I. INTRODUCTION
The tanning sector takes advantage of a residue from the meat industry, i.e., animal skin, and transform it into leather through different operations and unit processes; then, it is used to manufacture a wide variety of products. The consumption of chemical inputs, energy, and water by the leather tanning sector is considerable, as is the amount of solid and liquid waste [1]. The pollutant load of wastewater is extensive and contains high loads of organic and inorganic substances [2]. Some of the contaminants present in it are sulphides, ammonium salts, chlorides, dyes, chromium, and formic acid [3]. The high contaminant loads of this wastewater, as well as the diversity of substances present in it, have led to explore different treatment techniques to decontaminate them and carry out discharges with a lower environmental impact on the receiving body of water. Some of the techniques used to treat wastewater from the tanning sector are electrocoagulation [4], aerobic biological treatment [5], anaerobic biological treatment [6], clarification by coagulation, flocculation, and sedimentation [7], advanced oxidation processes [8], biomembrane reactors [9], and artificial wetlands [10].
The development of artificial wetlands as biological treatment systems to decontaminate wastewater not only is an excellent alternative to significantly reduce pollution levels in water bodies, but also an affordable alternative for these small industries, which do not have sufficient economic flow to acquire conventional wastewater treatment systems. In an artificial wetland, gravel, sand, soil, plants, and microorganisms present in the environment are used to decontaminate wastewater [11]. Constructed wetlands are mainly characterized by the presence of water on the surface - or below the surface of the support material or plant substrate - and by the direction of flow, horizontal or vertical [12]. A key aspect for the proper functioning of wetlands is the selection of plants, the difference between the ability to resist or adapt to residual water conditions influences the efficiency of the wetland in terms of contaminant removal [13]. In this work, an artificial wetland with horizontal and subsurface flow was analyzed at a laboratory scale for the wastewater treatment from the soaking process in tanneries. The main objective is to analyze the various effects of the plantation density of the species Limonium Perezzi on the removal of organic matter in terms of Chemical Oxygen Demand (COD), dissolved solids, and chlorides, which are the main contaminants present in that wastewater.
The salinity of wastewater produced in tanneries can hinder the correct development of plants that function as a means of phytoremediation. Halophytic plants can be used to solve this since they have salt glands, i.e., structures that store and secrete ions to regulate the salt content on the plant. These glands are epidermal and are found mainly on the leaves of plants that grow in coastal areas, saline soils, salt marshes, and mangroves; therefore, they can be considered specialized trichomes [14]. Salt moves from the xylem to the parenchyma in an apoplastic manner and then to the cells that support the salt glands, which are found at the base [14]. From these cells the salt moves easily through plasmodesmata to the storage cells where they accumulate in the vacuole. When the gland disintegrates, the salt is released, thus forming a white residue on the surface of the plant. Salt does not return to the plant from the gland because the cell walls are cutinized [15]. Functionally, these plants have two types of glands: those that secrete salt to the outside of the plant and those that store it in specialized cell vacuoles. Considering their organization, they are classified into four types: i) Saline vesicle (a large cell that accumulates salt); ii) multicellular (from 4 to 40 cells with collector and secretory cells covered with cuticle); iii) two-celled (threadlike structures with one basal and one apical cell); and iv) unicellular (vacuolar or filiform secretory cells) [16].
Limonium perezzi is selected for planting artificial wetlands because it is described as a halophytic plant, that is, a species adapted both morphologically and physiologically to grow in saline ecosystems. These plants capture salt and make it part of their metabolism; subsequently, they secrete it through buds and stems [17], which represents a significant advantage for their use in the soaking wastewater from tanneries. Moreover, this project aims to continue a previous study that analyzed the capacity of Limonium perezzi for tannery soaking process wastewater treatment. It should be noted that the use of artificial wetlands for wastewater treatment is related to the fulfillment of the Sustainable Development Goals, specifically 2, 3, 6, 12, 13, 14, and 15 [11].
II. METHODOLOGY
To build the wetlands, 0.95 m wide, 0.41 m long, and 0.3 m deep plastic containers were used; the surface area of each wetland was 0.3895 m2. Figure 1 is a representation of one of the wetlands used to conduct the project.
A total of 6 wetlands were used, three of them planted with 4 Limonium perezzi plants (HAP4) for a planting density of 10.27
; and three more planted with 6 plants (HAP6), 15.4
. At the time of planting, the plants’ height varied between 0.18 m and 0.25 m, they were weighed and the mass planted in each wetland was doublechecked to verify it was the same according to the number of plants.
The planting density was determined with the following equation:
Each wetland was filled as follows from bottom to surface: 0.05 m of stone, 0.05 m of gravel, 0.05 m of sand, and 0.1 m of soil. The average diameters of the stone, gravel, and sand material were 0.01 m, 0.0075 m, and 0.005 m, respectively. Each wetland was filled with water with peristaltic pumps, the feed flow rate to each wetland was 5.26 𝑚𝐿/𝑚𝑖𝑛 and the residual water outlet was at the bottom of each wetland using a siphon system to maintain a constant liquid height. Limonium perezzi plants were sown on the surface, soil was the support medium for the plants. The average porosity of the bed was 35%, this data was used to adjust the flow rate in such a way that the retention time in the wetlands was 4.5 days. The retention time in wetlands (τ) was determined as follows:
The wetlands were in a space with ventilation and natural light in Bogotá, Colombia (2630 masl; average temperature, 14ºC). The Limonium perezzi used in this study can be found in the Cundinamarca region and can survive between 1,000 and 2,620 masl.
Samples of influent and effluent water were taken every 1.5 days, the quality variables monitored were pH, COD
, TDS
and chlorides
. The analysis of each parameter was performed based on the procedures described in the Standard Methods for the Examination of Water and Wastewater [18]. Wastewater from the soaking stage was supplied by a tannery located in Chingacio village, municipality of Chocontá, Cundinamarca. The wetlands were identified as HAP4, the artificial wetland with 4 plants; and HAP6, the artificial wetland with 6 plants. The statistical analysis of data to determine whether there is a difference between the effectiveness of HAP4 and HAP6 was done through the Student’s T test (p<0.05) [19].
III. RESULTS AND DISCUSSION
This study allowed us to determine that the plants used in wetlands managed to survive for a period of 32 days, during this time, wastewater from soaking was treated and a maximum removal of COD and chlorides of 86.22% and 47.12%, respectively, were achieved [20].
The initial measured parameters of interest in the wastewater from the tanneries’ soaking process are presented in Table 1.
The Table 1 shows that the pH of wastewater is relatively alkaline due to the disinfecting agents, NaOH, and biocides used in the soaking process. The pH of the water was not modified to subject the wetlands to real conditions. Figure 2 corresponds to the variation of pH in the effluent water of the wetlands with respect to the time the tests were conducted.
Throughout the testing period, it is observed that the pH of water decreases, probably due to the neutralization of alkaline substances by the CO2 formed in the mineralization of organic matter in wastewater. This can be confirmed by the decrease in the concentration of COD. The pH is much lower in the HAP6 wetland, which suggests a greater decrease in the concentration of COD in it and agrees with the mentioned above. Figure 3 shows the variation of the COD concentration at the wetlands outlet and its removal percentage throughout the test time.
Approximately after day 14, the concentration of COD and the removal percentage do not present significant variations; this indicates that the plants and microorganisms adhered to the fill material of the wetland have adapted to the substrate contained in the affluent. That is, the maximum decrease in COD concentration has been reached, as can be seen in Figure 3. In the HAP4 wetland, small variations of the concentration can be noted in the mentioned period, this may be due to the lower number of plants in it and the affectation of salinity that deteriorates the planted species. It can also be noted that the stabilization period of the operating conditions of the wetland is relatively short. The rate of decline of COD in the HAP6 is considerably higher than in the HAP4, and the stabilization time for this wetland occurs approximately after day 10 of operation. This is attributed to the greater number of plants in the HAP6. The analysis of the kinetic trend of the COD concentration data against time, presented in Figure 3, acceptably fits a first-order model with an R2 of 0.981, and a slope corresponding to the kinetic coefficient equal to 0.2227 days-1. Equation 3 allows calculating the wetland effluent COD with respect to retention time.
where t is the water retention time in the wetland in days. Figure 4 represents the average chloride concentration data
with respect to the operation time of the wetland for the two types used in the tests.
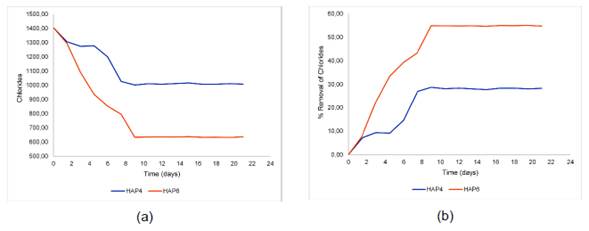
Fig. 4 Variation of chloride concentration in the wetland effluent. (a) Chlorides, (b) Percentage of chloride removal.
The difference between the concentration of chlorides in the effluents of the wetlands is much more noticeable than in the case of COD. Moreover, the stabilization time of the chloride concentration in the effluent of each wetland is close to 9 days. From that moment on, their variation is minimal, which confirms that the plants have adapted to the saline environment they are subjected to, and this may be the reason why the removal of COD reaches its stability after plants adapt to the chloride concentration in water. From day 10, the presence of crystals was observed on the surface of the leaves and stems of the plants in the HAP4 wetland, and from day 14 in the plants from the HAP6. The difference in the secretion times of NaCl crystals can be due to the difference in the number of plants in each wetland, i.e., the distribution of the chloride load in the plants. As for COD, the kinetic trend of chloride concentration in the wetland effluent was analyzed, in this case R2 reached a value of 0.985 for a first order kinetic model, the kinetic coefficient presented a value of 0.0397 days-1. Equation 4 corresponds to the concentration of chlorides in the wetland effluent with respect to the retention time.
where t is the retention time in the wetland in days-1. The concentration of TDS in the water was measured to have an additional parameter to analyze the removal or retention of salinity by the plants. Figure 5 shows the average results of the TDS concentration in the wetland effluent.
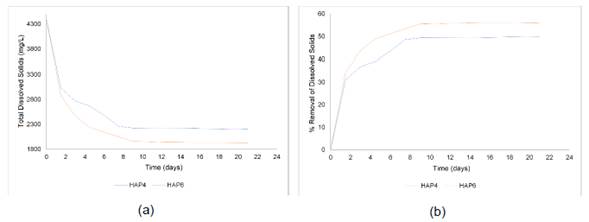
Fig. 5 Variation of TDS concentration in the wetland effluent. (a) TDS, (b) percentage of TDS removal.
As with the previously analyzed variables, the HAP6 wetland allows reaching a lower TDS concentration value in the effluent with respect to the HAP4. This is attributed to the planting density, i.e., the retention in each wetland is higher due to the greater number of plants, the load of chlorides, and other solids dissolved in the water. It is also observed that TDS presents a greater decrease in terms of concentration, this is because halophytic plants retain chlorides and sodium, which are then expelled as NaCl crystals. Figure 5 shows that the removal of TDS in the HAP6 wetland exceeds 50%, and that the stability in the concentration of TDS in the effluent follows the same trend that the chloride concentration.
Figure 6 contains photographs of the HAP4 wetland. It is possible to observe the change in the appearance of the plants with respect to the wetland’s operation time.
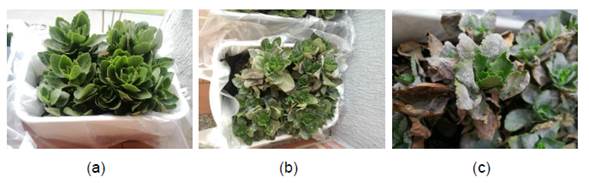
Fig. 6 HAP4 photographic record. (a) onset, (b) after 4 weeks of operation, (c) after 8 weeks of operation.
Figure 6 (a) shows the state of the plants on the day the wetland operation begins and the natural green color of the leaves and stems. After 4 weeks of operation (b) discoloration in some of the leaves and stems and NaCl crystals on their surfaces can be observed. In (c), it is noted that there are still leaves in good condition, but the amount of discolored and dry leaves on the plant is higher, as well as the amount of NaCl crystals on the surface of the leaves and stem. A possible cause of the discoloration of the leaves can be the lack of nitrogen; in this case, the nitrogen for the sustenance of the plants is found in the soil supporting the plantation and in the wastewater that contains animal blood; thus, it contains proteins that can provide nitrogen for plants survival. The deterioration of plants may be due to the competition of Na+ and Cl- ions with nutrients such as K+, Ca2+, and NO3- [21]. In other words, increased salinity in water increases osmotic pressure, reduces nutrient uptake, and can expose plant tissues to toxic ions [22].
Figure 7 shows photographs of the HAP6 wetland at various system operation times.
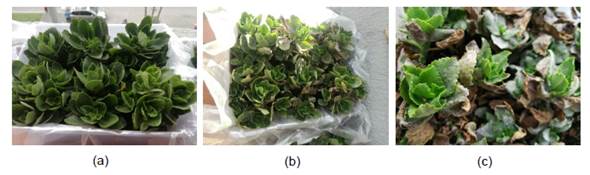
Fig. 7 HAP6 photographic record. (a) onset, (b) after 4 weeks of operation, (c) after 8 weeks of operation.
As for the HAP6 wetland, Figure 7 (a) shows the state of the plants at the onset of the process, and illustration 6 (b) after 4 weeks of operation. It is noticeable that some of the leaves of the plants present discoloration and NaCl crystals on the surface after 8 weeks of operation, the concentration of the effluent after 4 weeks is already in a stable state. In Figure 7 (c), the number of discolored leaves and the amount of NaCl crystals on the leaf surface is higher, but the portion of affected plants is smaller than the HAP4 wetland.
To determine if there is a difference in the performance of the wetlands in terms of the concentration of pollutants in the effluent, the comparison of means - t test (p<0.05) [12] - was conducted with the results obtained in the HAP4 and HAP6 wetlands. Figure 8 presents the results of the comparison test for the comparison among the average concentrations of COD, chlorides, and TDS in the effluent of each wetland.
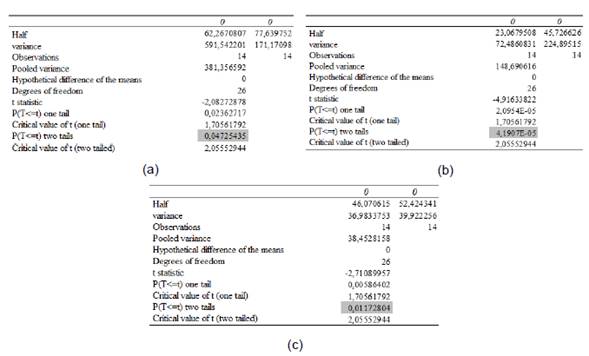
Fig. 8 Student’s T test results for comparison of average concentrations in the wetland effluent. (a) COD, (b) chlorides, and (c) TDS.
According to the test results, the p value was in all cases lower than 0.05. The results of the T test show significant differences between the concentrations of the parameters analyzed in the effluent of the HA4P and HA6P wetlands, with a confidence level of 95%. This difference can be explained because increasing the number of plants also increases the consumption of organic matter, absorption of chlorides, and dissolved solids by the plant material. Additionally, a greater number of halophytic plants distributes the pollutant load and the toxic effect on plant tissues caused by toxic ions and reduces the dose in each plant in the wetland.
Hence, by increasing the plantation density, it is possible to increase the removal of the parameters of interest and extend the life time of the plants due to the greater distribution of doses of contaminants in this species, as shown in the illustrations. It is necessary to determine the maximum planting density to ensure the removal of contaminants and the life of the plants. It is convenient to explore alternatives that allow automated removal of NaCl crystals from the surface of leaves and stems and prevent them from falling into the water, in this way NaCl can be recovered to be used in other activities, such as pickling prior to tanning. It is also convenient to determine the effect of removing NaCl crystals on the functionality and survival of plants and thus the integrity and functionality of the wetland.
IV. CONCLUSIONS
Artificial wetlands planted with Limonium perezzi enable reducing the concentration of COD, chlorides, and TDS in wastewater from the soaking stage in tanneries. It was confirmed that the Limonium perezzi species can develop in saline environments and meet the objective of decontaminating water with a high concentration of chlorides and TDS.
The results and the statistical analysis show significant differences between the concentrations of the effluent of the wetlands when the planting density varies. In other words, increasing the number of plants increases the purifying capacity of the wetland. Additionally, the plant survival becomes higher due to the distribution of the salt load in a greater number of plants.
The hydraulic retention time in each wetland was 4.5 days and the stabilization time of was approximately 14 days, this suggests that the adaptation capacity of the planted species is high. Moreover, the deterioration of the plants is observed from week 4 of operation, but the system continues in normal operation and in stable conditions in terms of concentration of pollutants in the effluent. It is convenient to have banks of Limonium perezzi plants to replace the ones that perish.
To confirm that planting density affects the affectivity of wetlands, it is necessary to explore what is the maximum planting density that can be implemented so that the plants can reach their development and avoid competition with the others present in the wetland.
The use of artificial wetlands planted with a halophytic species such as Limonium perezzi constitutes a treatment alternative that allows water to be decontaminated and simultaneously contributes to Sustainable Development Goals 2, 3, 6, 12, 13, 14, and 15 in aspects such as health and well-being, the guarantee of clean and healthy water, responsible production and consumption, and the guarantee of a healthy environment. Additional research is required to determine variables such as the maximum salinity that the plants can withstand without significantly affecting the effectiveness of the wetlands and the integrity of the plants, the optimal hydraulic retention time, the nutrient retention capacity, and the characteristics of the community of microorganisms that develop in the fill material of each wetland. It is also convenient to study the effect of inoculating microorganisms tolerant to high concentrations of salinity and to evaluate the effect on the wetlands performance.