Introduction
The World Health Organization (WHO) estimates that in Latin America 100 out of every 100,000 people are affected by at least one parasitic zoonosis (WHO, 2017). Although their incidence and prevalence are high, zoonotic parasitosis such as toxocariasis, is among the five most neglected diseases in the world (CDC, 2014); and is generally associated with the presence of animals in human environments (Marques et al., 2012) respectively. The aim of this study was to assess the environmental contamination by Toxocara spp. eggs and hookworms (Ancylostoma spp.). Infection with Toxocara spp. occurs through the ingestion of embryonated eggs of Toxocara canis and T. cati eliminated by infected dogs and cats through their feces. Once the eggs reach the environment, they can infect a significant number of people, especially children, which increases the importance in public health to this parasitic disease (Jones et al., 2008).
Companion animals represent potential reservoirs for Toxocara spp. to minimize the possible zoonotic transmission, the Companion Animal Parasite Council -CAPC- recommends deworming 15 day old puppies until six months of age with a broad-spectrum antiparasitic drug (CAPC, 2016). This recommendation is based on the epidemiological principle of a 100% probability of parasitosis due to Toxocara spp. in puppies due to the transplacental transmission of T. canis and the high level of environmental contamination by embryonated eggs (Lucio-Forster et al., 2016).
The global importance of parasitic diseases affecting humans and domestic animals, and the emergence of drug resistance (Köhler, 2001; Kopp et al., 2009; Bowman, 2012) promote the need for research to control parasitoses in companion animals and to reduce the exposure risks to humans and the development of parasitic zoonotic diseases. The development of a vaccine to control toxocariasis in dogs will play a fundamental role in the global management of this disease (Gasser, 2013) and would strengthen conventional anti-parasitic management schemes. This very much depends on the characteristics of such vaccine, like the duration and protection level, shelf-life, storage conditions, transport and the population to protect (Han, 2015).
Importance of toxocariasis in public health
From the epidemiological and public health perspectives, toxocariasis is an underestimated zoonosis, with difficult diagnosis, present in developing and developed countries (Torgerson and Budke, 2006; Lucio-Forster et al., 2016). It is a chronic disease composed of polymorphic clinical pictures, such as visceral larva migrans syndrome (Beaver, 1962), ocular larva migrans syndrome (Schantz et al., 1979), neurotoxocariasis (Finsterer and Auer, 2007) and covert (or asymptomatic) toxocariasis (Taylor et al., 1987; Taylor et al., 1988). The causal agents of this zoonosis are ascaridid nematodes from the Toxocara genus: T. canis and T. cati mainly infecting domestic and stray dogs and cats (Overgaauw, 1997).
Toxocara genus is composed of several species and have a broad spectrum of definitive hosts and they are ubiquitous in urban, periurban and rural areas worldwide. There are other Toxocara species that require epidemiological studies to determine their impact on public health because they could occasionally be sour-ces of infection for humans. The definitive hosts for T. vitolorum, are domestic and wild ruminants, which excrete larvae through milk, increasing the risk of infection, especially in rural areas of different countries where raw milk is ingested by people (Li et al., 2016). Another important species is T. pteropodis which is associated with bats and possible infection to domestic and synanthropic dogs (Prociv, 1989). It is believed that T. pteropodis is the causative agent of hepatitis-like disease in humans on Palm Island (Grenadines) (Moorhouse, 1982). In addition to these species T. tanuki, T. apodemi, T. lyncus, T. mackerrasae, T. paradoxura, T. sprenti and T. vajrasthirae, parasitize a variety of wildlife and synanthropic mammalians (such as bats and rodents, among others) (Gasser et al., 2006); in addition T. malaysense that has domestic and stray cats as definitive hosts (Le et al., 2016).
The female adult T. canis can oviposit up to 200,000 non-embryonated eggs daily which will be excreted through the feces of the host. These eggs develop to their infectious stage (embryonated eggs - containing stage 2 or 3 larvae, called L2-L3 infective larvae) in the environment (carpet, garden, soil, food) and/or in the fur of companion animals (Jones et al., 2008). The main risk factor associated with the development of human toxocariasis is to cohabit with companion animals (Wolfe and Wright, 2003), specifically puppies and kittens (Marmor et al., 1987; Lucio-forster et al., 2016). Puppies and kittens share interior resting areas with humans (bed, dining room, swimming pool, among others) (Scheibeck et al., 2011), creating a situation of great relevance in public health (Kollipara et al., 2016). Puppies can develop congenital parasitic infections due to the transplacental migration of these parasites while both, puppies and kittens, can be infected by ingestion of colostrum or breastmilk. These animals can become important disseminators of the nematode’s eggs when having large parasite loads in their intestine (Bowman, 2014). Humans and several animals are considered paratenic hosts since the parasites larvae do not develop to the adults, but migrate through somatic tissues (i.e. muscle, eye, Central Nervous System -CNS) (Figure 1) where they persist as an infectious stage L3 for extended periods. The presence of larvae in these tissues induces various pathological changes according to their migratory capacity through tissues and the immune responses of the host (Strube et al., 2013), both situations addressed below.
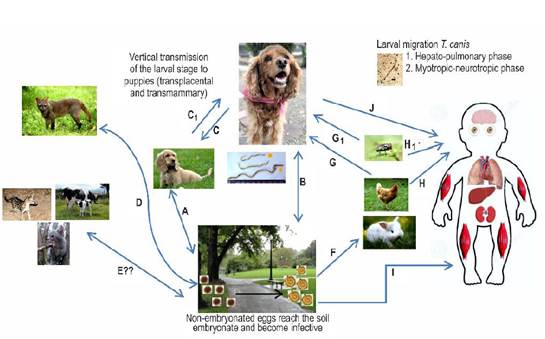
Figure 1 Biological cycle of Toxocara canis. A and B. Adult parasites are present in the intestine of domestic dogs (definitive host) which shed non-embryonated eggs through their feces. In the environment, they embryonate and become infective at temperatures of 25-30°C and a relative humidity of 85-95%. The development of the larval infective stage (L2-L3) within the egg requires 9-15 days. The main route of infection with embryonated eggs is the oral route. In the intestine, L2-L3 hatch and penetrate the intestinal wall, taking different actions related to the age of the infected dog. In younger dogs, L2-L3 migrate through the liver, kidneys, lungs and trachea, and then are swallowed to reach the small intestine. Once in the lumen of the small intestine, the larvae develop to the fourth (L4) and fifth (L5) larval stages. Finally, they reach the adult stage when after differentiation into male or female, for subsequent oviposition by the females (prepatent period 4-5 weeks). In dogs older than three months of age, after oral exposure and initiation of migration through the enterohepatic circulation, L2-L3 tends to encyst in various tissues (i.e. liver, skeletal muscle) where they enter a state of hypobiosis. C. In pregnant bitches during the last third of pregnancy, hypobiotic larvae are activated through hormone receptors associated with pregnancy (i.e. prolactin, progesterone), thus developing vertical infection (transplancental) or transmammary infection to neonates. C1. A highly infected puppy can excrete L2-L3 in the emetic content and the bitch can get infected when cleaning the vomited material from the puppies. D. The parasite can also complete their life stage and spreading to the environment through synanthropic and wild canids. These definitive hosts may directly acquire the infection by consuming of embryonated eggs from the environment (i.e. water sources) or predating previously infected paratenic hosts. E. Other unconventional hosts (i.e. wild and domestic feline species) may be associated with the life cycle of T. canis and its spread in the environment, but more research is required to determine the certainty of their active participation in this process. F. T. canis can be accidentally transmitted to other paratenic hosts (i.e. poultry, rabbits), which may ingest embryonated eggs from the environment. In these paratenic hosts the larvae migrate and form tissue cysts. G. Domestic, synanthropic or wild canids can prey on an infected paratenic host. In this case, the infective tissue encysted larva will complete its life cycle in the predator's small intestine. G1 and H1. Occasionally, the infection can be transmitted by passive vectors such as synanthropic flies. H. Humans are considered paratenic hosts and can become infected through the consumption of larvae from a paratenic host such as birds. I. The ingestion of embryonated eggs from the environment (e.g. geophagy). J. The dog’s fur can be contaminated with embryonated eggs acquired from the environment, being a possible source of infection for humans. After egg ingestion they behave similarly as in the other paratenic hosts with two determined larval migration phases: 1. Hepato-pulmonary phase, and 2. Myotropic-neurotropic phase. Encysted hypobiotic larvae will induce granuloma formation as a result of the host’s inflammatory response associated with the various toxocariasis syndromes.
The integral control of T. canis can be achieved by interrupting different stages of its life cycle (Figure 1). The main strategy is to deworm domestic dogs, giving special attention to pregnant bitches and puppies under 12 weeks of age. The implementation of massive deworming baits is useful to treat stray dogs. The challenge for the future is to establish vaccinations schemes to reduce and prevent the spread of eggs through feces into the environment (Hotez and Wilkins, 2009; Lee et al., 2014; Chen et al., 2018; Ma et al., 2018a).
The infection by T. canis is highly prevalent in the entire canine population that is not treated with anti-helmintics on a regular basis, and its presence in synanthropic and wild species makes its elimination almost impossible (Bowman, 2014). The high prevalence of toxocariasis can be explained by the hypobiosis of the parasite’s larval stage (L2-L3); larval viability has been found nine years after larvae have been encysted in non-human primate tissues. Furthermore, in the murine model, active migration has been identified after larval hypobiosis (Beaver, 1962). Concerning human toxocariasis, studies about its prevalence have been done in coun-tries of Africa, Asia, South America and specifically the United States, showing prevalence ranging from 5.1% up to 93% (Figure 2) (Buitrago and Gast-Galvis, 1965; Mendonça et al., 2012; Schoenardie et al., 2013; Macpherson, 2013; Cong et al., 2014; Moreira et al., 2014; Berrett et al., 2017; Sowemimo et al., 2017).
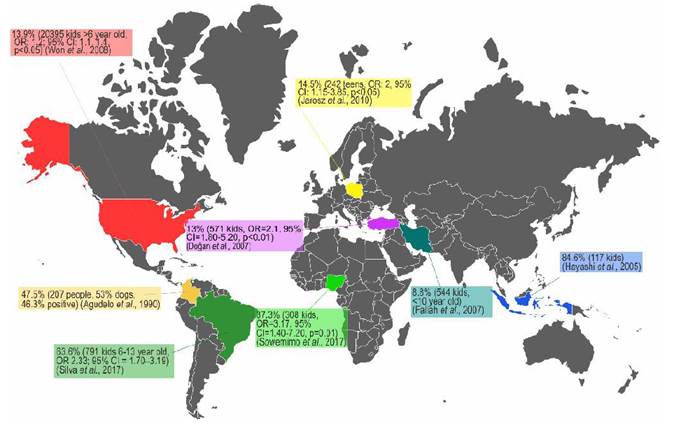
Figure 2 Several prevalence studies of T. canis infection in human population and epidemiological association with the presence of dogs. OR. Odds Ratio, CI. Confidence Interval.
Deworming domestic dogs is one of the main strategies to control world-wide toxocariasis, based on the fact that a significant number of families have dogs and they are a source of dissemination of viable T. canis eggs through their feces (Alcantara-Neves et. al., 1989). Furthermore they may have L2-L3 in their coat (fur) (Holland, 2017). Thus playing a preponderant role as a risk factor for exposure and infection with T. canis in humans (Regis et al., 2011; Strube et al., 2013; Sowemimo et al., 2017). The prevalence of this infection in the canine population is found in figure 3. The prevalence of canine toxocariasis ranges from 1.4% to 82.4% based on serodiagnosis and fecal exam. The real prevalence of canine toxorariasis is impossible to determine with the available information because most studies use different diagnostic methods, inclusion and excusion criteria; however, these studies are useful to show the epidemiological importance of canine toxocariasis in the world.
Moreover, other research has confirmed the importance of dogs in the dissemination of T. canis through their fur. In Ireland, Wolfe and Wright (2003) found that 15/60 dogs had viable eggs in their fur, where approximately 4.2% of these eggs were embryonated and 23.9% in the embryonation process. This study found 20 embryonated eggs/gram of dog hair. Roddie et al., (2008) examined the fur of 100 dogs, finding 67% of samples contaminated with viable T. canis eggs. Another study found 21.56% of viable eggs on the fur of 51 dogs, in which 21% of those viable eggs were embryonated or in embryonation process, with an average fur contamination of 8.45 embryonated eggs/gram of dog hair (Aydenizöz Ozkayhan et al., 2008). Furthermore, a recent study with a sample of 100 dogs, found that 14% of the samples were contaminated with viable eggs, with an average of 136 eggs per sample (Öge et al., 2014). In Brazil, Merigueti et al., (2017) reported a presence of 6.67% fur contaminated with T. canis eggs in a sample of 165 dogs, with an average of 12.2 embryonated eggs/gram of dog hair.
Most of T. canis infections are asymptomatic; even though, the parasite triggers the host’s immune response. Nematodes can exert the host’s immune responses to preserve its parasitic capacity. Shiny et al., (2011) reported high levels of regulatory/anti-inflammatory cytokines such as IL-10 and TGF-β between Wolbachia symbiotic phenomena with filariasis. Layland et al., (2013) found a significant recruitment of regulatory T cells CD4+Foxp3+ and the suppression of airway inflammation in a model of allergy in Schistosoma mansoni infected mice. Likewise Du et al., (2014 demonstrated that the excreted-secreted antigens from the Trichinella spiralis nematode inhibited the production of pro-inflammatory cytokines from classically activated macrophages (M1). The gastrointestinal nematode Trichuris muris can share epitopes of IFN-γ in the murine model, thus modulating chronic infectious processes by inducing changes in lymphoid cells (Grencis and Entwistle, 1997). In the same experimental model, it was shown that some secreted proteins of T. muris bind to Toll-like receptor 4 (TLR-4), activating MyD88 (essential part of middosome in the activation of inflamassome), downregulating Th2 responses and allowing chronic infection (Helmby and Grencis, 2003).
Specifically, for T. canis infection in children, a positive correlation was found between eosinophilia, IgE and sIgE levels (IgE specific for aeroallergens); a decreased cutaneous hypersensitivity for aeroallergens (Mendonça et al., 2012) and increased IL-10 production by blood cells (Alcantara-Neves et al., 2014). These conditions are the result of the interactions of T. canis and its hosts, altering the response to vaccination in children and predisposing to co-infections (Maizels and Mcsorley, 2016; Santos et al., 2017). Cooper et al., (2001) showed the potential modulation of immune responses by geohelminth like A. lumbricoides. After vaccination against cholera, the immune responses were effective in children that were treated with albendazol compared with children that received a placebo. On the other hand, significant interference has also been reported in antibody titration of puppies previously diagnosed with toxocariasis after receiving the rabies vaccine (Mojžišová et al., 2007).
Immune responses to infection with T. canis and creation of vaccines for the control of toxocariasis
Host immune responses to gastrointestinal helminth infection in general, cause a Th2-type immune response that in most cases make the host susceptible to reinfection (Hewitson et al., 2009). The acute inflammatory reaction is the result mainly of the innate immunity to the excreted-secreted antigens of the infective T. canis larvae (TES), which include more than 50 different macromolecules represented by a relatively simple set of glycoproteins, consisting of three gene families: 1) mucins (high molecular weight glycosylated proteins, between 120 and 40-45 kDa); 2) C-type lectins (sugar-bound proteins, whose molecular weights are around 70 and 32 kDa) (Maizels, 2006) and 3) other proteins that vary between 26 and 55 kDa (Maizels and Loukas, 2001). Mucins vary in number and volume (Loukas et al., 2000a) which are strongly glycosylated with galactose linked to O- and N-acetylgalactosamine groups (Meghji and Maizels, 1986). Apparently, this glycosylation capacity is related to the Th2 immune response and these molecules are specific targets for IgM (Schabussova et al., 2007). Furthermore, the larvae of T. canis periodically change their cuticle, releasing macromolecules to the host’s blood circulation, hindering the action of specific anti-Toxocara antibodies which do not affect the parasite. This biological action n is one of the forms of evasion of the host immune response and survival of this nematode inside their hosts’ bodies (Loukas et al., 2000b; Schabussova et al., 2007).
TES and somatic antigens, from the worm surface (hidden antigens) when used as vaccine, can bind to pattern recognition receptors (PRRs) such as TLRs and C-type lectin Receptors (CTLs) expressed on the cell membrane of enterocytes and other cells exposed to these TES and somatic antigens (SA) during migration and larval development (i.e. dendritic cells -DCs, macrophages) (Van Kooyk and Geijtenbeek, 2003). After binding with TLRs and CLRs, intracellularly, the middosome (MyD88, IRAK4 and IRAK2) activates the signatosome (IKKƔ, IKKα and IKKβ) and via nuclear factor NF-ĸB activates the inflammasome (e.i. NLRP3) and causes the production of the proinflammatory cytokines IL-1β and IL-18 (Gordon, 2002). There is also recruitment of various leukocytes such as neutrophils, monocytes, eosinophils, CD8+ T cells, basophils and DCs, the latter being in charge of antigen presentation and beginning of the adaptive immune response. Likewise, a Th2 type response characterized by the secretion of cytokines such as IL-4, IL-5 and IL-13 from CD4+ T cells and innate lymphoid cells (Smith et al., 2012). Particularly, IL-4 promotes the differentiation of B cells and antibody class-switching. In addition, IL-5 promotes the differentiation of eosinophils, and eosinophilia is a notable characteristic of Toxocara spp. infection (Beaver, 1962; Neill et al., 2010).
It is essential to establish integrated control guidelines (Magnaval et al., 2001), in which vaccination to control this parasitic disease in dogs would be the main action against the infection in several paratenic hosts including humans (Despommier, 2003; Gasser, 2013; Maizels, 2013). The development of vaccines for control and prevention of diseases caused by nematode has been restricted, and only some studies have shown positive results (González-Hernández et al., 2016). As example, the protection that can be induced with natural antigens derived from the intestine of Haemonchus contortus, an important gastrointestinal nematode affecting sheep and goats (Newton and Munn, 1999). However, other parasites present greater challenges for the identification of vaccine candidate proteins (Hewitson and Maizels, 2014). An important obstacle, even with successful natural antigens, has been the development of effective synthetic or recombinant vaccines. Gauci et al., (2008) successfully tested recombinant antigens identified in Taenia multiceps, a worm in sheep, where its oncosphere antigens associated with the QuilA® adjuvant significantly decreased the central nervous system parasite cysts. From immunoproteomic studies of Teladorsagia circumcincta, a gastrointestinal nematode of small ruminants, eight recombinant proteins were obtained and combined with the adjuvant QuilA®, getting 90% drop in fecal egg count for more than a year, and significant post-mortem reduction in the adult count in the gastrointestinal tract (Nisbet et al., 2013). These are some examples of success in the development of recombinant protein antigens for the control of gastrointestinal parasites. The limitation in the efficient development of vaccines against parasitic agents may be associated with the key roles that co-evolution and adaptation have played in the host-parasite relationship (Smith and Zarlenga, 2006). The different studies performed until now to evaluate vaccine proposals for the control of toxocariasis are summarized in Table 1, starting from the processes where embryonated parasite eggs exposed to ultraviolet radiation were used as the first approach to experimentation in dogs.
Reverse vaccinology and new generation adjuvants as a strategy for vaccine development in the control of T. canis infection
Advances in development computational software and discoveries of molecular functionality in modern biology have provided important opportunities to investigate epidemiological, diagnosis and even prophylaxy of Toxocara spp. (Ma et al., 2019). The clearest example of these applied cognitive development processes refers to genomic technologies of this parasite (Mardis, 2008, Ma et al., 2018b). The principle of reverse vaccinology starts from the genome sequence of the pathogen of interest and bioinformatics analysis, predicting those antigens that could be good candidates vaccine development, without the need to grow the specific organism to obtain natural antigens. The genome sequence provides a catalogue of virtually all proteic antigens that the pathogen eventually expresses. Furthermore, it is possible to generate new antigens (such as chimeric molecules), establishing new paradigms in immunodiagnosis or immunoprophylaxis for the infectious diseases control (Rappuoli, 2000; Mora et al., 2003; Sette and Rappuoli, 2010; Del Tordello et al., 2017).
There are several online databases for different important human and animal parasites (i.e. NEMBASE http://www.nematodes.org/nembase4, Nematode.net http://nematode.net, WormBase ParaSite http://parasite.wormbase.org/) that may be useful in the development of reverse vaccinology. In the case of T. canis the crucial point for the development of studies at this level is the completion of the T. canis genome project, in which Zhu et al., (2015) reported a genome size of 317 Mb. Recent studies led by Zhou et al., (2017) explored the details about the molecular biological processes of this nematode using high-performance transcriptomic sequencing of the 18,596 genes of the adult T. canis and bioinformatic analysis to explore aspects of reproduction and biological development of this parasite.Sperotto et al., (2017) developed the proteomic analysis of TES proteins using liquid chromatography-tandem mass spectrometry, identifying 19 proteins from the parasite’s genome. More detailed studies in proteomics developed by our research team (da Silva et al., 2018) have identified 582 proteins from larval extract and 64 proteins in TES. In this study we identified proteins that include immunomodulatory molecules involved in the evasion mechanisms and those that may be involved in pathogenicity. Some of these proteins have potential for the development of immunotherapy and immunodiagnosis. Based on these studies a series of proteins of immunological interest have been identified as vaccines candidates using reverse vaccinology. Some of these proteins include: A) TES-32 - a secreted protein that shows similarity with C-type lectins present in mammalian immune cells in the pathogen response process, molecular weight 32 KDa and composed of 219 amino acids (aa) (Maizels et al., 2000), B) TES-26 (Tc-PEB-1) - Phosphatidylethanolamine-bound protein, composed of 262 aa and a molecular weight of 26 KDa (Gems et al., 1995), C) TES-120 (Tc-MUC-3) - mucin-3 composed of 269 aa and a molecular weight of 45 KDa (Loukas et al., 2000a) and D) TES-70 (Tc-CTL-4) - C-type Lectin-4 composed of 288 aa and a molecular weight 70 KDa, identified as an important canine cell surface ligation protein (Loukas et al., 2000b). TES and somatic proteins from the worms’ surfaces are potential vaccine candidates because of their ability to generate a specific antibody response. However, their potency and efficiency of immune system stimulation is controversial (Soltys et al., 1996; Munn, 1997; Dvorožňáková et al., 2000; Dvorožňáko et al., 2002).
Vaccines are preparations used to stimulate humoral and cellular immunity against a specific pathogen, and are prepared using a harmless form such as the attenuated organisms or their recombinant proteins (Hewitson and Maizels, 2014; Han, 2015). To achieve its maximum immunogenic potential, it is strictly necessary to use adjuvants (Chan and Gack, 2016). Adjuvants exert their function by increasing the efficacy of antigens through the stimulation of the innate immune system, directly by stimulating DCs, macrophages and neutrophils, which lead to the activation of the adaptive immune system (Bonam et al., 2017). Nowadays, the use of adjuvants in vaccination seeks to direct the response of the adaptive immune system to the inoculated antigen (Reed et al., 2016), an action called “adjuvant effect”. This effect is the administration of an antigen with a specific microbial, among other compounds with biological activity, to enhance a specific immune response to the antigen. The microbial components of the adjuvants activate antigen presenting cells (APC) to produce pro-inflammatory cytokines and to upregulate the essential molecules for antigen presentation. An example of these molecules is the major histocompatibility complex (MHC) class II and B7-1/2 (CD80/CD86, co-stimulatory signaling of B lymphocytes and mononuclear phagocytes). This adjuvant effect allows a more effective antigen presentation, resulting in activation and clonal expansion of T cells (O’Hagan and Valiante, 2003).
There are several adjuvants that can provide greater potency and efficacy of T. canis TES and surface somatic antigens, such as imidazoquinolines (Th1 immune response adjuvants) which activate TLR7 and TLR8 (single stranded RNA receptors). After imidazoquinolines bind to these TLRs, transcription factors initiate transcription of multiple pro-inflammatory cytokines such as IL-17, which plays an important role in cellular immunity, particularly in infection-responsive immune response (Ma et al., 2010). Thus, after stimulation with this adjuvant there is a general increase in IL-17 producing thymocytes, which are relevant cells in the response to infectious pathogens and tumors (Cho and Celis, 2009). Allthough a classic adjuvant is aluminum hydroxide (Th2 immune response adjuvant), the mechanisms of its immunomodulation are still not completely elucidated. HogenEsch (2002) summarized the possible activities of aluminum salts as modulators of the immune responses by stimulating directly and indirectly dendritic cells and complement activation. Other studies have found induction of chemokine secretion (Ulanova et al., 2001). It has also been shown that aluminum salts develop inflammasome responses (e.g. NALP3) and IL-1β secretion (Eisenbarth et al., 2008). Recently, there are new adjuvants capable of stimulating a balanced Th1/Th2 responses, which is beneficial for immunoprophylaxis of helminthiasis (Diemert et al., 2018). One of these is the purified fraction of saponins (triterpenoid glucoside) extracted from the bark of the Quillaja saponaria, a Molina tree. In addition to its use as a surfactant, it is also used in a pseudo-ternary system with cholesterol and phospholipid to form colloidal structures known as ISCOM (immunostimulating complexes) (Kensil, 1996). These saponins generate a strong response to T cell dependent and non-dependent antigens (Petrovsky and Aguilar, 2004). They also induce cytotoxic CD8+ T cell proliferation and response (Newman et al., 1992) and enhance the response to mucosal antigens (Singh and O’Hagan, 2003). Another example of these new adjuvants is the AS01, which is composed of liposomes containing two immunostimulants: 3-O-deacyl-4’-monophosphoryl lipid A (MPL) and QS-21. MPL is a non-toxic LPS-derived compound from Salmonella minnesota and QS-21 is a saponin extracted from Q. saponaria (Didierlaurent et al., 2016). They act as agonists and synergistically bind to TLR4 inducing resident NK cells and CD8+ T cells to release IFN-γ into the regional lymph node, activate the macrophages and IL-12 and IL-18 secretion, hours after AS01 application (Marty-Roix et al., 2016 ).
Other adjuvants that may provide greater potency and efficacy of the T. canis TES and surface somatic antigens are the synthetic TLR1/TLR2 (Th1 immune response adjuvants) agonists such as triacylated lipopeptides which include Pam3CSK4, a molecule that mimics the acylated amino terminus of bacterial lipopeptides, and has the ability to bind to different receptors. TLR activating pro-inflammatory transcriptional factors such as NF-κB and modulating both cellular and humoral immune responses (Steinhagen et al., 2011). The immunogenic effect of Pam3CSK4 is underpinned by its ability to negatively regulates IL-13 and IL-10 responses (Pratti et al., 2016) and to lead Th1 immune response-based production of IFN-γ and TNF-α (Martínez-Orellana et al., 2017). In addition, Liu et al., (2013) showed that intravenous administrations of TLR-1/-2 ligands were able to activate the MyD88 signaling pathway and promote T cell differentiation through IL-12 secretion.
Final consideration
Finally, it is important to clarify the emerging role of domestic cats in toxocariasis, recent studies have shown that domestic cats are a significant source of T. cati eggs for the environment, having a possible preponderant role within this zoonosis (Lucio-Forster et al, 2016). From the epidemiological perspective, conventional diagnostic tests (serodiagnosis using anti-IgG-TES, parasitological tests in feces) do not differentiate infectious agents that cause toxocariasis in animals or people (de Savigny et al., 1979; Zhu et al., 1998, Alcântara -Neves et al., 2008) generating a dismissal of T. cati as a causative agent of human and animal clinical toxocariasis (Fisher, 2003). Although there are specific diagnostic, methods such as PCR using the second internal transcribed spacer (ITS-2) of the ribosomal DNA of T. canis, T. cati and Toxascaris leonina (Jacobs et al., 1997) they are used only in research. Other serodiagnostic tests such as ELISA , excretory-secretory antigen (ES Ag) from T. cati larvae for the diagnosis of human toxocariasis caused by T. cati (Petithory and Beddock, 1997) and Western blot using T. canis and T. cati ES Ag (Poulsen et al., 2015) have generated controversial results (i.e. cross reaction). It is possible that the cross reaction between T. canis and T. cati in seodiagnostics techniques is due to a) their somatic protein and TES homologues (Kennedy et al., 1987; Zahabiun et al., 2015); b) the low intraspecific variation between these helminths of different geographical regions (Zhu et al., 2000; Fogt-Wyrwas et al., 2013) and c) cross protection between T. cati and T. canis (Hosin and Al-Kubaysi, 2008). These actions could allow a single immunotherapeutic development to be able to control the two most important parasitic agents of this zoonosis in the world.
Conclusion
Toxocariasis is a disease that must be controlled in domestic and stray dogs and cats. This disease has a direct relationship with humans and has a potential zoonotic connotation. Among the control strategies, vaccination plays a major role by altering the parasitic cycle (i.e. vertical transmission: transplacental and transmammary infection) or significantly reducing the viability of eggs and their environmental concentration, consequently reducing the possibility of human infection. Given all the important advances in the molecular characterization of T. canis (transcriptomics, proteomics and genomics), and the identification of vaccine candidate proteins, reverse vaccinology is a potential strategy for the development of immunoprophylactic compounds. These studies would allow the generation of new vaccine antigens that can be enhanced by the use of the latest generation of adjuvants, thus creating one of the fundamental pillars in the integrated control of one of the most important neglected zoonotic parasitic disease that affects the poor human populations in the world.