Introduction
Starch is a homopolymer composed of units of α-D-glucopyranose that can be linked by α-D-(1,4) and α-D-(1,6) glycosidic bonds to form two different polymeric structures: amylose and amylopectin. Amylose and amylopectin are densely packed in a semi-crystalline state that affects the susceptibility of starch to enzymatic attack (O'Brien & Wang, 2008). Compared to cereal starches, for example, tuberous root granules have a smoother surface free of porosities (Chen et al., 2011). Cassava starch has been widely incorporated in the formulation of food matrices as a stabilizer, texture modifier, fat emulator, or encapsulating material for bioactive compounds (Jayakody & Hoover, 2008). To improve the properties of thermo- or photosensitive substances as carriers of micronutrients or encapsulating materials, their adsorption properties should be enhanced through modification that promotes the development of structures with excellent micro- or mesoporous characteristics.
There are many methods to produce porous starches that involve physical, chemical, and enzymatic treatments, or a combination of them. Enzymatic modification is characterized by polymer degradation, total or partial loss of the native morphology, and molecular disorganization, altering the physicochemical properties of the granules (Rocha et al., 2010; Figueroa-Flórez et al., 2019). These biocatalytic processes offer several advantages: they are efficient, safe, and ecologically sustainable. In addition, the enzymatic modification involves the use of polypeptides in the production of porous starches, whose granules have lacerations, cracks, or pores that run from the surface to the interior (Xie et al., 2019) and are used in the industry for carrying or encapsulating pigments, antioxidants, fatty acids, etc. (Gao et al., 2013; Dura et al., 2014; Xie et al., 2019).
Several studies have reported the use of endo- or exo-amylases from different botanical sources in the production of porous starches (O'Brien & Wang, 2008; Wu et al., 2011; Zhang et al., 2012; Gao et al., 2013; Dura et al., 2014; Wang et al., 2016; Benavent-Gil & Rosell, 2017; Jung et al., 2017; Xie et al., 2019). Although few articles in the literature have examined the biocatalytic action of amylolytic enzymes in the production of porous cassava starches using α-amylase or amyloglucosidase, the articles have concluded that tuberous root starches are less susceptible to enzymatic attacks because they show small superficial lacerations and shallow holes (Rocha et al., 2010; Chen et al., 2011; Benavent-Gil & Rosell, 2017; Figueroa-Flórez et al., 2019).
Other authors have proposed the use of physical treatments in the production of porous corn starches to increase the size and volume of the pores and improve the adsorption capacity of the starches. Zhao et al. (2018) explored a freezing-cooling combination and obtained significant changes in the crystallinity index, thermal, and morphological properties, as well as in the water adsorption capacity and solubility of corn granules. Thermal processes such as annealing have been implemented to optimize enzymatic hydrolysis; as a result, significant changes in structural properties and granules with a microporous morphology have been reported (Tukomane et al., 2007; O'Brien & Wang, 2008; Shariffa et al., 2017; Xie et al., 2019).
Previous studies have identified the presence of lacerations or small porosities in enzymatically modified cassava granules (Shariffa et al., 2009; Chen et al., 2011; Dura et al., 2014). However, few authors have evaluated the effect of physical pretreatments on the enzymatic hydrolysis to develop porous cassava starches. We hypothesize that physical-thermal pre-treatments alter the native semicrystalline packing that favors the enzymatic absorption phenomenon, endo-corrosion processes, and the formation of micro-porous granules. The literature does not include reports on the characteristics of the pores in cassava-modified starches in terms of type, size, and pore volume. The aim of this study was to evaluate the effect of physical and thermal pretreatments on the degree of hydrolysis and the structural and morphological properties of native cassava starches during enzymatic modification.
Materials and methods
Materials
Native cassava starch (Manihot esculenta cv. M-Tai) was supplied by Almidones de Sucre S.A (Induyuca®, Sincelejo, Colombia). Amyloglucosidase from Aspergillus niger (Dextrozyme® GA, Novozymes, Denmark) and α-amylase from Bacillus licheniformis (Liquozyme® Supra 2.2X, Novozymes, Denmark) were used in the experiment. Analytical grade potato amylose (AO512, Sigma Aldrich, Germany) was also used.
Physical and thermal pretreatments
Native cassava starch (NCS) with a moisture content of ~50% (dry weight basis), was subjected to the following pretreatments:
Annealing (ANN): Heating for 60 min at 60°C under constant agitation at 250 rpm in a thermostatic bath (MaxQ® 4450, Thermo Scientific, USA);
Homogenization (HMG): Pretreatment using an ultraturrax disperser (T25 Basic®, IKA, Germany) under stirring at 3000 rpm for 60 min at 60°C;
Ultrasound (UTS): Process performed in an ultrasonic cleaner (Model CPX3800H, Branson, USA) at a frequency of 40 kHz and 60°C for 60 min;
Annealing and ultra-rapid freezing (ANN-C): Heating at 60°C with shaking for 240 min with subsequent storage in an ultra-low temperature at -12°C for 8 h (Kaltis®, F390, USA). Pretreated starch was recovered and dried in a forced convection oven (UFB500, Memmert, Germany) at 35°C for 8 h.
Enzymatic hydrolysis of starch
Pretreated-starch suspensions at 20% (w/v) were subjected to enzymatic hydrolysis with the simultaneous application of α-amylase (15 U/g starch) and amyloglucosidase (10 U/g starch) in a sodium citrate/citric acid buffer solution at pH 5.0, 60°C and 250 rpm for 6 h (Figueroa-Flórez et al., 2019). Subsequently, hydrolysates were removed by centrifuga-tion at 5000 rpm for 15 min. Samples were washed with ethanol and distilled water to remove the residual enzyme content. Finally, the starch was recovered and dried in a forced convection oven at 35°C for 8 h.
Native cassava starch (NCS) and non-physically pre-treated starch granules (NPS) were referred to as control treatments. The NPS treatment corresponded to samples of enzymatically modified starch without physical and thermal pretreatment.
Hydrolysis degree (HD)
The degree of hydrolysis was evaluated from the reaction of reducing sugars released with 3,5-dinitrosalicylic acid (DNS) by UV-Vis spectrophotometry (UV-2550, Shimadzu, Japan) at a wavelength of 540 nm (Salcedo-Mendoza et al., 2018). We used 500 μl of sample to react with an excess amount of DNS for 8 min at 80°C with subsequent cooling to 10°C to stop the reaction. The degree of hydrolysis was expressed as the ratio between weight at the beginning and at the end of the period of enzymatic action (Figueroa-Flórez et al., 2019). The concentration of sugars was expressed in glucose equivalents (GE).
Starch morphology
Starch granules were fixed in a sample holder with electrically conductive carbon tape covered with a platinum/ gold alloy (Chen et al., 2011). Samples were observed on a scanning electron microscope (JEOL, JSU LV-5600, Japan) under conditions set at 15 KV, 30 mA and an amplitude margin 3000X.
Determination of granular size
Granular size was determined by light scattering using a Mastersizer particle analyzer (Model 3000E, Malvern, UK) and expressed as a function of the average diameter DX (50). Measurements were performed in triplicate at room temperature with a refractive index of 1.52 (Monroy et al., 2018). Pore characteristics were determined using an automatic specific surface area and porosity analyzer (Quantachrome Inc., NOVA 2000e, USA). Pure nitrogen (> 99.99%) was used to determine adsorption-desorption isotherms. The starch samples were dried for 20 h under vacuum at 100°C, degassed at 125°C for 24 h, and immersed in liquid nitrogen (-196°C) in the range of relative pressure P/Po « 5-19% (Gao et al., 2013). The mean pore diameter and the specific surface area (AS) were calculated with the BET method (Brunauer-Emmett-Teller). The total pore volume (v) was estimated with the BJH (Barrett-Joyner-Halenda) method (Guo et al., 2020).
Amylose content and infrared spectroscopy (FTIR)
Amylose content was estimated by the colorimetric iodine method using a UV-Vis spectrophotometer (UV-2550, Shimadzu, Japan) at a wavelength of 620 nm (Salcedo-Mendoza et al., 2018). Infrared spectra were obtained in the region of 500 to 4000 cm-1 making 32 readings at a resolution of 4 cm-1 using a spectrometer (Nicolet IS50 FT-IR, Thermo Scientific, USA). The order degree (OD) was estimated as the absorbance ratio in the 1047/1022 cm-1 bands, expressed as a percentage (Ma et al., 2018).
XRD diffraction patterns
Diffraction patterns were obtained with an XRD diffrac-tometer (X'Pert Pro-MPD, Panalytical, Italy) in the range of 4-35°, operating at 1.8 kW with a current of 40 mA (Salcedo-Mendoza et al., 2018). The crystallinity index (CI) was calculated by the ratio of areas of the absorption peaks (crystalline zone) over the total area using Origin Lab v8.0 (OriginLab Corporation, USA).
Water solubility index (WSI), water absorption index (WAI), and swelling power (SP)
WSI, WAI, and SP were determined with slight modifications according to Rocha et al. (2010). One g of starch was dispersed in 25 ml of distilled water under agitation at 60°C for 30 min, then centrifugation was performed at 5000 rpm for 15 min. The pellet was removed, and weight was quantified. The SP was estimated as the ratio between the gel weight and the initial weight of dry starch, using the equation reported by Figueroa-Flórez et al. (2019). The resulting gel was weighed to estimate WAI. An aliquot of 10 ml was taken from the supernatant, poured into Petri dishes, and evaporated in an oven at 70°C for 16 h. WSI was calculated as the amount of dry solids recovered by evaporating the water absorption test supernatant.
Thermal properties
Gelatinization properties were determined using a differential scanning calorimeter (DSC-Q2000, TA Instruments, USA) and a suspension in an aluminum pan with a 2 mg of starch and 6 mg of water ratio (Xie et al., 2019). Capsules were sealed and stored at 25°C for 24 h to balance the system. Subsequently, they were heated from 20°C to 120°C at a speed of 10°C min-1 and cooled to a ramp of 25°C min-1 under a nitrogen atmosphere. Onset temperature (TO), peak temperature (TP), and conclusion temperature (TC) were determined from the DSC curves. The gelatinization enthalpy (AH) was estimated based on the endothermic peak area expressed in J/g.
Experimental design
A unifactorial categorical design was implemented where each level is related to a specific pretreatment. Results were analyzed using statistical tools such as analysis of variance (ANOVA) and Tukey test for comparison of means with a level of significance of 5% using the Statgraphics software (Centurion XVI, Statgraphics Technologies Inc., USA).
Results and discussion
Effect of pretreatments on the enzymatic hydrolysis of starch granules
The hydrolysis degree oscillated between 11.99 ± 0.65% and 24.92 ± 0.40% (Tab. 1). Similar results have been reported during hydrolysis with α-amylase and/or amyloglucosidase in starches from various amylaceous sources (Rocha et al., 2010; Shariffa et al., 2017). This behavior is probably due to the degradation of amylose and amylopectin polymer fractions by the synergistic action of amylolitic enzymes, affecting structural and morphological characteristics of the granules (Chen et al., 2011).
A decrease in the degree of hydrolysis was found in the UTS treatment (21.40 ± 0.82%) with respect to the NPS treatment (11.99 ± 0.65%). These results were lower than those reported in the hydrolysis of corn starch through the application of ultrasonic technology (Li et al., 2018). However, treatment of UTS can alter swelling and solubility properties, changing the interaction of polymer chains with water molecules and the semicrystalline order of the granules, and forming granules less susceptible to enzymatic attack (Wu et al., 2011). This same behavior associated with the redistribution or recrystallization of amylose and amylopectin chains during ANN-C treatment could explain the molecular resistance of the granules to enzymatic degradation and the decrease in the degree of hydrolysis (16.71 ± 0.60%).
The results of the degree of hydrolysis correlate with morphological changes of granules. In Figure 1, NCS granules with oval or spherical morphologies of relatively smooth surfaces without lacerations can be seen, with truncated ends possibly inherent to the extraction process (Salcedo-Mendoza et al., 2018). But, after the enzymatic treatment, granules showed erosion on their external surface and generalized cavities with apparent depth (Fig. 1NPS), as well as a rupture or fragmentation, probably associated with the physical and thermal pretreatments established before the biocatalytic process. Similar results have been reported previously, where the same pretreatments facilitated the release of starch components from the amorphous zone affecting the morphological characteristics of the granule on a superficial level (Chen et al., 2011; Wu et al., 2011; Shariffa et al., 2017; Zhao et al., 2018; Wang et al., 2022).
The microphotographs of the starches pretreated with UTS (Fig. 1UTS) suggest a granular surface and microstructural modifications. Likewise, the starches modified with the ANN treatment showed more generalized superficial granular lacerations with respect to NPS samples (Fig.1NPS), in line with the results reported for hydrolyzed cassava and sweet potato starches pretreated under gelatinization temperature for 72 h (Shariffa et al., 2017). With the HMG pretreatment there were deep surface erosions, presumably due to the enzymatic action (Fig. 1HMG) and a generalized loss of granule integrity due to exposure to shear stress. These data are consistent with those reported for mechanically modified cassava starches (He et al., 2014). After the ultrasonic pretreatment noticeable changes were observed in granular morphology that presented greater cracks and superficial lacerations at a lower degree of hydrolysis with respect to its NPS counterpart.
TABLE 1 Hydrolysis degree, amylose content, and physicochemical properties in native and modified cassava starches.
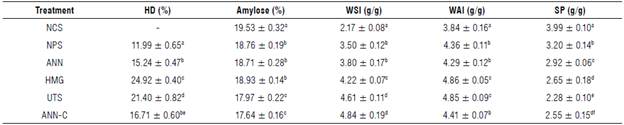
HD - hydrolysis degree; WSI - water solubility index; WAI - water absorption index; SP - swelling power. Native cassava starch (NCS); non-pretreated enzymatic modified starch (NPS); modified starch pretreated by annealing (ANN); modified starch pretreated by homogenization (HMG); modified starch pretreated by ultrasound (UTS), and modified starch pretreated by annealing and ultra-rapid freezing (ANN-C). Different superscripted letters in the same column indicate significant differences for Tukey test (P<0.05).
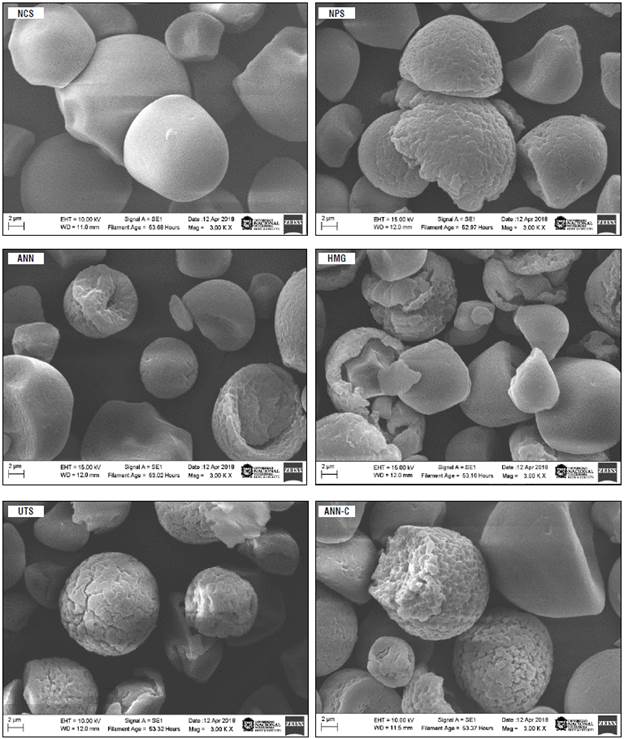
FIGURE 1 Microphotographs of native and modified cassava starches (3000x). Native cassava starch (NCS); non-pretreated enzymatic modified starch (NPS); modified starch pretreated by annealing (ANN); modified starch pretreated by homogenization (HMG); modified starch pretreated by ultrasound (UTS); modified starch pretreated by annealing and ultra-rapid freezing (ANN-C).
Similar observations have been reported regarding corn starches modified using various ultrasound wave frequencies (Li et al, 2018). With the ANN-C treatment, an extended and uniform porosity was observed in the granules, accompanied by more pronounced lacerations or cracks in their surfaces (Fig. 1ANN-C). Experiments conducted with sweet potato and waxy rice starches have revealed an analogous behavior after applications of annealing or successive freezing (Tonon et al., 2009; Tao et al., 2015).
The annealing treatment produces cracks in the granules of sweet potato starch that exhibit a greater proportion of porous granules that preserve the integrity of their morphology (Tonon et al., 2009). Tao et al. (2015) consider that the presence of some fragmented granules is due to the powerful compression force exerted by the formation of ice crystals during freezing. Therefore, a greater generation of pores and lacerations is a consequence of thermal treatments that increase the surface area and favor the enzymatic action on polyglucan chains (Zhao et al., 2018).
The granule size had a bimodal distribution between 5 and 100 [im for NCS, and the mean diameter Dx (50) ranged from 17.05 to 21.65 [im for all samples (Fig. 2, Tab. 2). The diameters Dx(50) decreased after the enzymatic treatment in the following order: HMG<UTS<ANN-C<ANN<NPS. The samples of modified starch pretreated with HMG exhibited the smallest diameters (17.05 ± 0.35 цт), possibly caused by shear force action (Hossen et al., 2011). These results are consistent with the morphological changes of the granules analyzed by microscopy (Fig. 1HMG).
TABLE 2 Physical characteristics of starch granule populations in samples.
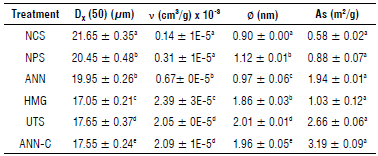
Dx (50) - average diameter of microporous granule; v - total volume of microporous granule; ∅ - average diameter of microporous granule; As - specific surface area. Native cassava starch (NCS); non-pretreated enzymatic modified starch (NPS); modified starch pretreated by annealing (ANN); modified starch pretreated by homogenization (HMG); modified starch pretreated by ultrasound (UTS), and modified starch pretreated by annealing and ultra-rapid freezing (ANN-C). Different superscripted letters in the same column indicate significant differences for Tukey test (P<0.05).
UTS and ANN-C exhibited significantly reduced diameter Dx (50) in NCS. Ultrasonic cavitation and molecular reorganization (caused by ice crystals during freezing), probably, triggered structural changes (Wang et al., 2022) that favored hydrolysis, with morphological changes in granular size, pore size, and volume increase (Tab. 2). The ANN treatment only caused a slight reduction in the diameter Dx(50) with respect to its NPS counterpart. These results confirm that physical and thermal treatments reduce granular size and increase the porosity of the granules based on the depolymerization of the unstable amorphous materials present in the granular surface and the endogenous action of ct-amylase hydrolyzing from the surface to the hilum (Tukomane et al., 2007; Monroy et al., 2018; Zhao étal, 2018).
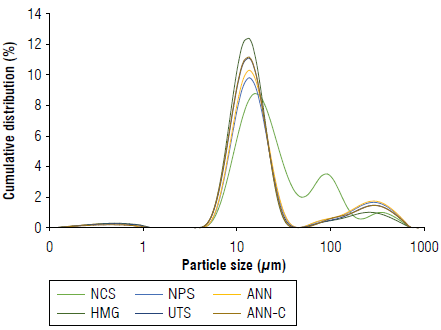
FIGURE 2 Particle size distribution of native and modified cassava starches. Native cassava starch (NCS); non-pretreated enzymatic modified starch (NPS); modified starch pretreated by annealing (ANN); modified starch pretreated by homogenization (HMG); modified starch pretreated by ultrasound (UTS), and modified starch pretreated by annealing and ultra-rapid freezing (ANN-C).
Modification processes caused a significant increase in specific surface area, volume, and pore diameter (P<0.05) (Tab. 2). The N2 adsorption isotherms of the modified starches were type IV with a hysteresis curve type H3 (results not shown) that is characteristic of mesoporous materials (Rouquerol et al, 1994). In this study, pore size was greater than 2 nm. Therefore, according to the classification presented by Xie et al. (2019), the material was mesoporous. The mesopores identified and estimated in NCS samples were probably caused by the presence of intrinsic porosities of the granule in its native state or the action of endogenous enzymes during the extraction process (Foresti et al, 2014). The results with the NCS treatment showed that enzymatic action increased the surface area, diameter, and volume of the pores compared to native starch (Tab. 2). However, no significant increase in mesoporous properties was detected with the HMG treatment, possibly because the mechanical action during the size reduction facilitated the amyloglucosidase endo-corrosion phenomenon and led to a higher production of fragmented granules. Specifically, the granules pretreated with UTS and ANN-C exhibited more uniform porosity and larger pores that did not affect their native spherical or oval shape, as shown in the SEM microphotographs. Sharrifa et al. (2009) consider that temperatures close to gelatinization can swell the granule during pregelatinization, increasing the opening and size of the pores that could facilitate enzyme absorption and degrade the external part of the granule by exo-corrosion. As pore size grows due to exo-corrosion, it may also favor the phenomenon of endo-erosion toward the inside of the granule (Keeratiburana et al., 2020). All the enzymatic actions occurred at the surface or internal level, leading to the production of mesoporous granules with better hydrophilic properties.
The enzymatic process significantly altered the interaction of polymer chains with water molecules (Zhong et al., 2022). There was an increase in modified starches WSI and WAI with respect to the NCS samples (Tab. 1), possibly due to the breakdown of intermolecular bonds and the depolymerization of long-length chains, helping the release and availability of soluble polymer components during granules swelling process (Wang et al., 2016; Jung et al., 2017). The WSI values with respect to the control increased in the following order: HMG>UTS>ANN-C>ANN>NPS, while WAI values increased in the order: HMG>UTS>ANN-C>NPS>ANN. The increase of WSI and WAI in modified starches pretreated by HMG and UTS, possibly depends on granule size reduction by the HMG process or the increase of surface porosity, since those effects increase the surface area improving the interaction and penetration of water molecules, and other authors (Gao et al., 2013; He et al., 2014; Benavent-Gil & Rosell, 2017) report an increase in water solubility and absorption properties in corn starches subjected to a size reduction process. However, this contrasts with a decrease in WSI of modified starches that underwent annealing before the hydrolytic process (Gomes et al., 2005). The decrease in solubility may be due to molecular reorganization between amylose and/or amylopectin helices during annealing, producing a more stable structure that prevents the leaching of soluble components of the granules (Dias et al., 2010; Shariffa et al., 2017).
The SP decreased after enzymatic hydrolysis (Tab. 1), and similar results were reported in the production of porous corn starches with amyloglucosidase and glycosyltransferases (Dura et al., 2014; Benavent-Gil & Rosell, 2017). The decrease in SP has been attributed to the increase in crystal perfection, the intermolecular binding forces, and the decrease in WSI of polymer chains (Waduge et al., 2006; Jayakody & Hoover, 2008; Shariffa et al., 2017). The foregoing could explain the behavior in SP reduction in starches pretreated by UTS and ANN-C that showed higher relative crystallinity index and lower susceptibility to the enzymatic attack, as a consequence of strengthening between the amylose-amylose and amylose-amylopectin interactions. In relation to the pretreatment, SP values decreased in the following order: UTS<ANN-C<HMG<ANN<NPS. Several studies have reported the reduction of SP after annealing in starches from different starch sources associated with changes in amylose content and reordering of amylopectin double helices during cooling (Gomes et al., 2005; Waduge et al., 2006; Jayakody & Hoover, 2008; Dias et al., 2010; Shariffa et al., 2017).
Structural analysis of native and modified cassava starches
In FT-IR spectrum, characteristic peaks were observed in the 900 to 1200 cm-1 region (Fig. 3A), associated with crystalline and amorphous regions (Zhang et al., 2012; Salcedo-Mendoza et al., 2018; Figueroa-Flórez et al., 2019; Zhang et al., 2019). The absorption bands at 1130 and 1160 cm-1 attributed to the vibration of the C-O, C-C or O-H bonds present in amylose and amylopectin molecules, increased the intensity of the signal, possibly by the depolymerization and breakdown of glycosidic bonds with the enzymatic treatment (Salcedo-Mendoza et al., 2018). In addition, a characteristic peak of the starch is band length at 995 cm-1 associated with the presence of water bound in the structure, whose band possibly moved to a greater availability of OH groups after depolymerization, favoring the capacity of the starch granule to retain and absorb water (Zhang et al., 2012; Salcedo-Mendoza et al., 2018). Absorption peaks at 1047 and 1022 cm-1 are closely related to the crystalline and amorphous structure (Ma et al., 2018; Figueroa-Flórez et al., 2019). The FT-IR spectra depend on the changes in the starch structure in a short-range order, defined as the double helix order, and that reflects the number of double helices ratio (ordered domains) with simple helices (amorphous domains) (Xu et al., 2018). The determination of the order degree (OD) was made from the FT-IR spectra (Tab. 3), showing an increase after the enzymatic treatment (ANN-C>UTS>HMG>ANN>NPS) that are consistent results with those reported in previous studies on the enzymatic modification of cassava starch (Salcedo-Mendoza et al., 2018; Figueroa-Flórez et al., 2019). Modified starches pretreated by UTS and ANN-C showed the highest values of OD, possibly due to the release of free amylaceous materials and reorganization/ recrystallization of amylose chains.
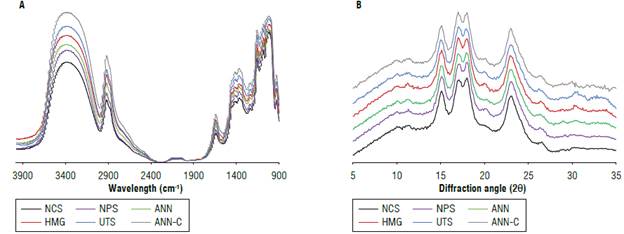
FIGURE 3 A) FTIR - infrared spectra and В) XRD - diffraction patterns in native and modified cassava starches. Native cassava starch (NCS); non-pretreated enzymatic modified starch (NPS); modified starch pretreated by annealing (ANN); modified starch pretreated by homogenization (HMG); modified starch pretreated by ultrasound (UTS), and modified starch pretreated by annealing and ultra-rapid freezing (ANN-C).
These results agree with the crystallinity index behavior estimated by XRD diffraction. In turn, Xu et al. (2018) linked the increase in the bands 1045/1024 ratio with the time and number of cycles of annealing treatment in bean starches and to the reorganization and a greater ordering of double helices of amylose and amylopectin in the crystalline domains of starch granules. By XRD diffraction, four crystallographic peaks were identified under angles of 15°, 17°, 17.8°, and 23° characteristic of tubular starches with diffraction pattern A-type (Fig. 3B) whose double amylopectin helices achieve a conformation in monoclinic form (Jayakody & Hoover, 2008). Starches showed higher intensity in peaks after physical and enzymatic treatments without evident changes in diffraction patterns. A similar behavior was found in previous studies possibly due to the decrease in amylose content in cassava starch granules (Salcedo-Mendoza et al., 2018; Figueroa-Flórez et al., 2019).
The crystallinity index increased in modified starches with respect to the control, in the following order: ANN-C>UTS>ANN>NPS>HMG (Tab. 3). The increase in CI of modified starches pretreated by UTS and ANN-C could be attributed initially to the removal of amorphous and unstable amylaceous materials by the action of the ultrasonic frequency, thermal energy, or enzymatic action (Li et al., 2018) affecting the structural and morphological properties as seen in FT-IR spectra and microscopy analysis.
Other research also has reported an increase in the crystallinity index of starch granules with the annealing process without affecting the characteristic diffraction pattern, due to the hydration and mobility of glucan chains by the action of temperature accelerate interaction and reorganization phenomena of amylose or amylopectin double helices (Jayakody & Hoover, 2008; Xu et al., 2018; Zhang et al., 2019), as well as the formation of additional intra-helical hydrogen bonds that result in an increase in crystalline sheet thickness during cooling (Jayakody & Hoover, 2008; Seetapan et al., 2016).
These results allow inferring that restructuring the short chains present in the amorphous and crystalline domains, together with the decrease of amylose content, altered the semicrystalline order of granules, and affected both the structural resistance of the granules to the enzymatic action and the behavior of thermal properties.
Thermal properties
In relation to gelatinization properties, significant changes in temperatures (T0, TP, and Tc) after the enzymatic process were estimated (Tab. 3), where the increase in T0 can be explained by the cleavage of glycosidic bonds that induce molecular and structural changes, affecting mainly the swelling process that regulates gelatinization (Chen et al., 2011; Dura et al., 2014). Likewise, recent investigations have explained that changes in transition temperatures are related to an increase of the crystallinity index, an indicator of structural stability and granular molecular resistance to gelatinization (Jung et al., 2017; Zhao et al., 2018; Xie et al., 2019). These results are consistent with those found from the values of OD and CI determined by FT-IR and XRD diffraction. No significant differences were detected in T0 values between samples of hydrolyzed starches with the NPS, ANN, HMG and UTS treatments, except for the exception of the ANN-C treatment. The difference in the behavior of T0 through the ANN-C cycle maybe due to the initial endothermic transition caused in granules during the annealing process, where water diffuses freely in the amorphous and crystalline regions altering the semicrys-talline order, together with the reorganization of short chains during ultra-rapid-freezing process (Waduge et al., 2006; Jayakody & Hoover, 2008; Seetapan et al., 2016; Zhao et al., 2018). Authors argue that a greater reorganization of double helices during annealing resulting from an accentuation in the mobility of the glucan chain leads to the formation of intra-helical hydrogen bonds changing the thickness of the crystalline sheet and ultimately the melting temperature of double helices, delaying gelatinization (Kiseleva et al., 2004). Analogous results have been found in the SP behavior (Tab. 1).
TABLE 3 Crystallinity index and gelatinization properties in native and modified cassava starches.
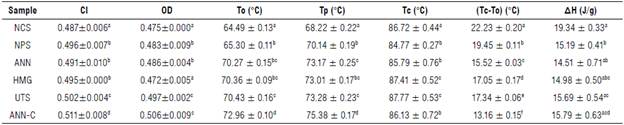
OD - order degree; CI - crystallinity index; To - onset temperature; Tp - peak temperature; Tc - conclusion temperature; ΔH - gelatinization enthalpy. Native cassava starch (NCS); non-pretreated enzymatic modified starch (NPS); modified starch pretreated by annealing (ANN); modified starch pretreated by homogenization (HMG); modified starch pretreated by ultrasound (UTS), and modified starch pretreated by annealing and ultra-rapid freezing (ANN-C). Different superscripted letters in the same column indicate significant differences for Tukey test (P<0.05).
The temperature range (TO-TC) showed a marked difference with the implementation of pretreatments followed by the enzymatic process with respect to the NCS samples. This behavior is supported by the premise that enzymatic hydrolysis causes both morphological and structural changes that limit the thermal behavior of starch granules (Gao et al., 2013; Li et al., 2018). Likewise, the increase in gelatinization temperature is more pronounced for TO (fusion of the weakest crystallites) and less for TC (fusion of stable and more perfect crystals) during the annealing process (Kiseleva et al., 2004; Jayakody & Hoover, 2008). For this reason, the decrease of TO -TC range with the ANN-C treatment, possibly due to a higher homogeneity, cooperative fusion, and more perfect crystals. This allows us to understand the most notorious changes on properties such as swelling, crystallinity, and susceptibility to enzymatic action after the hydrothermal pretreatment ANN-C. These same premises could explain the increase of AH in modified starches that is influenced by the hydration of the macromolecule and the interaction between polymer chains that alter the assembly of amorphous and crystalline lamellar structures (Jayakody & Hoover, 2008; Shariffa et al., 2017; Xie et al., 2019). However, the increase of ΔH in starches with different amylose contents after annealing can be correlated with an interaction of the short chains that can form new double helices or the granular conformation of the smectic type, whose reorganization will require higher values of energy to melt and start the gelatinization process (Kiseleva et al., 2004; Waduge et al., 2006; O'Brien & Wang, 2008; Dias et al, 2010).
Conclusions
The integrated processes of enzymatic biocatalysis and physical-thermal pre-treatment introduced here allowed the development of modified cassava starches with micro-porous surfaces. The enzymatic process caused significant changes in the structural characteristics of such starches, altering their semicrystalline order and gelatinization. The HMG decreased the size of the granules, thus, affecting their swelling capacity and gelatinization properties that are defined by a flexible and unstable granular configuration of small and fragmented granules. In turn, UTS pretreatments and the ANN-C cycle caused changes in morphological properties and homogeneously increased the size and volume of the pores in the granular surface, thus decreasing the range and enthalpy of the gelatinization. The ANN-C cycle can be an affordable and effective pretreatment in the production of thermally stable microporous cassava starches. As a result, we recommend optimizing the hydrothermal annealing process in the production of porous cassava starches considering variables such as starch/water ratio, heating time, and temperature.