I. INTRODUCTION
The fifth generation of mobile networks (5G) emerges as a solution that allows meeting the requirements of today's technological society. 5G NR is the global standard for the unified 5G wireless interface and link layer protocols [1]. This standard is developed by the 3rd Generation Partnership Project (3GPP) and seeks to establish the guidelines, criteria, and regulations around this technology. The main characteristic of 5G is that it can support a wide variety of services simultaneously, thanks to the fact that it incorporates several scalable numerologies ((), unlike Long Term Evolution (LTE) networks, where there is only one [2]. This feature is fundamental to allow a massive growth of the network in the number of connected devices, the volume of data traffic and the capability to offer a very diverse number of services with different types of requirements. These services are classified in 5G NR into three groups: Enhanced Mobile Broadband (eMBB), which requires very high data rates and large bandwidths; Ultra Reliable Low Latency Communications (uRLLC), which requires very low latency and very high reliability and availability; and Massive Machine-Type Communications (mMTC), which require low bandwidth, multiple access points, enhanced coverage, and low power consumption at the user end [3]. The flexibility and scalability of 5G to deal with multiple services should ensure the adaptation of such mobile networks as needed. Consequently, and to solve this condition, the concept of Network Slicing or network segmentation arises.
Network segmentation is a 5G NR feature that allows the management of different requirements such as available bandwidth, transport network, or number of access points demanded by the wide range of services in a common network infrastructure. The allocation of these network segments is done flexibly to support the variety of services and management of the variable traffic loads of the transport network [4].
Designing network architectures and transport systems that meet the requirements of the previously mentioned technologies is a challenge. Therefore, adequate components and infrastructure must be used to allow the network to operate effectively. In this context, AWGs that have been widely used in long-haul transport networks to perform optical channel multiplexing and demultiplexing, may perform certain complex functionalities that 5G networks require in the front-haul segment [5].
Previous works have shown the flexibility that multiple numerologies provide to 5G networks, compared to LTE, due to the use of numerologies [6]. Also, [7] presents the performance of NR for different scenarios of the mobile network based on the numerologies’ parameters established by 3GPP. In [8], the segmentation analysis for Radio Access Networks (RAN) in the physical layer is performed to relate the multiple numerologies with the network slicing. On the other hand, a comprehensive description of the 3GPP standards for network segmentation and the identification of key issues that must be addressed in the future for the development of the network division is presented in [9]. The use of AWGs in the fronthaul segment to evaluate and improve the filtering performance of 5G networks is presented in [10]. The design of a fronthaul transport network based on WDM with its corresponding technical study covering different topologies and WDM equipment that can be used when designing a fronthaul network is proposed in [11]. An experimental study on polarization-multiplexed transmission of a radio over fiber system for next-generation mobile fronthaul is presented in [12]. And a hybrid microwave photonic link based on a polarization division multiplexing Mach-Zehnder modulator for the co-transmission of millimeter-wave (mmW) and sub-6 GHz wireless signals over a seamless single-mode fiber (SMF) and free-space optics (FSO) channels [13].
This paper presents the design of an optical transport system for the fronthaul segment that deals with different numerologies and network slices in a 5G NR network. The proposed architecture is evaluated by software modeling using Optisystem software. The proposal consists of two key devices: a PS, for network slicing, that enables the transport of different service profiles by exploiting the polarization multiplexing dimension, and an AWG that allows the distribution of the numerologies for each polarization multiplexed stream within the fronthaul segment. To the best of our knowledge, it is the first time that two domains of multiplexing, i.e., polarization and wavelength, are used to enable the parallel transport of different services and distribution of different numerologies in a 5G fronthaul network.
II. METHODOLOGY
Figure 1 shows the proposed architecture. The Polarization Splitter and the Polarization Combiner enable the simultaneous transmission of two sources of information based on OFDM onto the same wavelength channel, i.e., two different network slices transmitted by splitting into two orthogonal components the optical carrier emitted by the laser source. Such polarized components were used to feed the optical modulator. Subsequently, the AWG enables the allocation and distribution of numerologies for each one of the slices. Insets (a) and (b) in Figure 1 show the way the distribution can be performed in the AWG depending on the wavelength channel and the Free Spectral Range (FSR). As seen in inset (a), each AWG output port distributes a single numerology, or in inset (b), where multiple numerologies can be allocated at each port.
The expression of the FSR in terms of the AWG parameters was calculated using Equation (1).
Where N is the number of ports of the AWG at the output end, and ∆fs is the frequency spacing between the carriers.
Numerologies are related to the subcarrier spacing (SCS) of the OFDM transmission. Thus, the configuration of the OFDM modulator and demodulator varies according to the numerology, as described in Table 1. In particular, 5G NR supports numerologies (=0, 1, 2, 3, and 4, where SCS is given by Δfµ = 2µ ●15(kHz) . In this sense, we assessed the system performance for the 5G numerologies that correspond to (=0, 1, 2, and 3, which correspond to SCS of 15 kHz, 30 kHz, 60 kHz, and 120 kHz, respectively.
Table 1 OFDM configuration for each numerology.
( | Δf µ = 2µ ● 15(kHz) | Number of subcarriers | Number of FFT points |
---|---|---|---|
0 | 15 | 3333 | 4000 |
1 | 30 | 1666 | 2000 |
2 | 60 | 833 | 1000 |
3 | 120 | 416 | 500 |
The radio frequency carrier used for the OFDM configuration was 6 GHz. As the subcarrier separation parameter cannot be configured directly on the OFDM modules of the simulator, Equation (2) was used to set up the SCS in the software.
Where ∆f is the subcarrier spacing, the Bit rate is the transmission rate, M is the number of symbols, in this case, 4, since the transmission is 4QAM, and N SC is the number of subcarriers. From this expression, N SC is used as one of the parameters available in the OFDM module, along with the number of points of the FFT to configure the SCS, as seen in Figure 2. Next, in order to distribute the services to multiple users, the system must support point-to-multipoint operation. In such a context, a power divider is placed in port 1 of the AWG. Thus, simulations were performed modifying the splitting ratio (1:n) of the power divider while increasing n by 2 k with k = 1, 2, 3... until determining the number of users according to the criteria to meet the signal quality requirements depending on the link length and the power of the optical transmitter. Our proposal evaluated the link length from 5 km up to 80 km, and the optical power was 15 dBm.
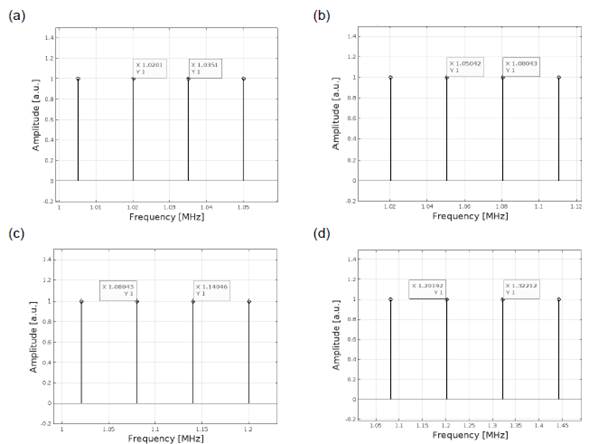
Fig. 2 FFT of the OFDM signal transmitted: (a) SCS 15 kHz, (b) SCS 30 kHz, (c) SCS 60 kHz, (d) SCS 120 kHz.
It is important to note that this system is scalable depending on the available AWG ports, i.e., the number of end users is Mxn, where M corresponds to the AWG size and n is the splitting ratio of the power divider connected to each AWG output port. Then, the modulated signals are recovered by means of a polarization divider at the receiver-end after the transmission link in standard single-mode fiber operating at 1550 nm.
Regarding the fact that the SMF does not maintain polarization, a key aspect deals with the configuration of the Polarization Splitters and Combiners in the simulation setup. In this context, the angle of such modules must be the same in order to keep the polarization states in the transmitter and the receiver aligned.
In our proposal, based on the characterization of the Polarization Combiners and Splitters, an angle at 45º assured an even power distribution between orthogonal outputs, i.e., a Polarization Extinction Ratio of 0 dB that avoids churning or interference between signals. Subsequently, the rotation of the received constellations was rectified by a script in Matlab in order to perform the quality measurements. Also, the OFDM demodulator in Figure 1, in addition to including the QAM demodulator and the OFDM demapper, includes a PIN photodiode whose configuration comprises a responsivity of 0.7 and a thermal noise of 1x10-22 W/Hz. These values correspond with a regular PIN photodiode operating at 1550 nm.
Regarding the quality measurements, while the BER accounts for the number of bits that were not correctly received within a bit stream, the EVM is a quality measurement for constellation diagrams, in which it is possible to quantify the percentage of the difference between the sent symbols and the received symbols. The expression to find the EVM in percentage is given by Equation (3) [14].
Where X i,k is the k-th ideal symbol of the constellation, X m,k is the k-th measured symbol, and N is the number of symbols of the constellation.
III. RESULTS
The distribution of numerologies in the AWG based on the wavelength channel and the FSR, following Eq. 1, is shown in Figure 3. In our proposal, as the frequency spacing between carriers in the AWG is
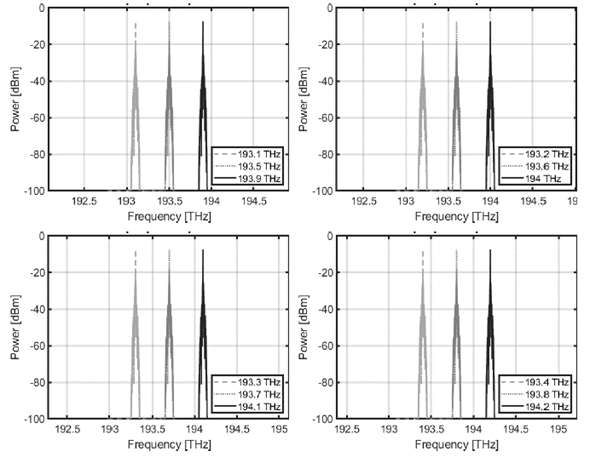
Fig. 3 Simulation results: Distribution of numerologies through the AWG based on the wavelength channel and FSR in (a) Port 1, (b) Port 2, (c) Port 3, (d) Port 4.
Figure 4 shows the results of the BER measurements for the proposed approach. In particular, Figure 4(a) represents the BER measurements corresponding to service profile_1, odd outputs of the polarization splitters at the receiver-end in Figure 1, BER 2N+1, with N = 0, 1, 2, ..., n. The results show that the performance is similar for 16, 32, and 64 users. For 128 users, it is found that the system has a good performance up to approximately 50 km.
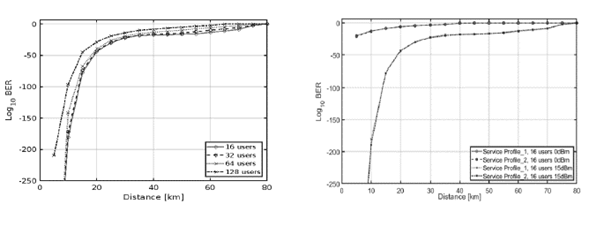
Fig. 4 Simulations results: (a) BER performance as a function of the distance and number of end-users. (b) BER performance as a function of the distance and transmission power.
According to what was found, if n=128, the number of end users corresponds to 128M, the maximum number of end users could be 12,288 as there is commercial availability of AWGs with up to 1×96 ports [15]. In addition, Figure 4(b) shows a comparison of the results with the transmitters operating at 0 dBm and 15 dBm with the common splitting ratio of 1:16.
These results are important from the network-dimensioning point of view, which indicates that similar results were found for both network slices, i.e., service profile_1 at the odd output ports and service profile_2 at the even output ports. However, the performance on both slices notably may be jeopardized by reducing the optical transmission power or incrementing the optical losses in the network. This later can be originated by deploying longer links or increasing the splitting ratio beyond 1:128.
Similarly, Figure 5 shows the results of the EVM measurements for each numerology and service profile. The results of our proposal are contrasted against the back-to-back signal, i.e., the signal obtained in a common point-to-point architecture. Considering the standards defined by 3GPP and ETSI, the minimum EVM requirement for a QPSK or 4QAM transmission is 17.5% [16]. In this way, as can be seen in the aforementioned table, the EVM values comply with the acceptance limit, particularly for (=0, 1 and 2. In addition, it can be noted that there are no significant differences in performance between network slices.
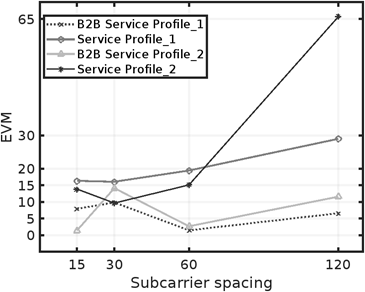
Fig. 5 Simulations results: EVM performance as a function of the subcarrier spacing for two service profiles.
The changing behavior of the EVM values as a function of the subcarrier spacing is due to different signal group delays caused by the chromatic dispersion of the fiber and the direct detection process performed in the photodiode. These two aspects mean that the different frequency components of the electrical field after the photodiode do not add themselves coherently to form an electrical signal that reproduces the optical signal present at the input of the photodiode, but generates distortions that affect the OFDM-based signal in an unalike way instead.
The constellations corresponding to the EVM measurement shown in Figure 5 are depicted in Figure 6. The upper row represents the constellations for the back-to-back signal for each numerology, and the lower row shows the constellations at the receiver-end in the point-to-multipoint architecture.
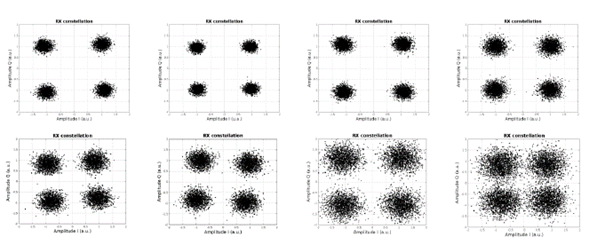
Fig. 6 Simulation results: Constellation diagram for the corresponding numerologies. Upper row: Back-to-back measurement. Lower row: Constellation at the receiver-end.
Higher symbol dispersion can be observed in the point-to-point topology and, therefore, a greater EVM, as shown in Figure 5. For the SCS numerologies of 15 kHz, 30 kHz, and 60 kHz, it is found that the EVM meets the requirements, in contrast to the SCS of 120 kHz, which does not assure the transmission quality. Such a situation may be a consequence of using a fixed radio frequency carrier at 6 GHz since, for numerologies with a greater SCS, frequency carriers close to or greater than 20 GHz are suggested.
IV. DISCUSSION AND CONCLUSIONS
The presented work proposes an alternative transport system for the fronthaul segment in a 5G network that exploits the polarization and wavelength domains to enable the transport and allocation of multiple numerologies and service profiles. This system allows the flexible management of the numerologies corresponding to subcarriers spacing of 15 kHz, 30 kHz, 60 kHz, and 120 kHz, transmitting two service profiles simultaneously per user for 128M end-users, scalable according to the M AWG ports. The response of the AWG performing a cyclical operation enables the numerologies to be distributed with a periodic response according to the FSR and depending on the input port and frequency.
It was found that the quality of the transmitted signal, measured with the BER indicator, decreases as a function of distance and that the greater the number of users, the lesser the link reach. The maximum range obtained with an optical transmitter operating at 0 dBm was 20 km for 16 users, whereas with a 15 dBm transmitter, the reach was 50 km for 128 users on a single port. Therefore, it is necessary to point out that the dimensioning of the system depends on the design considerations of the network in terms of these variables, number of end-users, and maximum reach.
The metrics used to assess the proposal were the bit error rate (BER) and the error magnitude vector (EVM). The results show that the greater the number of users, the lesser the link reach, with 12,288 being the maximum number of end-users found for the architecture. In addition, the EVM is lower than 17.5% for SCS 15 kHz, 30 kHz, and 60 kHz, fulfilling the minimum requirement established for a 4QAM transmission.