1. Introduction
Drinking water scarcity is one of the major threats to world sustainability. Efficient use of water resources together with technological approaches are required to address the potable water threat faced by an increasing population. In this context, the demand for water desalination, deionization, and softening technologies is growing. Technologies such as solar desalination, distillation, reverse osmosis (RO) and other membrane- based technologies are available. RO is now very well established and has become the benchmark for novel desalination strategies. There is, of course, plenty of room for flexible technologies that can be used for small or large scales 1.
Desalination and deionization technologies based on electrochemical cells have been developed for a wide variety of applications because of their relatively low energy consumption 2,3. In this category, electrodialysis 4 is the one technology that has reached commercial success. In turn, capacitive deionization (CDI), that has been widely researched in the past two decades, is trying to make the transition from the laboratory bench to the market 5. Finally, ion concentration polarization (ICP) is a more recent, promising technology 6.
CDI is a technology that removes ions from water as opposed to RO, which removes water from salty solutions. In a CDI electrochemical cell, saltwater flows between a cathode and an anode held at a potential difference sufficiently high to attract and adsorb cations and anions, respectively. The electrodes are typically carbon based. Once the two electrodes are saturated with ions, the polarity of the cell is reversed to release the adsorbed ions into a drain stream. In more recent developments, the CDI electrodes are coated with deionization membranes to overcome efficiency issues and sluggish kinetics. In this regard, conducting polymers, such as polypyrrole (PPy) and polyaniline (PANI), have been used as membranes for individual electrodes in CDI 7-9.
Conducting polymers are organic polymeric materials that can be reversibly switched from a neutral (electrical insulator) to an oxidized (electronically conducting) state by chemical or electrochemical oxidation and reduction 10,11. The electronic transitions are accompanied by changes in ion transport, optical, and wetting properties. The transition is accompanied by a flux of ions and solvent in and out the polymer. In the past 30 years, a lot of knowledge has been accumulated on the mechanical, electronic, optical, and other properties 12.
Charge transfer and transport in conducting polymers has been amply studied 13,14. Ion exchange properties are largely determined by the nature of the electrolyte used for electrosynthesis. Thus, when a conducting polymer (for instance, PPy) is prepared as an oxidized film by anodic deposition in solutions of small electrolytes (typically, NaCl, KNO3 or LiClO4), anions enter the polymer to compensate for the positive charges developed along the oxidized polymer backbone. Equation (1) represents the anion (A-) exchange behavior in PPy as the polymer film is switched between its insulator (reduced) and its electrically conducting (oxidized) form.
PPy
‒ne- +nA- (from electrolyte)
⇆ ⬚ PPyn+(A-)n
“Reduced” form
“Oxidized” form
It is also known that electro synthesis in the presence of large anions (for instance, anionic surfactants and polyelectrolytes) induces cation rather than anion exchanging properties in the polymer films as schematically shown in equation (2) using sodium polystyrene sulfonate (PSS) as an example of an anionic polyelectrolyte.
PPy/PSSn-(Na+)n
‒ne-
⇆ ⬚ PPyn-(PSSn-)
+Na+
“Reduced” form
“Oxidized” form
By combining (1) and the reverse of (2) as the anodic and cathodic reactions, respectively, one obtains (3), which is the basis for an electrochemical deionization cell
PPy + PPyn+(PSSAn-)
+nNa+ + nA-
⇆ ⬚ PPyn+(A-)n + PPy/PSSn-(Na+)n
Once this reaction is complete, the fully charged polymer electrodes can be discharged by switching the cell polarity. In 1988, Shimidzu and coworkers used the concept described by equation (3) to build an electrochemical cell made from PPy and a composite from PPy and poly(vinyl sulfate) (PPy/PVS) 15. PPy and PPy/PVS were electrochemically synthesized on indium tin oxide electrodes. The electrochemical cell was used as a proof of concept to decrease the concentration of milimolar and submilimolar solutions of KCl by one order of magnitude.
Relatively thick films of PPy can be grown on several metal electrodes, including stainless steel and nickel. For this particular application, electrode materials with high surface-to-volume ratios, such as carbon cloth and carbon felt would allow for higher ion exchange capacity, operationally defined here as the ratio of ions (salt) exchanged to mass of dry, neutral polymer. These carbon electrodes are also useful because of their high over potential for the reduction and oxidation of water.
This paper refers to the fabrication of a reversible electrochemical desalination system based on carbon cloth electrodes coated with PPy (as the anode) and a PPy/PSS composite (as the cathode). PPy/PSS was used in this work because the mechanical and ion exchange properties of this composite have been amply studied. The cell was used for galvanostatic desalination of sodium chloride batch solutions in the 0.1 and 0.01 M regime. Monitoring of the evolution of the cell voltage as a function of electrolysis time (or charge passed) is useful to detect the end point of the desalination process. The ion exchange capacities of the polymers in the cell are comparable to those of conventional ion exchange resins. However, secondary reactions of the films degrade the electroactivity of these polymers after a few desalination cycles.
2. Experimental section
2.1 Reagents and Solvents
Pyrrole (Py, Aldrich) was purified in a neutral alumina column and stored under nitrogen in the dark, Poly(sodium-4-styrenesulfonate) (PSS, average molecular weight 70,000, Aldrich), lithium perchlorate (LiClO4, J. T. Baker), and sodium chloride (NaCl, J. T. Baker) were used as received).
Electrodes and Cells
All electrochemical experiments were performed with a PINE WaveDriver 10. Potentiostat/Galvanostat in home-made single- or double-compartment cells. For electroanalytical experiments (chronoamperometry and cyclic voltammetry), platinum disk electrodes (diameter = 0.5 mm) and stainless steel foils (area = 0.5 cm2) were used as working and auxiliary electrodes, respectively. Potentials were measured against either a saturated calomel electrode or an AgCl/Ag quasi-reference.
For bulk electrolysis (polymerization and desalination), electrodes were made out of graphite tape (square weave, 0.56 mm-thick, Johnson Matthey) and/or stainless steel (302 series). Figure 1 shows the schematics of a cell used for polymerization and deionization experiments. The electrodes and spacers fit in the grooves on the walls and on the bottom to adjust the separation between the electrodes and the volume of the cell at will. Graphite felt was placed between the electrodes to use the cell in the double-compartment mode. Typical inter electrode distances were 1 and 2 cm, for single- and double-compartment cells, respectively.
Polymerizations
Polypyrrole, PPy, was anodically synthesized at constant current in a two-compartment cell from 0.1 M pyrrole + 0.2 M LiClO4 in water or acetonitrile containing 1% water at 101( C. In turn, polypyrrole/poly(sodium-4-styrenesulfonate), PPy/PSS, was prepared from 0.1 M pyrrole and 0.3 M (in monomer) PSS in water at 101( C. The geometric area of the working electrode was 5 cm2. Typical applied polymerization current densities and charge were in the ranges from 2 to 10 mA/cm2 and from 100 to 2,000º C, respectively. A fresh polymerization solution (volume = 100 mL) was used for every 1,000º C polymerization charge.
Desalination
Before use for deionization experiments, the freshly prepared PPy and PPy/PSS polymer-modified electrodes were cleaned in deionized water in order to desorb residual, unbound PSS and oligomers of pyrrole. The desorption process was monitored by recording electronic spectra of the aqueous solutions in contact with the polymer-modified electrodes which shows characteristic features of PSS (bands at 228 and 265 nm) and soluble PPy oligomers (broad absorption bands in the 300-400 and in the 600-700 nm region).
The one-compartment desalination cells were made from PPy and PPy/PSS in 25 mL of NaCl or KCl solution. The deionization solution was stirred and the temperature kept at 22(1º C. The initial concentration of the electrolyte was varied in the range from 0.023 to 1.0 M. For the analysis of ion exchange capacity of individual polymers in desalination cells, the polymerization charge of the polymer at the counterelectrode was typically 50% higher than that of the working electrode. That is, the counter electrode contains an “excess” of electroactive material.
During desalination, concentrations of the electrolyte were measured by potentiometry. Typically, 0.1 mL of the electrolyte solution was diluted to make the test solution for analysis in the concentration range from 0.10 mM to 1.0 mM. The activities of chloride and sodium ions in the test solutions were then measured with Hanna HI4107 (50 uM to 1M) and FC300B (10uM to 1 M) ion-selective electrodes by the known addition method. Conductance experiments were done with a YSI 3417 probe (cell K = 1/cm) and a YSI 35 conductance meter.
Elemental Analysis
For these experiments, sample films of PPy and PPy/PSS were prepared on stainless steel under galvanostatic control by passing 1,000º C. Freshly prepared films were used to measure the composition of the films in their oxidized form. The samples of the films in their neutral (reduced) form were obtained by reducing freshly prepared films at -1.0 V vs Ag/AgCl. Both the oxidized and reduced films were rinsed with water and then dried in vacuum at room temperature for one day and then peeled off from the stainless steel electrodes for analysis. Elemental analysis was conducted with a Perkin Elmer 2400 CHNS/O Series II Elemental Analyzer.
3. Results and discussion
3.1 Electropolymerization of Monomers On Carbon Cloth Under Current Control
Figure 1 shows the cell voltage as a function of charge recorded during the anodic electro synthesis of PPy and PPy/PSS on carbon electrodes. Polymerization at a temperature close to the freezing point of the solvent is known to yield relatively uniform and highly conducting polymer films 16.
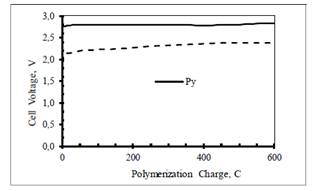
Figure 1 Anodic polymerization of 0.10 M pyrrole in water containing 0.32 M LiClO4 (continuous line) and 0.32 M PSS (dashed line) at carbon cloth electrodes (geometric area = 5 cm2) at 50 mA at 10º C in a double compartment cell. A 10 cm2 stainless steel sheet was used as the counter-electrode. The experiments were run under an Ar blanket.
The cell voltage increases sharply in the first few seconds and then it increases slowly until the end of the electrolysis, reflecting the increase of solution and film resistance with time. The voltage required for the polymerization of pyrrole in the presence of PSS is lower than that for the polymerization of pyrrole in the LiClO4 solution. This is consistent with the higher electronic conductivity of the oxidized form of PPy/PSS composite compared to that of PPy.
Table 1 shows elemental analysis of freshly prepared PPy and PPy/PSS films in their oxidized and neutral forms. For PPy, the small difference between the expected and experimentally found composition of the neutral PPy film is within experimental error. The much higher than expected experimental H/N molar ratio found for the oxidized PPy film is related to residual water. Oxidized PPy is known to be very hydrophilic. The Cl/N ratios reflect the amount of counterion present in the neutral (reduced) and oxidized films, indicating that the film can exchange ca. 0.28 mol of counterion per mol of monomeric pyrrole, in agreement with typically reported values (between 0.2 and 0.3).
Table 1 Composition of Free-Standing Films of PPy and PPy/PSS.
Films | Molar Composition Relative To Nitrogen | ||||||
---|---|---|---|---|---|---|---|
Expected | Experimental | ||||||
C | H | N | Cl | S | Na | ||
PPy | |||||||
Neutral | C4H3N | 4.06 | 3.35 | 1 | 0.04 | ||
Oxidized | C4H3N(ClO4)x | 4.11 | 4.70 | 1 | 0.28 | ||
PPy/PSS | |||||||
Neutral | C4H3N/(C8H7SO3Na)x | 1 | 0.00 | 0.25 | 0.24 | ||
Oxidized | C4H3N /((C8H7SO3)xNay | 1 | 0.00 | 0.24 | 0.01 |
For the PPy/PSS composites, the S/N ratio is a good indicator of the amount of PSS incorporated into the films and the differences in Na/S ratios between the oxidized and neutral films yield the amount of cation exchanged during redox cycling, ca. 0.24 mol of Na per pyrrole monomer. It is important to mention that the amount of PSS that is incorporated in the composite film depends on the concentrations of pyrrole and PSS present in the polymerization solution 16. Therefore, for this study, all of the polymers films were prepared under the same initial concentrations of monomer and doping ion.
3.1 Desalination Cells Made From PPy Anodes and PPy/PSS Cathodes
Curves of cell voltage as a function of charge, recorded during desalination of NaCl solutions at C/PPy anodes and C/PPy/PSS cathodes electrodes, reaction (3), are shown in Figure 2. Curves a, b, and c are titrations in 0.023, 0.11, and 0.1 M NaCl, respectively. After the initial steep rise, the voltage increases slowly as a consequence of the potential drop across the polymer-solution interface and the solution resistance. The subsequent sharp voltage increase indicates the end of the ion exchange reaction. For curves, a, b, and c, the first derivatives (dV/dC) show that the end of the reaction happens at 46, 61, and 72 C, respectively.
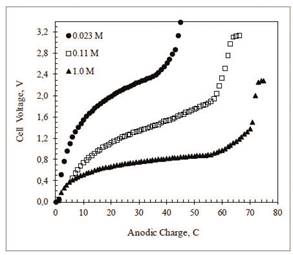
Figure 2 Evolution of cell voltage as a function of charge passed during desalination of NaCl aqueous solutions at C/PPy (anode) and C/PPy/PSS (cathode). Initial NaCl concentrations: 1.0 M (full circles), 0.11 M (empty squares), and 0.023 M (full triangles). Constant current = 0.25 mA. Electrode geometric area: 5.0 cm2. Cell volume = 25.0 mL. Experiments under an Ar blanket at 25º C.
The beginning of high voltage plateau, at the end of the curves, is consistent with the start of a new electrochemical process. Oxidation of chloride ions to chorine gas was readily detected at the anode. In addition, chlorine evolution is likely to induce irreversible overoxidation of the PPy films after the ion exchange reaction has finished. Overoxidation highly decreases the electronic conductivity of the film.
The charge used for ion exchange cannot be calculated from these curves because the total charge during the reaction has two major contributions, namely the faradaic reactions and the double layer charging which cannot be easily resolved 17,18. Better estimates of ion exchange capacities can be obtained by comparing the conductivity of the contacting solutions at the beginning and the end of the reaction. Thus, Table 2 shows the molar concentration of NaCl before and after the desalination experiments in Figure 1 as calculated by ionic conductivity and Na+ and Cl- ion activity measurements with ion selective electrodes.
Table 2 Desalination of NaCl Solutionsa.
Charge at rxn end point, C | Mass of dry PPy film, g | NaCl Concentration, M | meqCl- /g of PPy | ||
---|---|---|---|---|---|
Initial | Final | ||||
45.3 | 0.244 | 0.0231 | 0.0099 | 1.34 | |
61.6 | 0.238 | 0.1100 | 0.0920 | 1.56 | |
72.5 | 0.256 | 1.0000 | 0.9800 | 1.96 |
The ion exchange capacity of the desalination cell, expressed as the mili-equivalents of chloride ion exchanged per unit of mass of dry PPy film is very similar to those of commercially available ion exchange resins 19. The lower ion exchange capacity in the more diluted salt solutions can be improved by better cell design and by decreasing the current density in order to minimize the voltage drop across the cell.
Electrochemical cells as described in Figure 2.
3.2 Electrochemical Stability
The fully loaded polymers can be discharged by switching the cell polarity so the inverse of reaction (3) takes place. A very desirable feature of an electrochemical cell used for desalination is long term cyclability. Figure 3 shows the dependence of the ion exchange capacity on continuous electrochemical cycling in 0.10 M NaCl solutions as monitored by potentiometry with Cl- and Na+ ion selective electrodes.
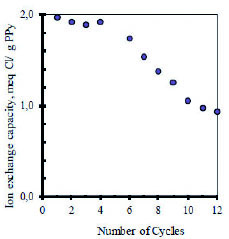
Figure 3 Ion exchange capacity (expressed as miliequivalents of chloride ion exchanged) of desalination cells as a function of electrochemical cycles. Initial concentration of NaCl: 0.11 M. Experiments at 25º C under an Ar blanket.
For the first five cycles, the system exchanges about 1.9 meq Cl- per g of dry PPy. After that, the ion exchange capacity decreases by nearly 50 % in another seven cycles. This is not necessarily surprising and it can be ascribed to degradation of the PPy based films by both generation of chlorine gas and over oxidation of PPy which is relatively unstable in air. Over oxidation of conducting polymers is known to cause interruption of the backbone conjugation by formation of hydroxyl and carbonyl groups, resulting in loss of electro activity 20,21.
Because of the low reusability of the PPy films, more stable polymers will have to be used in future studies. In principle, polyfuran (PF) and polythiophene (PT) derivatives might be attractive because they show electroactivity at higher potentials than that of PPy, and, therefore, they are more stable to degradation by oxygen and water. However, the electroactivity of PF and PT is poor in aqueous media because these polymers are more hydrophobic than PPy. Instead, copolymers of pyrrole, furan, and thiophene derivatives, for instance, alkylfurans and alkylthiophenes, might offer a better choice providing the resulting copolymers combine the hydrophilicity of PPy and the stability of PF and PT.
4. Conclusions
The electrochemical cell made from a PPy anode and a PPy/PSS cathode described in this work can substantially decrease the concentration of NaCl solution in the 10-1 and 10-2 M regime. The ion exchange capacities of the films in this cell are comparable to those of commercially available ion exchange resins. However, the electrochemical cyclability of the cell is poor because the PPy based films overoxidize. Copolymer films made from Py, furan, and thiophene derivatives may help to improve the long term stability of the cell.