I. INTRODUCTION
Cylindrical steel tanks are special structures built for the purpose of storing liquids such as water, fuels, chemicals, hydrocarbons and their derivatives, among others; they are widely used in the gas, petrochemical and energy industries. These structures are susceptible to suffer some type of failure in the event of earthquakes of medium and high seismic magnitudes [1], such as large deformations in the upper and lower part of the tank wall, rupture in the connections between the tank and the pipes, rupture of the welded joint between the walls and the bottom, buckling in the lower part of the tank walls, among others.
Earthquakes such as those that occurred in 1960 in Chile; 1994 in Northridge, California; 1995 in Kobe, Japan; 1999 in Kocaeli, Turkey; Haiti and Chile in 2010; show evidence of the susceptibility of this type of structures to seismic actions. For this reason, the response of such structures to intense earthquakes has been the subject of numerous experimental and analytical investigations, mainly aimed at determining both the influence of hydrodynamic pressures and proposing reliable procedures to evaluate the dynamic forces induced by the lateral component of a seismic movement from simplified mechanical models [2]. The interest for a safe behavior under seismic loads lies not only in the cost of replacement in case of failure, but also in the environmental disasters that an accident can cause [3].
The analysis of liquid storage tanks subjected to seismic action requires specific consideration of the hydrodynamic forces exerted by the fluid on the tank wall, since it has been shown that the dynamic response of this type of structure is affected by the interaction between the fluid and the structure, and therefore, such interaction must be considered in the design of the tanks. According to tests carried out by Graham and Rodriguez [4], it was proved that the oscillating fluid inside the tank has two components, one that moves in unison with the tank (impulsive component) and another one that represents the wave motion on the surface (convective component); based on these studies, Housner [5] - [7] proposed the first simplified models for the calculation of the dynamic response of tanks.
The development of mechanical models, which approximate the response of tanks, has considerably simplified the analysis of this type of structures; these models represent the tank-liquid system by means of an equivalent mass-spring system, which simplifies the evaluation of hydrodynamic forces and allows predicting the response in terms of basal shear, overturning moment and maximum wave height. These simplified models are currently used by several design codes, such as API 650 (American Petroleum Institute), NZSEE (New Zealand National Society for Earthquake Engineering), Eurocode 8, among others.
On the other hand, the appearance of finite elements for the solution of several engineering problems has allowed to achieve important advances in the field of fluid-structure interaction, as in the case of storage tanks, allowing a better approximation to the numerical solution of the hydrodynamic problem, generated by seismic action. The finite element method (FEM) is often used to model the fluid-structure interaction in tanks with flexible walls, as is the case of cylindrical steel tanks. These models, based on the use of finite elements, constitute a way to represent certain real, complex physical phenomena.
That is why, the present research focuses on evaluating the fundamental parameters of dynamic response of a vertical cylindrical tank, subjected to horizontal seismic excitation, located in the southeastern area of Cuba, specifically in the province of Santiago de Cuba, by applying the simplified procedure established in the API 650 design code of 2013 and the parameters that characterize the seismic hazard in the area where the tank is located; with respect to the response obtained as a result of the use of finite elements, and the response spectrum method, implemented in the professional software SAP2000, version 22.0, with which a much more adequate and accurate response to the problem can be assumed. A study of this type makes it possible to establish correlations between the response parameters obtained, from which the reliability of the simplified procedures commonly used is validated.
II. METHODS
The study presents the dynamic analysis of a vertical cylindrical steel tank of approximately 12000 m3 of capacity, mechanically anchored to the foundation, and subjected to horizontal seismic excitation taking into account different filling percentages (75 and 100 %). The tank has a diameter of 36 m and a total wall height equal to 11.6 m (H/D<1.33), the walls have an equivalent thickness of 0.016 m, and both the bottom and the roof have a thickness of 0.008 m. The tank material has a modulus of elasticity equal to 1.999E+08 kN/m2, and the stored liquid has a density of 9.71 kN/m3 (Diesel fuel). This tank is located in the province of Santiago de Cuba, the most dangerous seismic zone of Cuba (zone 5), in a soil profile type E.
The analysis is performed from two different approaches, (1) through the application of an equivalent mechanical model described in the API 650 (2013) design code, by which the fluid-structure interaction is represented by an equivalent mass-spring system that simplifies the evaluation of hydrodynamic forces and allows to predict the response in terms of basal shear, overturning moment, and maximum wave height; and (2) through the development of a mathematical model based on the finite element method (FEM), in which the simplified modal analysis as a response spectrum method is used as a calculation method, using the CQC (Complete Quadratic Combination) as a superposition formula, solving the eigenvalue problem by the Ritz vector method. Finally, a comparison is made among the applied procedures regarding the fundamental dynamic parameters that characterize the dynamic response of the tank.
A. Simplified Model
The simplified model is based on the equivalent mechanical system (mass-spring) established in API 650 (2013), for which a spreadsheet is elaborated and supported in Excel with the formulations described in the code mentioned above. Thus, obtaining the tank response parameters quickly and safely by reducing the computation time and calculation errors. In the calculations performed, the following considerations are taken into account: (1) the parameters that characterize the seismic hazard are those corresponding to the province and municipality of Santiago de Cuba, according to table 1; (2) it is considered a response reduction factor equal to two for the convective mode and four for the impulsive mode; (3) the importance coefficient is equal to one; (4) the damping value for the convective and impulsive modes is 0.5 %, and 5 % respectively.
Table 1 Seismic hazard parameters established for Santiago de Cuba [9].
Province | Municipality | S0(g) | Ss(g) | S1(g) | TL(seg) | Zone |
---|---|---|---|---|---|---|
Santiago de Cuba | Santiago de Cuba | 0.513 | 1.035 | 0.428 | 6.00 | 5 |
To determine the resultant forces at the tank's base, the Square Root of the Sum of the Squares (SRSS) method is used for the impulsive and convective components. This is justified due to the significant difference between the impulsive and convective principal periods.
B. Complex Model
In order to perform the dynamic analysis in a complex way, the professional software SAP2000, v.22.0 was used, for which it was necessary to define a response spectrum that covered a wide range of periods associated with the predominant modes of the structure and the liquid in motion. For the construction of such a spectrum, a response spectrum with damping of 5 % (impulsive mode) is first calculated and then reduced to 0.5 % (convective mode) by means of the amplification factors developed by Newmark and Hall in 1982 [10]. Then, considering the existing decoupling between the convective and impulsive modes of vibration, it is possible to define ranges of periods over which the behavior is only impulsive or convective; thus, to perform the combined spectrum, the following considerations must be included [11]: (1) the impulsive response is characteristic of short period, typically, these periods are less than TS; (2) the convective modes are characterized by presenting long period responses, usually greater than 2 seconds; and (3) there is a range of periods between 1 and 2 seconds in which there is no modal participation; therefore, they are negligible. The spectrum branches in this zone can be interpolated to join the impulsive and convective spectrum portions (range between 1 and 2 seconds).
This type of analysis allows the application of the corresponding response reduction factor associated with each branch in the same spectrum. Thus, Figure 1 shows the combined spectrum (black dashed lines) obtained from spectra with different damping values. Finally, in red color and with dashed lines, a combined and reduced spectrum is shown with its corresponding response factor, which is used to model seismic actions.
Figure 2 shows the geometry of the computational model generated in which parameters such as effective masses, effective heights, and elastic springs are introduced to link the convective mass to the tank walls previously determined in the two-dimensional analysis.
The tank is analyzed under the action of two orthogonal horizontal components of ground motion, using combinations of loads (1-4).
Where: D: effects generated by dead loads (own weight of all structural elements), L: effects generated by live loads, F: load due to the weight of the stored fluid, with precise knowledge of the pressure and maximum height of the same, E: Effects generated by seismic action.
Since the analysis performed is modal, the SRSS is used as the modal superposition formula given that the movement of the impulsive and convective masses occur with very distant periods and in an uncoupled manner. In this sense, the design code API 650, 2013, remarks that when the responses are widely separated, an almost simultaneous occurrence of maximum values can occur; however, the convective response takes longer to accumulate than the impulsive response. Therefore, it is likely that the impulsive component decreases when the convective component reaches its peak.
III. RESULTS
A. Simplified Analysis
After applying the simplified procedure described in API code 650 (2013), Table 2 shows the calculated response parameters in terms of periods, resultant forces, stresses, wave height, among others.
Table 2 Response parameters. Simplified model.
Parameters | Symbol | Unit | % of filling | |
---|---|---|---|---|
100% | 75% | |||
Impulsive period | Ti | seg. | 0.19 | 0.17 |
Convective period | Tc | seg. | 6.85 | 7.4 |
Impulsive mass | V | kN.s²/m | 4308.43 | 2442.27 |
Convective mass | Mc | kN.s²/m | 6909.39 | 5921.79 |
Impulsive height | hi | m | 4.35 | 3.26 |
Convective height | hc | m | 6.39 | 4.61 |
Shear | V | kN | 10114.14 | 6483.12 |
Moment | M | kN-m | 51877.39 | 25799.96 |
Wave height | d | m | 1.68 | 1.44 |
Compressive stress | Sc | kN/m² | 4523.77 | 2922.85 |
Tangential tensile stress | St | kN/m² | 118637.71 | 87378.51 |
B. Complex Analysis
As a result of the modeling carried out using the finite element technique, Table 3 shows the analysis results in terms of periods, reactive forces, and stresses. Likewise, tables 4 and 5 present the modal participation of the masses in the dynamic behavior of the analyzed variants, where it can be observed that the models have been calculated taking into account a modal participation of the masses higher than 90 %, for which a sufficient number of modes were introduced to achieve a satisfactory response of the system.
Table 3 Response parameters. Computational model.
Parameters | Symbol | Unit | % of filling | |
---|---|---|---|---|
100 % | 75 % | |||
Impulsive period | Ti | seg. | 0.187 | 0.156 |
Convective period | Tc | seg. | 6.91 | 7.46 |
Shear | V | kN | 8187.34 | 5622.31 |
Moment | M | kN-m | 43403.61 | 23677.83 |
Compressive stress | Sc | kN/m² | 5473.76 | 3653.56 |
Tangential tensile stress | St | kN/m² | 142365.25 | 104854.21 |
Table 4 Modal participation of tank masses, at 100% of their filling capacity.
Case | Mode | Period [sec.] | UX | UY | Sum. UX | Sum. UY |
---|---|---|---|---|---|---|
Mode | 1 | 6.914275 | 0.62099 | 0 | 0.62099 | 0 |
Mode | 16 | 0.187267 | 0 | 0.18034 | 0.62112 | 0.80145 |
Mode | 20 | 0.0592 | 0 | 0.13267 | 0.92755 | 0.95073 |
Table 5 Modal participation of tank at 75% of its filling capacity.
Case | Mode | Period [sec.] | UX | UY | Sum. UX | Sum. UY |
---|---|---|---|---|---|---|
Mode | 1 | 7.46295 | 0 | 0.70493 | 0 | 0.70493 |
Mode | 32 | 0.156705 | 0 | 0.11521 | 0.70534 | 0.82034 |
Mode | 40 | 0.035224 | 0 | 0.098 | 0.95141 | 0.97428 |
For example, Figure 3 shows the deformations that occur in the walls of the filled tank due to the impulsive mode.
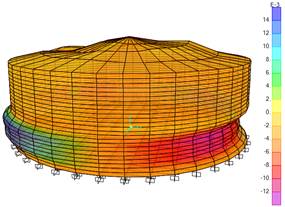
Fig. 3 Deformations in the walls of the filled tank as a result of the influence of the impulsive mode (mode 16, T=0.18s).
Because of its importance in the research, Figure 4 shows the distribution of stresses that occur in the tank walls, which are responsible for the different failure modes of the tanks under seismic actions.
IV. DISCUSSION
A. Vibration Period
When analyzing the values of periods produced as a consequence of the imposed seismic excitation, it is possible to observe a remarkable similarity between these values, which indicates a good accuracy between the methods used.
The dynamic analysis performed is based on the modal analysis of the structure; therefore, it is necessary to find, within a certain number of modes introduced, which are those that contribute the most to the modal participation of the masses, since the number of modes that contribute to the global response of the system is limited. Thus, it can be observed in Table 4 that, for the cases analyzed, the first mode of vibration matches with the period of the convective mode providing the highest mass participation, followed by mass contribution by high modes, indicating decoupling between the convective and impulsive modes.
Based on these results, it is possible to conclude that the convective and impulsive modes together account for more than 80 % of the total mass of the fluid, which shows that the response of the tanks is associated with the influence of the impulsive and convective modes of the fluid.
B. Resulting Forces
Table 6 shows a comparison between the values of resultant forces obtained based on the models analyzed according to the types of analysis performed, evidencing that the differences are less than 20 %. In this sense, previous research states that it is common to appreciate differences that oscillate between 30 and 35 % approximately [12].
C. Wall Stresses
The stresses obtained through the simplified dynamic analysis correspond to tangential tensile and axial compression stresses, determined in the lower part of the wall of the tanks under study, where their effects are considered more significant. Then, by performing a more complex analysis based on finite elements, it is possible to obtain a similar level of response by the applied analysis methods. Table 7 shows a comparison between the procedures applied, showing that the differences achieved are less than 25%, which are considered acceptable.
Table 7 Differences between the tension states.
Procedure | Filling | Shear | Difference | Moment | Difference |
---|---|---|---|---|---|
[%] | [KN/m²] | [%] | [KN/m²] | [%] | |
Simplified | 100 | 4523.77 | 21 | 118637.71 | 20 |
Finite elements | 5473.76 | 142365.25 | |||
Simplified | 75 | 2922.85 | 25 | 87378.51 | 20 |
Finite elements | 3653.56 | 104854.21 |
Based on the results obtained, it is possible to observe from Figure 5 that the maximum axial compressive stresses developed in the tank wall are concentrated at a short distance above the base plate (approximately 0.5 m) due to the constrained condition of the tank base. These high axial compressive stresses are consistent with the deformations known as "elephant's foot". On the other hand, the high values of tangential tensile stresses observed are responsible for the failure known as "diamond point".
V. CONCLUSIONS
From the results obtained, it is clear that i) the values of periods associated with fluid movement inside storage tanks are high; ii) the results obtained in terms of global response show some agreement between the analytical method specified in API. 650 and the computational models developed using finite elements. The percentage differences observed in these results can be considered acceptable since they show variations of less than 25%; iii) the models developed from the use of finite elements corroborate the existence of deformations in the lower part of the tank walls known as "elephant's foot" and "diamond point"; iv) when analyzing the tank under study with different aspect ratios (H/R), keeping the radius constant, the period of the convective mode is sensitive to decrease as the aspect ratio decreases becoming even, the convective mode is sensitive to decrease as the aspect ratio decreases; becoming at the same time, the impulsive mode less dominant.