I. INTRODUCTION
Silk fibroin (SF), secreted by the Bombyx mori worm 1, is a naturally occurring fibrous protein composed of the amino acid sequence (Gly-Ser-Gly-Ala-Gly-Ala) n 2; fibroin has been used as a biomaterial for various biomedical applications, including surgical sutures, membranes, drug delivery compounds, and more recently in regenerative tissue engineering 3-7. Due to its excellent biocompatibility properties, and to its minimal inflammatory response in vivo, SF is considered to be one of the best materials for tissue regeneration 2,8; additionally, it presents optimal characteristics of biodegradability and mechanical resistance. The potential of SF to replace the extracellular matrix collagen (EMC) offers many advantages over other synthetic biomaterials 9, and allows its use in various forms, such as sponges, gels, films, and nanofibers, and for the construction of scaffolds of various tissues, such as bone, cartilage, ligament, tendon, and blood vessels 10-12.
The standard biomaterial used in the repair of abdominal hernias is the surgical mesh of polypropylene (PP) that is a non-degradable synthetic polymer 13,14. PP has shown excellent mechanical properties, and a positive effect on abdominal hernia repair and pelvic organ prolapse; however, the post-implantation soft tissue response has been characterized by the presence of chronic inflammation, fibrosis, and decreased tissue dispensability 13,15. These sequelae may lead to discomfort for the patient, increased morbidity, and eventually removal of the mesh 13,14. The reaction to foreign bodies has been well characterized from a histopathological perspective; it has been found that, usually, the components of the innate immune response, especially macrophages, rapidly accumulate on the surface of the biomaterial, and thus play a determining role in the implant outcome 13,16.
The purpose of this study was to evaluate the influence of both SF and PP materials on cell growth in vitro.
II. METHODOLOGY
Fibroin was isolated from the buds of Bombyx mori silkworms, following the standard extraction procedure reported by Rockwood et al. ( 17, with slight modifications. The polypropylene mesh was obtained from the market for medical-surgical use.
A. Cell culture
HeLa cells (ATCC CCL-2) were seeded in 75 cm2 culture flasks (nunc™), in Dulbecco's Modified Eagle Medium (DMEM) with calcium and magnesium (Caisson LABS), supplemented with 1 % L-glutamine (Lonza Biowhittaker), 10 % fetal bovine serum (FBS) (Gibco® Invitrogen ™), and 1 % antibiotics (100 U/ml penicillin, 100 mg/ml streptomycin, and 25 ug/ml amphotericin) (Lonza Biowhittaker®). Cells were incubated at 37 °C in an atmosphere of 5 % CO2, and a relative humidity of 90 % (Binder incubator). Subculture was maintained for 8 days, and the DMEM medium was changed every 48 h. Subsequently, the DMEM medium was removed, and the cells washed twice with 5 ml of phosphate buffered saline (PBS) (Baxter), followed by treatment with trypsin/ EDTA solution (Lonza Biowhittaker®). A cell pellet was collected after centrifugation at 1500 rpm for 10 minutes (Hettich Rotofix 32A centrifuge) at room temperature, followed by removal of the supernatant. A homogenous cell suspension was obtained by mixing the cell pellet with 1 ml of medium supplemented with L-glutamine, 10 % of fetal calf serum (FBS), and antibiotics, previously evaluated for the presence of microbial contamination.
B. Direct cytotoxicity test
In a 96-well plate (BrandPlateTM) about 7.0 ± 0.4 mg per well of sterile material was added (n = 9 fibroin, n = 9 polypropylene, and n=4 cells control groups for each study). The HeLa cells were then seeded directly on each material, at a density of 1 x 104 cells per 100 ul of medium. The plates were incubated at 37 °C and 5 % CO2 for 72 hours.
C. Indirect cytotoxicity test
Sample extracts of fibroin and polypropylene materials were prepared in 50 ml falcon tubes for each type of material (n=3), by immersing them into the previously described supplemented DMEM medium. The sample/ extraction ratio volume was 1 mg/10 ul, and the incubation conditions were 37 °C and 5 % CO2 for 72 h.
HeLa cells were seeded at a density of 1x104 cells per 100 ul of DMEM medium supplemented in 96-well cell culture dishes. After 24 h and cell adhesion testing, the culture medium was discarded and replaced with 100 ul of the material extract (n=3 for each falcon tube for fibroin and polypropylene), DMEM was used as the control (4 wells for fibroin, and 4 wells for polypropylene). The plates were incubated under standard conditions, at 37 °C with 95 % humidity and 5 % CO2 for 72 h.
D. Evaluation of cellular cytotoxicity by the MTT assay
In order to determine cell proliferation in the culture, the number of cells per sample was measured by the MTT (molecular probes®-Life Technologies™) assay for bromide [4, 5-dimethyl-2-thiazoyl- 2, 5-diphenyltetrazolic]. The protocol supplied by Life Technologies™, in which the culture medium was removed, was replaced with 100 ul of HBSS (Lonza Biowhittaker®), then 10 ul of the MTT solution (12 mM) was added to each well, and the plates were incubated for 4 h at 37 °C and 5 % CO2. Subsequently, 85 ul of the HBSS + MTT mixture was withdrawn, and a 50 ul aliquot of DMSO (Sigma-Aldrich®) was added to each well by vigorously mixing, and rapidly incubating for 10 minutes at 37 °C, then each well was again mixed with a micropipette, and the plates were finally read at an absorbance of 540nm in a microplate reader (Synergy H1 Biotek®). Additionally, a calibration curve was performed for the following concentrations 0.1, 0.3, 0.7, 1.0, 2.0, 5.0 and 8.0 x 104 cells/well, following the same protocol.
E. Statistical analysis
A completely randomized experimental design (DCA) was performed for each of the evaluation tests. Each response variable was tested for normality and homogeneity of variances, and a variance analysis (ANOVA) followed by the DUNCAN post hoc multiple comparison test at a significance level of 5 % were performed. Statistical analyses were conducted using the SPSS/PC program (SPSS Inc., Chicago, IL, USA).
III. RESULTS
The results obtained with respect to cell growth by means of the direct and indirect cytotoxicity test for fibroin (test F) and polypropylene (U assay) materials are described below.
In the direct cytotoxicity test for fibroin (F), although the fibroin sample (F) showed 5.4 % more living cells than the control (CF), this difference was not statistically significant (Fig. 1). In contrast, the test for the polypropylene sample (U) showed a significant statistical difference (p <0.005) in cell growth compared to the control (CU); in this assay the polypropylene (U) sample showed 80.2 % less living cells than the control (CU) (Fig. 1).
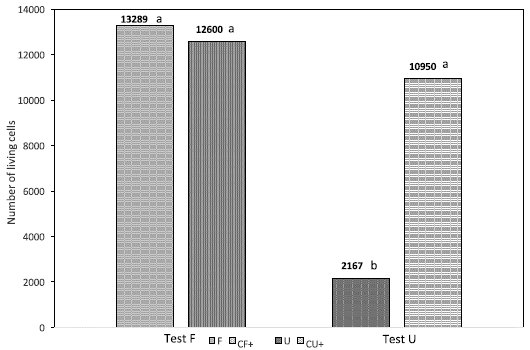
Fig. 1 Direct cytotoxicity test. Comparison of fibroin samples (F test) and polypropylene samples (U test); CF: positive control sample for the fibroin, CU: positive control sample for the polypropylene.
For the F test, the indirect cytotoxicity test showed a significant statistical difference between the fibroin (F) sample and the positive control (CF); the positive control (CF) showed 54900 living cell on average, while the fibroin (F) sample showed 39822, representing a 37.9 % increase in living cells in the control (CF) group (Fig. 2). For the U assay, the control (CU) presented 52225 living cells on average, while the polypropylene (U) sample only had 111 living cells, representing a reduction in the amount of living cells by 99.8 % in the U sample with respect to the control group (CU) (Fig. 2).
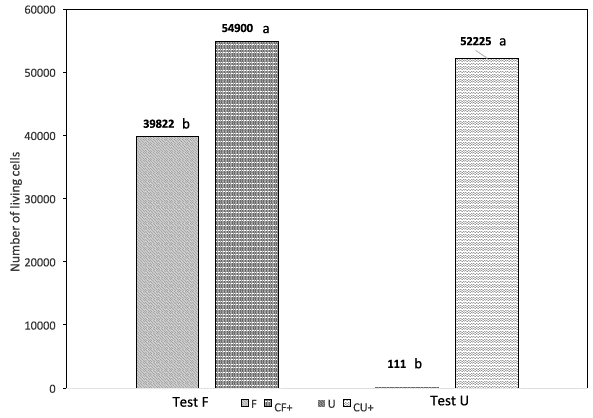
Fig. 2 Indirect cytotoxicity test. Comparison between fibroin (F test) and polypropylene (U test) samples. CF: positive control sample for the fibroin, CU: positive control sample for the polypropylene.
When comparing the direct and indirect cytotoxicity tests for the fibroin (F) and the polypropylene (U) samples, we found a statistical difference in the average number of living cells in favor to the F sample, regardless of the cytotoxicity test (i.e., direct or indirect). A difference greater than 6 times the number of living cells in the polypropylene sample versus fibroin sample, and a difference greater than 100 times for the direct and indirect tests respectively was clearly seen (Fig. 3); this suggests that the fibroin material (F) allows for greater cell growth. Furthermore, when comparing the materials analyzed with MTT in each test, we found that in the indirect test, fibroin had a higher cell growth, whereas in the direct cytotoxicity test, the polypropylene had the highest growth (Fig. 4).
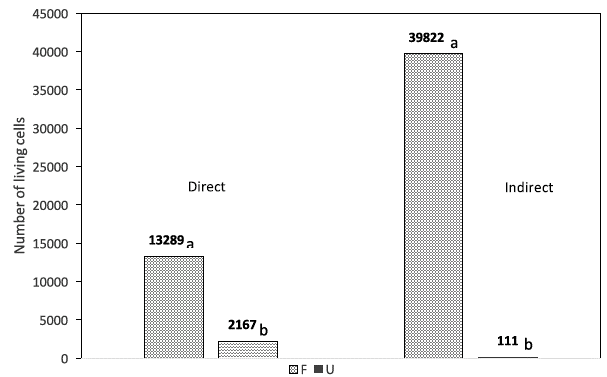
Fig. 3 Average number of living cells with fibroin (F) and polypropylene (U) samples for direct and indirect cytotoxicity tests.
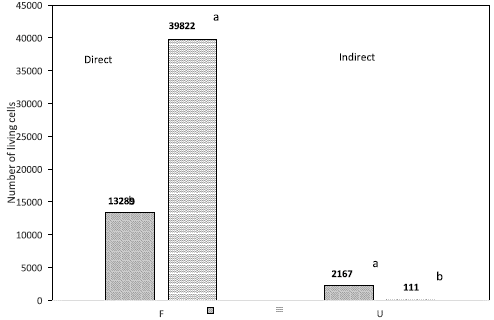
Fig. 4 Comparison between direct and indirect cytotoxicity tests for the evaluated material: fibroin (F), and polypropylene (U).
The comparison of the direct and indirect tests for the fibroin samples (F) showed that a higher average number of living cells (39822) was obtained through the indirect method than through the direct method (13289) (Fig. 4); this difference represents a reduction of 66.6 % of living cells in the direct test. For the polypropylene samples (U), a statistical difference between the two tests was observed, with 2167 living cells on average in the direct test and 111 in the indirect; this ratio represents a 94.9 % reduction of living cells in the indirect test with respect to the direct test (Fig. 4). It should be noted that while in the F sample the indirect test allowed a significant increase of living cells, in the U sample the opposite was observed. Visual results of differences in density and cell adhesion are shown in figure 5.
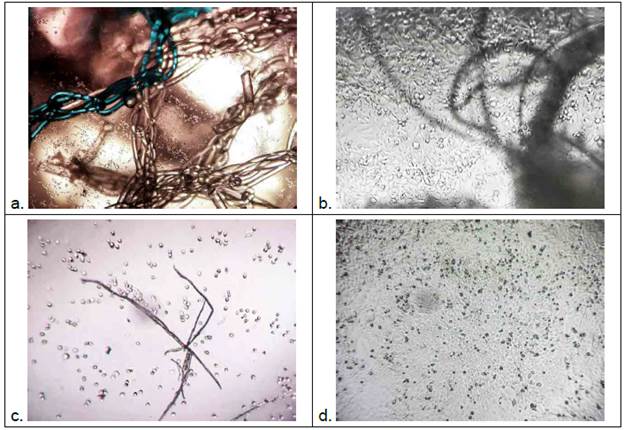
Fig. 5 a. Hela cells (4x) in direct contact with the polypropylene mesh; B. Cells in direct contact with fibroin material (10x); C. Hela cells (4x) (indirect test) with low adherence to the surface subjected to polypropylene extract (concentration 1x); D. Cell monolayer (4x) (indirect test), high adhesion is observed, cells subjected to fibroin extract (concentration 1x).
IV. DISCUSSION
Fare et al. ( 18 studied the macroscopic structure of fibroin and its use in the repair of the anterior cruciate ligament; additionally, they determined the viability of the fibroblasts in fibroin extracts through the indirect cytotoxicity test, and found that cells cultured in such extract showed no decrease in viability and much less damage. In this test, the authors used L929 fibroblasts due to their high adhesion to fibroin 18,19.
In our study, we used Hela cells, and applied the respective cell viability tests. In the indirect cytotoxicity test for fibroin, a significant difference was observed with respect to the control (cultured only with DMEM medium), with a larger average number of living cells present in the control than in the fibroin assay. The marked decrease in living cells in the polypropylene assay was also evident, resulting in a 99.8 % reduction in cell viability. In contrast, for the direct cytotoxicity test, a statistically significant difference was not obtained in the fibroin assay with respect to its control group, with only a difference of 5.4 % of more living cells in the fibroin. As for the commercial mesh, the number of living cells was lower with respect to its control group (80.2 %).
Our results agree with studies showing alterations in human tissue caused by materials such as polypropylene. Sternschuss et al. ( 20 found that the pristine form of the material is non-toxic and compatible with human tissue; however, by adding numerous substances, they were able to produce toxic reactions that depended on the migration of these materials into the body after implantation. The sterilization process of polypropylene, at high temperatures, leads to the diffusion of toxic substances toward the polymer surface. Thus, the substances found on its surface migrate more easily into the extracellular body fluids, which can result in an inflammatory response in patients who have polypropylene meshes implants, showing elevated levels of tumor necrosis factors, cytokines, and interferons associated with these adverse effects 20. On the other hand, the difficulties in cell adhesion to the PP mesh have been documented in various studies. For instance, Zhang et al. ( 21, who used polypropylene meshes implants for transvaginal repair, found foreign body reactions due to deposition of large amounts of fibrous tissue, increasing the risk of mesh erosion, urinary problems, bleeding, and infection; these results could be attributed to the hydrophobicity of PP, which limits cell adhesion to tissue, and scar formation. Furthermore, Zhang et al.21 modified the polypropylene meshes with a polypeptide material called fibroblast growth factor (bFGF) that promotes cell growth and differentiation, and observed a greater adhesion of fibroblasts to the PP, and a greater degree of organization in the deposited collagen, compared to the PP used in the absence of this growth factor.
Ina study conductedinMacacus rhesus, thepost-implant inflammatory response to prolapse was characterized, elucidating the presence of proinflammatory cells after the PP mesh implantation 22; in this study, the cellular immune response generated by the polypropylene mesh showed a predominance of proinflammatory cells type M1 macrophages within a few weeks of the surgical procedure; the researchers also found other proinflammatory cells to a lesser extent, such as CD45 (panleucocytes) that were distributed in the interstitium between the PP mesh and the host tissue, in addition to CD68-type macrophages that were widely distributed in each fiber of the mesh, while other cells were distributed in the periphery of its surface 22. Several researchers have studied the post implantation effect with various polymeric materials, and have consistently observed the presence of inflammatory cells in polypropylene mesh tissue samples up to 8 years after their implantation; other researchers have observed the degradation of this material after implantation in humans 20. An immune response has been observed in the acute inflammatory phase, followed by the secretion of acid by the macrophages, which attacks the biomaterial, starting the oxidative process that was initiated by the heat due to the manufacturing process of the mesh; in turn, the degradation of polypropylene releases additives that contribute to the inflammatory reaction. All these processes culminate with the appearance of fibrosis, causing mesh contraction and chronic pelvic pain, since the nerve fibers are trapped into the fibrotic tissue. Eventually, the inflammatory response may lead to the erosion or contamination of the mesh, causing infection at the implant site 20. Lastly, polypropylene has been extensively studied, and is recognized as a super-hydrophobic material that has good physicochemical characteristics 23; however, when analyzing its biological properties, PP shows problems of cellular compatibility due to the lack of charge on its surface, which does not allow suitable binding of molecules, such as integrins, cadherins, and selectins, among others 24.
Fibroin is a protein considered to positively influence cell proliferation and metabolism, because it is composed primarily of amino acids such as alanine, glycine and serine that have terminal carboxyl groups 25; these carboxyl groups confer an anchoring property to the protein surface, allowing the interaction with different types of ligands: peptides, adhesion proteins, hormones, and growth factors 26. In our study, direct and indirect cytotoxicity tests were performed for fibroin and polypropylene, observing greater cell growth in the fibroid sample, regardless of the cytotoxicity test applied.
Under different approaches, several studies have confirmed the low cytotoxicity of silk fibroin. For instance, Jia et al. ( 27 found high colloidal stability and low cytotoxicity in covers made of silk fibroin and gold nanoparticles, in addition to the fibroin inherent biocompatibility; in this study, the researchers evaluated by MTT the preliminary cytotoxicity with fibroin/gold-nanoparticles, and gold nanoparticles, finding insignificant cytotoxicity in both groups when the concentrations of these substances were below 4.2 mg/ml 27. Several investigators have found dose-dependent cytotoxic effects when using gold nanoparticles, whose increment in cytotoxicity seems to be caused by aggregation, and have attributed the low cytotoxicity of these nanoparticles to two aspects: the inherent biocompatibility of silk fibroin, and the good colloidal stability 27,28. With respect to colloidal stability, it has been observed that small gold nanoparticles could perform endocytosis, and thus form internal aggregates that produce cytotoxicity 28. Therefore, prevention of particles aggregation in the internal environment of the cells can, to a large extent, reduce such toxicity. The MTT test compared the materials used in our study, and found a higher cell growth in the indirect test for fibroin, while polypropylene always showed a significantly low viability independent of the type of test; however, studies on polypropylene surface modifications to improve material biocompatibility are still ongoing 29-31.
V. CONCLUSIONS
The results of this study showed the advantage of using fibroin obtained from the silkworm (Bombyx morí), in terms of viability and cell adhesion when compared to synthetic polypropylene polymer (PP). Fibroin has demonstrated a better biocompatibility, which may encourage further studies where this biomaterial can be used to replace surgical mesh in the repair of abdominal hernias.