I. INTRODUCTION
Several bone injuries and defects cause bone loss. Although these defects have been commonly treated with conventional therapies that involve the implantation of autologous, allogeneic or xenogeneic tissue, still complications associated with these implants arise, such as the limited amount of bone to be extracted, the cell death at the extraction site (pain, infection, hematoma, abdominal hernias, etc.), and the potential risks of infectious and immunological diseases (e.g., hepatitis, HIV, prions, etc.). Therefore, it is necessary to explore various alternatives like the use of biomaterials [1] that are bioactive, biocompatible, and that have adequate mechanical properties to withstand the loads to which they will be subjected. Biomaterials that help manufacture hard tissue cell growth platforms, known as scaffolds, provide mechanical resistance to tissues. The scaffolds can be found in different biomaterials, mostly the ceramic type, and can be obtained through different methods such as infiltration, gel-casting, and compaction, among others.
Given that the skeletal system endures heavy loads, the scaffolds that serve as support must provide solid structure, porosity, interconnectivity, and adequate composition, considering that the goal is to develop a structure similar to that generated by collagen cross-linking fibers in the bone [2]. Titanium dioxide is a biomaterial of inert nature, which, due to its ceramic characteristics, provides similar bone properties. This biomaterial has been used to produce scaffolds as an alternative to hydroxyapatite, a biomaterial commonly used that lacks the sufficient mechanical properties for this purpose [3].
In this project, we prepared titanium dioxide scaffolds using infiltration, lyophilization, and compaction, and assessed them mechanically. We used degradability tests and analyzed how the preparation techniques improve the mechanical properties and the porosity of the scaffolds. We established the behavior and degradation time of the samples in Simulated Body Fluid (SBF) and compared the results with those reported in literature for bone.
II. METHODS
A. Scaffold preparation
Scaffolds were prepared using three different techniques: infiltration, lyophilization and compaction. For the infiltration technique, we started with a titanium dioxide solution composed of distilled water and 50% solids, to which sodium polyacrylate was added as a dispersant; the solution was stirred for 10 minutes. Then, with the desired mold size, the polymeric foam was impregnated with the ceramic solution, applying pressure to achieve homogeneous wetting and then dried in an oven for one hour at 120 °C. The same impregnation procedure was repeated. The material was calcined with heating ramps at 450 °C for one hour, followed by 750 °C for one hour, and finally the scaffold was sintered at 1200 °C for two hours.
The scaffolds produced by compaction were prepared with a mixture of titanium dioxide, sodium chloride and corn flour to provide porosity differences. Once the mixtures were made and homogenized, they were compacted using a press with a load of 30 Mpa for two minutes. Subsequently, the formed scaffolds were taken to a muffle to undergo calcination and sintering using the same temperatures used during infiltration. Finally, the scaffolds obtained were subjected to repetitive washes to solubilize the sodium chloride.
To prepare the scaffolds with the lyophilization technique, we started with a titanium dioxide solution composed of distilled water and 50% solids, to which sodium polyacrylate was added as a dispersant; the solution was stirred for 10 minutes. Afterwards, we added different concentrations of collagen (10%, 15%, 20% and 30%) and PVA (10% and 15%) for the different scaffolds. The solutions were subsequently frozen at -80 °C and underwent lyophilization for 48 hours.
B. Scaffold characterization
The morphological characterization of the scaffolds was carried out using a Scanning Electron Microscope (SEM) to describe the shape, pore size, porosity type, and interconnectivity. We used a Scanning Electron Microscope Jeol JSM-6490LV that operates with a 20 KV voltage acceleration and is coupled with an EDS brand Oxford Instrument INCA PentaFETx3 for chemical analysis, which allowed to differentiate the elements present in the sample and their proportions. The samples were coated with a layer of gold and observed at different magnifications.
The procedure to determine the scaffolds' porosity followed Liu [4]. Initially, we obtained the net weight of the dry samples and saturated them with distilled water according to the NTC 4321-3 norm, whose protocol instruct immersing the samples in water, removing the excess of water, and weighing them. In addition to measuring the scaffolds' porosity, calculations were made through image analysis using a software coupled to the SEM; this software allowed processing the images on a gray scale and measuring the size of the different pores obtained, which were subsequently averaged. The porosity was also measured by the MOTIC software attached to the stereoscope.
C. Scaffold evaluation
The mechanical evaluation was performed through compression tests under the ASTM D882 standard using a Diggimes universal test machine, with a load cell capacity of 4900 N, a loading speed of 5 mm/min, and a displacement or deformation limit of 75%. The samples had a cylindrical shape with average heights of 22 mm and average radius of 9 mm.
The degradation test was performed according to the ASTM F1635-11 standard for weight loss. The dried samples were weighed, immersed in SBF at a neutral pH (7.0), and incubated at 37 °C for 28 days. Testing was executed three times. Once the incubation period ended, the samples were removed from the solution and dried to proceed with the weighing. In addition, the pH of the SBF solution was measured to determine whether degradation had any effect on this value.
III. RESULTS
In general terms, the morphology of the resulting scaffolds was similar (Fig. 1), however, they differed in their shape due to the type of mold used. The scaffolds obtained by infiltration with polymeric foam (Figs. 1a, 1b) maintained the structure copied from the polyurethane foam, with differences in porosity since the pores can be clogged before the impregnation sequences. The scaffolds obtained by compacting with different percentages of NaCl (Figs. 1c-1e) had no significant morphological differences when examined with the naked eye, but a strong structure was detected even after the washing was completed to dissolve the salt, maintaining dimensional stability. The scaffolds compacted with organic material (Figs. 1d-1g) retained the block shape after sintering, however, the structural stability achieved was not as good as with the salt, falling apart when manipulated.
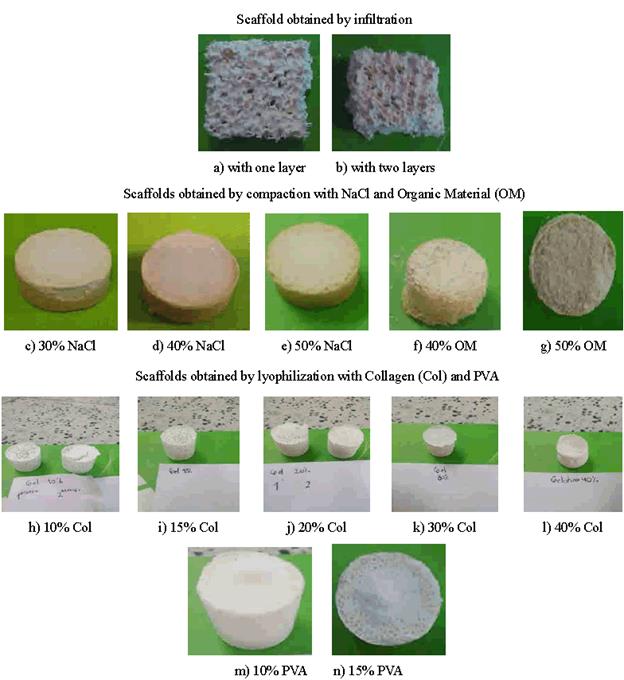
FIG. 1 Morphology of the scaffolds obtained by infiltration (a-b), compaction (c-g), and lyophilization (h-n).
As the percentage of collagen increased, the obtained scaffolds became more compact with a more continuous matrix, achieving a better pore distribution and homogeneous pore size. The consistency to touch was much more stable and retained the shape and dimensions longer when dry; moreover, a higher percentage of PVA increased porosity without affecting the structure after lyophilization.
A. Mechanical evaluation of the scaffolds
The mechanical properties obtained showed that for the infiltrated samples, the number of layers and sinters influenced the mechanical resistance, being the compressive strength greater for the sample with one layer (13.3 MPa) than for the sample with two (4.6 MPa) (Table 1). This may occur due to the impregnation process of intermediate drying, where the particles do not adhere easily and, when sintered later, acquire a lower capacity to resist mechanical stress due to the formation of an interface, making the scaffold a sample with low particle adhesion [5,6]. In contrast, the samples compacted with NaCl increased in compression resistance slightly when the percentage of NaCl increased from 30% to 40% but decreased again when NaCl increased to 50% (Table I). Scaffolds so compact displayed an unstable behavior to calculate the mechanical properties, perhaps because solubilization was inadequate, leaving salt crystals inside the sintered blocks. The samples compacted with organic material showed that increasing the percentage of organic material decreases the mechanical properties; this phenomenon occurs when the organic material degrades during sintering of the scaffold and generates a greater porosity within the material, as reported by Saiz [7].
The lyophilized samples with collagen showed a direct relationship between the percentage of collagen and the mechanical strength of the material: the lower the percentage of collagen, the lower the mechanical strength (Table 1); the higher the collagen percentage, the fewer defects in the internal structure of the material [8], which increases the scaffolds compressive strength without fracturing. Moreover, the samples lyophilized with PVA showed that increasing PVA percentage decreases the compressive strength, which suggests that the nature of the polymer binder influences the mechanical behavior strongly (Table I).
After 28 days, the scaffolds obtained by infiltration and by compaction with NaCl did not degrade (Figs. 2a and 2b), whereas the rest of the scaffolds deteriorated considering that the weight of the dried sample changed substantially from the initial one. Noticeably, the samples obtained by lyophilization failed before 24 hours, probably because of the presence of PVA and collagen, which dissolve with an increase in temperature, while the scaffolds obtained by infiltration showed a better structure. Additionally, the scaffolds obtained by lyophilization with collagen absorbed water considerably, increasing their volume with regards to the initial calculation; while those with PVA tended to deform, losing their original shape, and becoming a viscous paste, therefore, the porosity calculations yielded unreliable results.
The results show that the porosity of the manufactured scaffolds varied between 52% and 67% (Table II); this range is within that reported in the literature for trabecular bone (30-90%) [9,10]. However, the porosities found using the three techniques differed. For example, the scaffolds infiltrated with two layers showed lower porosity because the ceramic solution sealed the pores once they were formed. Likewise, the porosity of the samples obtained by compaction depended on the binder material used; for instance, the sodium chloride, although soluble, crystallized at high temperatures, affecting the speed of solubilization, and given the short washing time, the porosity was lower than with the organic material, which seems to have evaporated almost completely with the sintering temperature. Finally, the porosities in the lyophilized scaffolds with different percentages of collagen and PVA were directly proportional to the amount of agent used: the more collagen and PVA used, the greater the percentage of porosity; this occurs because the amount of solid solvent to sublimate during lyophilization affects the pore morphology differently (Fig. 3).
The microscopies reveal structures with superficial porosities (Fig. 3). The scaffolds obtained by infiltration (Fig. 3 a) retained the polymeric foam structure and had a homogenous pore distribution all around the surface, which would guarantee good cell adhesion. Additionally, the scaffolds obtained by compaction, both for 30% NaCl and 50% organic material, had very compact structures with closed porosities (Figs. 3b, 3c), although the sample compacted with organic material had a little more porosity. The lyophilized scaffolds had useful porosities for medical applications.
As reported in the literature, for an adequate cellular adhesion to take place, the pore size should be between 5 µm and 500 µm [3]. Comparing these data with the sizes observed with the stereoscope, the scaffold that approximates the most is the one obtained by lyophilization with PVA, with an average pore size of 592.3 µm (Table III), a 18% deviation with respect to the maximum value reported in the literature.
The morphological differences between all the samples were verified with the microscopy images. The porosity inside the samples compacted with NaCl was lower, corroborating the crystallinity of the salt and the non-solubility during the final washings (Fig. 4b); whereas the opposite was observed in the samples compacted with organic material, which apparently evaporated with the sintering temperature. The samples lyophilized with collagen (Fig. 4d) and PVA (Fig. 4e), as well as the samples obtained by infiltration, had higher porosities and even pore inter-connectivity between them.
IV. CONCLUSIONS
The different binders used to carry out the lyophilization technique caused an elastic behavior in the samples, obtaining acceptable values for Young's Modulus. Furthermore, the porogenic agents (e.g., sodium) used in the compaction technique were adequate, but they require rigorous washing to guarantee the solubility of the salts and the achievement of the appropriate pore size. Finally, the scaffolds obtained through lyophilization using 10% PVA had a more similar pore size to that of the bone, along with similar mechanical properties; this makes this technique one of the most promising in manufacturing scaffolds useful for bone tissue engineering.