); each plot included three tomato twin rows (200 plants). The P. ramosa infestation was measured by counting the emerging shoots during the tomato growth cycle at 45, 76, and 96 (harvest) DAT on three sampling areas of 4 m2 (along the central row) for each plot. Moreover, at tomato harvest on August 14, 2018, the final number of parasites per host plant was counted. Dry weight of holoparasitic shoots and tubercles was also recorded. The dry weight was determined by drying samples in a ventilated stove at 70°C for about 12 h.
Statistical analysis
All data were submitted to ANOVA by using the GLM Procedure of SAS software, version 6 (SAS Institute, 1999). When the main effects were significant, differences between means were compared with the Tukey's test at P<0.05. Standard deviations (SD) were calculated using Excel software of the Office 2007® suite. Percentage values of fruit dry matter were transformed to arcsine prior to analysis of variance.
Results and discussion
Plowing depth
P. ramosa infection and density
Figure 1 shows the average number of emerged shoots per m2 at 56, 78, and 92 DAT of 30 cm (control) and 50 cm plowing depth treatments in both fields.
At 56 DAT, the number of emerged shoots was low in both fields with no significant difference between plowing depth treatments. At 78 and 92 DAT, the infestation increased, albeit with some variations between the two fields, particularly at 78 DAT, with a significantly higher number of emerged shoots at 30 cm plowing depth than at 50 cm. From 78 up to 92 DAT, the shoot density slightly increased for the 30 cm plowing depth in both fields, while, for the 50 cm plowing depth in field B, the infestation continued to increase. Significant higher emerged shoots were measured at the tomato harvest (92 DAT) in 30 cm plowing in both fields A and В (34.8 and 44.7 m2, respectively) compared to the 50 cm plowing (18.2 and 27.8 rrf2, respectively).
In Table 2, the average number of P. ramosa emerged shoots at different days after transplant of the two fields are reported.
TABLE 2 Average shoot density (plants/m2) of P ramosa for each 30 and 50 cm for each plowing depth and for each field at different days after transplant (DAT).

Data are means±SD,n=9. Different letters on cumulative values of each DAT and in each column of each field and treatment indicate significant differences atP≤0.05 according to the Tukey's test.
In both field trials, in accordance with what has been described for the emerging shoots, the mean values of total attachments, dry weights of sprouts and tubercles were significantly higher in the 30 cm plowing than in the 50 cm plowing (Tab. 3). Some differences were noted between the two fields. In field A, the number of total attachments, dry weight of shoots, and dry weight of tubercles for deeper plowing (50 cm) respect to shallower plowing (30 cm) were lower (-52.6%, -52.5%, and -52.9%, respectively) than those in field В (-61.7%, -63.3%, and -62.1%, respectively). This maybe due to differences in the level of Phelipanche infestation and/or of soil characteristics between the two fields.
TABLE 3 Mean of total attachments per m2, dry weight of shoots, and dry weight of tubercles (g) per tomato plant for 30 and 50 cm plowing depths In each field, for each plowing depth and for each field.
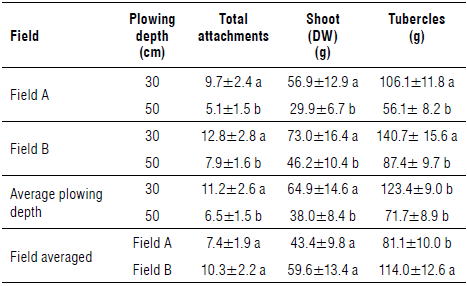
Data are means ±SD, n=30. Different letters in each column of each field and plowing treatment indicate significant differences at P≤0.05 according to the Tukey's test.
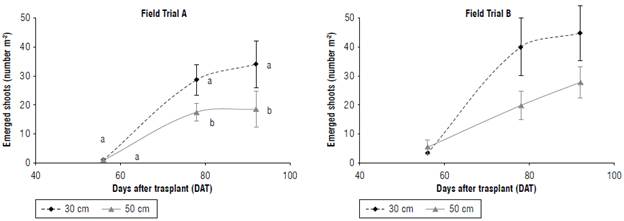
FIGURE 1 Shoot density (plants/m2) of P. ramosa for 30 and 50 cm plowing depth at different days after transplant. Values are means±SD, n=3. Different lowercase letters Indicate significant differences between plowing depth treatments in each day at P≤0.05 according to the Tukey's test.
As an overall average between fields of each plowing depth (Tab. 3), the number of total attachments, dry weight of shoots, and dry weight of tubercles were lower in the 50 cm plowing than the 30 cm plowing by 58.0%, 58.5%, and 58.1%, respectively. Moreover, as an overall average between plowing depths of each field, the same parameters in field В showed an increase compared to field A of 39.2%, 37.2%, and 40.6%, respectively. Although these P. ramosa reductions are to be considered positive, they are lower than those reported on tobacco by Habimana et al. (2014) (80-90%), using the same 50 cm deep plowing. Our results are in accordance with those of Eizenberg et al. (2007) in which deep inversion plowing (to more than 30 cm) would bury broomrape seeds to a depth below the soil layer where attachment to tomato root can occur.
P. ramosa flowering stems
Multiple shoots develop from the tubercle and emerge above the soil surface, then grow into stems from 15 to 30 cm in height. Figure 2 shows the frequency class distribution in the range of 2 amplitudes of emerging flowering stems per P. ramosa branched plant. The number of flowering stems ranged from 1 to 20, but most ranged from 1 and 10. The highest percentage (32.3%) was shown by 6 stems, followed in order by 4 (20.3%), 8 (16.9%), 2 (14.9%) and 10 stems (6.2%).
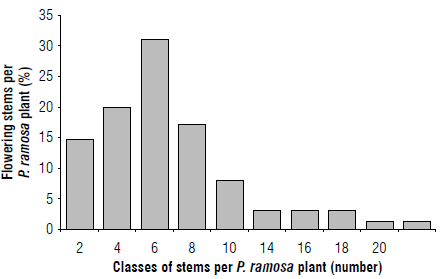
FIGURE 2 The frequency distribution for classes of 2-amplitude interval of flowering stems per P. ramosa emerged shoots.
Tomato fruit yield and qualitative traits
Results of the tomato productive traits for the two compared plowing depths are reported in Table 4. In both fields A and B, the 50 cm plowing depth provided significantly higher marketable yield (66.9 and 93.11 ha4, respectively) than the 30 cm plowing depth (55.5 and 74.3 t ha4, respectively). No significant differences were found for the qualitative characteristics of the fruits between plowing depths and fields.
In Figure 3, the negative correlations between marketable yield and P. ramosa emerged shoots and of both fields A (R 2 = 0.83) and В (R 2 = 0.94) are shown.
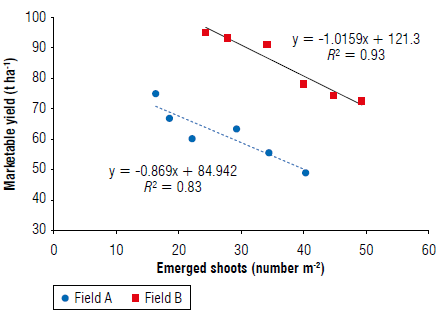
FIGURE 3 Linear regression between tomato marketable yield and number of emerged shoots of P. ramosa detected at tomato harvest in each field (data used in the analysis refer to the single plot of each field).
In general, the tomato marketable yield and P. ramosa infestation were related; the smaller numbers of emerged shoots resulted in increased marketable yield. This result agrees with previous research performed on tomato (Conversa et al., 2017; Disciglio et al., 2018). The regression lines show almost similar slopes in both fields A and В (-0.87 and -1.01, respectively), thus, highlighting a similar depressive effect due to the variation of the infestation.
Tomato resistance to P. ramosa
P. ramosa infection and density
In this experiment, the level of resistance of two tomato cultivars to P. ramosa was different. According to Table 5, P. ramosa infestation was detected at 45, 76, and 96 DAT. On all sampling dates, significant higher emerged shoots in the cv. SV 5197 than cv. SV8840 were observed. At 96 DAT, a 65.1% reduction of emerging shoots in cv. SV8840 compared to cv. SV 5197 was measured.
TABLE 5 Mean values of P. ramosa emerged branched shoots (plants/ m2) in each tomato variety at different days after transplant (DAT).
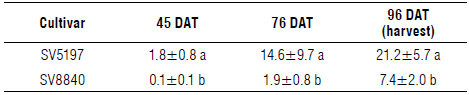
Data are means±SD, n=9. Different letters on cumulative values of each DAT and in each column indicate significant differences at P≤0.05 according to the Tukey's test.
Table 6 reports the number of P. ramosa total attachment on tomato roots and the dry weight of emerged and underground branched shoots per host plant, detected at the end of the experiment. Differences in responses of tomato cultivars to P. ramosa infection were observed for these measured parameters. The results, in accordance with what has been described for the emerging shoots, showed that, for the three measured parameters, higher values were obtained for the SV5197 cultivar than for SV8840 cultivar, which could be correlated with the different level of resistance of the host plant. Differences in susceptibility to infection were also seen among tomato varieties based on their difference in the number of emerged shoots of Phelipanche and Phelipanche plant dry weight (shoots and tubercles) (Tokasi et al., 2014). The SV8840 cultivar could exude stimulants at a lower level and, therefore, could be suitable for reducing Phelipanche infection. These results agree with previous studies by Kasrawi and Abu-Irmaileh (1989), Qasem and Kasrawi (1995), and Mariam and Su-wanketnikom (2004).
TABLE 6 Total attachments, dry weights of emerged shoots and tubercles of Phelipanche ramosa per tomato host plant of the two varieties.
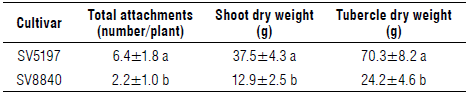
Data are means±SD, n=30. Different letters in each column indicate significant differences at P≤0.05 according to the Tukey's test.
Tomato productivity
Results of the tomato productive traits for the two compared tomato cultivars are reported in Table 7. The SV8840 cultivar, which showed the lowest P. ramosa infection, had significantly higher yield than the SV5197 cultivar. This could be due to the low infestation, as seen previously, although other factors dependent on the two genotypes used in the experiment may have been involved. In this last respect, higher mean weight and width, and lower length were shown from the SV8840 round-shaped fruit compared to the SV5197 pear-shaped ones. Finally, fruit characteristics were not significantly different between the tested cultivars.
Conclusions
This study addressed the agronomic strategies of depth of plowing of the soil and use of resistant varieties to reduce the infestation by P. ramosa in processing tomato cultivation. Inversion plowing or deep burial of weed seed using a moldboard plow is a very effective method of decreasing seedling emergence. This is consistent with the results of many ecological studies which showed that weed seedling emergence is inversely related to the depth of seed burial and that maximum emergence is from shallow depths of around 1 cm for most species. Small-seeded weeds can only emerge from shallow depths while large-seeded ones can germinate from greater depths if conditions are suitable, but rarely do they emerge from depths of15 cm or more. In our environments, plowing is usually carried out in autumn or spring. In the latter period, it is performed just before sowing, with the main objective of avoiding hardening of the clods, caused by the gradual appearance of the sun and from the sun heat.
Based on our data obtained from two experiments in natural infested fields on clay-loamy and medium-texture soils, for the same tomato cultivar (DRES F1), the 50 cm plowing depth overall reduced infestation by about 41% compared to the plowing depth of 30 cm, commonly adopted by farmers in Italy. However, this reduction was slightly lower (- 52.7%) in clay-loamy soil than in medium texture one (- 62.4%). Multiple shoots develop from the tubercle and emerge above the soil surface. Most of them (70%) ranged from 4 to 8.
Moreover, in another experiment, different infection levels by P. ramosa were detected between two commercial tomato varieties (SV5197 and SV8840) . The SV8840 results in a tolerant variety capable of enduring infection.
In both experiments, the largest number of Peliphanche emerged shoots provided a significantly reduced market yield, while no significant influence was found on the qualitative characteristics of the fruits.
Therefore, the results obtained in this study, carried out under infested field conditions, show that agronomic strategies, such as soil plowing depth and resistant tomato cultivars are suitable to reduce P. ramosa infestation. For the continuing reduction of parasite seed bank in the soil, the utilization of both the aforementioned methods could be even more efficient if they are used jointly.