INTRODUCTION
Alterations in the function and structure of the blood vessel wall account for most cardiovascular clinical events. Cardiovascular drugs may exert beneficial effects on the vascular wall both at the level of the endothelium and vascular smooth muscle cells. It has been recognized that endothelial dysfunction is an important early event in the development of various vascular diseases, including hypertension, diabetes, and atherosclerosis. This thin layer of cells that lines the interior surface of all blood vessels, modulates vascular tone by releasing endothelium-derived relaxing factor (EDRF) and contracting factors. Nitric oxide (NO) is the best characterized EDRF and plays a key role in protecting the vascular wall (1, 2). NO is produced via endothelial NO synthase (eNOS) and mediates the increase of intracellular cGMP which can inhibit Ca2+ entry into the cell thus producing the smooth muscle relaxation. It is widely accepted that the Ca2+ influx through plasma membrane Ca2+ channels plays an important role in the control of muscle tone (3).
Medicinal plants have been used for millennia for the treatment of diseases, and still many of today’s drugs are plant-derived natural products (4). Most of the therapies involve the use of plant extracts or their active compounds, as terpenoids that include their main subclasses mono-, diand tetraterpenes (5). At cardiovascular level, vasorelaxation, decreased heart rate and blood pressure were the main effects of 33 monoterpenes previously reviewed by Márcio et al (6).
Isoespintanol is a monoterpene isolated from leaves of Oxandra cf. xylopioides and their anti-inflamatory activity has been previously demonstrated (7). However, the vascular actions of this compound have not yet been examined.
Therefore, the objective of this study was to assess the action of isoespintanol on contraction and relaxation of isolated thoracic aorta rings analyzing the participation of NO.
MATERIALS AND METHODS
Extraction and identification of isoespintanol
Isoespintanol was obtained from Oxandra cf xylopioides as previously detailed (7). Briefly, dry and ground leaves of this vegetal product were extracted with petroleum ether by percolation and dried by rotary evaporation. The extract was subjected to various chromatographic columns by gravity, eluting with hexane-dichloromethane mixtures and finally recrystallizing. The structure of the compound was established on the basis of the interpretation of its NMR and MS data (8).
Experimental preparation
All procedures followed during this investigation were approved by the Institutional Animal Care and Use Committee (IACUC) of the Faculty of Medicine, University of La Plata following the Guide for the Care and Use of Laboratory Animals published by the Nacional Research Council, National Academy Press, Washington DC 2010 and/or European Union Directive for Animal Experiments 2010/63/EU.
Wistar rats of 4-5 months years old were anesthetized with an intraperitoneal injection of 25% urethane (0.6 mL by 100 g). The thoracic aorta was removed and placed in physiological salt solution (PSS) containing (in mmol/L): NaCl 130, KCl 4.7, Na2HPO4H 1.17, MgSO4 1.16, NaHCO3 24, CaCl2 1.6 and dextrose 11. The PSS was saturated with a mixture of 95% O2-5% CO2, had a pH 7.4, and was maintained at 37°C. After then, several rings 2-3 mm wide were obtained from every aorta, but each ring was used for only one experimental protocol. This model has been recently re-validated and considered as a “golden tool” in pharmacological researches (9). Aortic rings were suspended between a fixed point and a force transducer (Grass FT0.03D). A resting tension of 2 g was imposed by stretching and the preparation was stabilized in PSS for 1 hour prior to any experimental protocol (with one wash every 20 minutes). The signal from the transducer was amplified and entered into a DT2801A analog/ digital board (Data Translation, Inc.) mounted on a personal computer, originating a file using data acquisition software (Labtech Notebook Pro).
Protocols
Aortic contraction in presence of extracellular Ca 2+
Arterial ring contraction was induced through KCl due to its ability to depolarize cell membranes, and thereby opening voltage-dependent Ca2+ channels. The influx of extracellular Ca2+ into arterial cells occurs through those channels and results in vasoconstriction. In this functional study, 80 mM KCl was added to an organ bath media to induce constant contractions in TA rings after equilibration. Control aortic rings and rings exposed to ISO 0.6 μg/mL for 10 min were contracted with KCl. To assess the participation of nitric oxide synthase (NOS) L-NG-Nitro-Larginine methyl ester (L-NAME, inhibitior of NOS) 100 μmol/L was added in the last minutes 20 min of stabilization and the aforementioned ISO protocol was repeated. The contractile responses were calculated in mg of force per mg of tissue wet weight (mgF/mgW).
Relaxation aortic in absence of extracellular Ca 2+
The rings were exposed to contracting PSS, and when the force stabilized (~ 10-15 minutes) the solution was replaced by Ca2+-free relaxing PSS (made by omitting CaCl2 and adding 0.1 mM EGTA), obtaining a relaxation to the baseline. Control rings and rings incubated 10 min with ISO 30 μg/mL, in presence or in absence of NOS inhibition were contracted with KCl and relaxed in absence of Ca2+. The time to obtain 50% of relaxation (T-50) was measured. The tracing of relaxation was fitted to a monoexponential curve-fitting equation of the form F = a e -t/t where F is force, t is time and t is the relaxation time constant.
RESULTS
Exposure aortic rings to contracting solution produced a sustained contraction, which relaxed rapidly after switching to Ca2+-free relaxing PSS. The contraction was significantly greater in control than in rings treated with ISO 30 μg/mL (257 ± 19 vs. 360 ± 18 mgF/mgW). Relaxation was similar in control and ISO treated aortic rings, acquiring T-50 equal values in both groups (68 sec, Figure 1).
The curve-fitting analysis of the relaxation curves showed that ꞇ was similar in control and ISO treated aortic rings (103 ± 6 and 94 ± 11 msec, respectively). The inhibition of NOS with L-NAME abolished the decrease of maximal force of contraction observed after ISO addition. The values of F/W ratio were 319 ± 13 and 346 ± 34 for ISO+L-NAME and control +L-NAME, respectively (Figure 2A). The ꞇ values of control and ISO in presence of L-NAME were significantly higher than the same group in absence of NOS inhibition (Figure 2B).
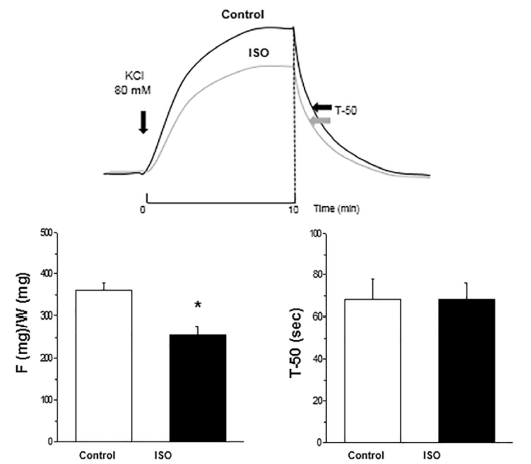
Figure 1 Top panel: Representative tracings of contractions induced in control and ISO treated aortic rings. Bottom panel: Average results of maximal force of contraction (F/W) and T-50 of relaxation for control and ISO groups. Note that F/W decreased and T-50 did not change in ISO compared to control aortic rings. *p< 0.05 ISO vs. Control.
DISCUSSION
This study demonstrates by the first time that the monoterpene isolated from the leaves of Oxandra cf xylopioides isoespintanol attenuates the contraction of aortic rings induced by high potassium and does not modify the magnitude and velocity of relaxation. Our data also show that the vascular effect of ISO was abolished by NOS inhibition with L-NAME.
Contraction in vascular smooth muscle can be initiated by mechanical, electrical, and chemical stimuli. Electrical depolarization of the vascular smooth muscle cell membrane (as produced by 80 mM KCl) elicits contraction by increasing the intracellular Ca2+ concentration, most likely by opening voltage dependent Ca2+ channels (L-type Ca2+ channels) (10). Accumulating evidence suggests that the transient receptor potential (TRP) channels modulate the driving force for Ca2+ entry through L-type Ca2+ channels and provide intracellular pathways for Ca2+ release from cellular organelles, thus increasing the intracellular Ca2+ concentration (11). The free Ca2+ binds to calmodulin and the complex Ca2+-calmodulin leads to myosin light chains (MLC) phosphorylation, then cross-bridge formation, and hence, smooth muscle contraction. Enhanced Ca2+ entry via upregulation of TRP or L-type voltage-dependent Ca2+ channels represent a critical mechanism involved in the development of pulmonary and systemic hypertension (12). In our experiments, isoespintanol attenuated the contractile force generated by 80 mM KCl suggesting that a lesser activation of Ca2+ channels and a reduction of intracellular Ca2+ concentration could be taking place in comparison to control.
Vascular smooth muscle relaxation occurs when there is reduced phosphorylation of MLC that can result from a reduction of Ca2+ or activation of phosphatase among other causes. Several members of TRP family such as TRP canonical type 3 channels have also been involved in this process (13-15). In this study, isoespintanol did not modify the aortic relaxation suggesting that this drug do not have any action on its involved mechanisms.
The eNOS-mediated signal pathway in vascular tissue has been well established (16). This enzyme synthesizes NO in a pulsatile manner and its activity markedly increases when intracellular Ca2+ rises (17). NO acting through cGMP can stimulate a cGMP-dependent protein kinase (cGK) that activates MLC phosphatase and also can inhibit Ca2+ entry through L-type Ca2+ (18) or/and TRP channels (19). Both actions lead to smooth muscle relaxation. Our data show that the decreased contraction force achieved by isoespintanol was abolished by L-NAME treatment indicating that NO participates in the vascular effects of that compound.
One of the major groups of biologically active phytochemicals is the terpenes. These compounds are mostly hydrocarbon in nature and their building block is a five-carbon isoprene unit. The structure of most terpenes consist of ‘head-to-tail’ condensation of isoprene units and they are classified according to the number of isoprene units. Consequently, there are monoterpenes, diterpenes, triterpenes, etc, (20). Earlier studies have shown that both monoterpenes and their synthetic derivatives possess a variety of pharmacological properties through modulation of activities of various ion channels (21). In this sense, previous investigations show that changes of Ca2+ influx via TRP channels are involved in the vascular effects of monoterpenes carvacrol and menthol (22, 23). Our data does not allow us to evaluate any action of isoespintanol on TRP and/or L-type Ca2+ channels. Knowing the important role of Ca2+ in the smooth muscle contraction-relaxation cycle we only can speculate that in presence of isoespintanol the intracellular Ca2+ concentration must be lower than in absent of the compound.
Vascular function can also be regulated through several types of potassium channels, expressed not only on sarcolemma but also on endothelial layer. It has been demonstrated that a high level of depolarization (induced by high KCl) produced a reduction in the potency and efficacy of drugs activating potassium channels (24).
Considering the important roles of ion channels in cardiovascular disorders (25), further investigations on the effects of isoespintanol on the functional properties of these channels could enable its utilization as a preventive therapy of those pathologies.
CONCLUSIONS
Our results show that pretreatment with isoespintanol produces a relaxant effect on aorta that was significantly attenuated by NOS inhibition with L-NAME.
Therefore, our findings propose that isoespintanol acting on endothelial TRP channels and/or membrane receptors induces Ca2+-mediated eNOS activation and a consequent increase of NO production. This molecule reaches the smooth muscle cell and decreases the Ca2+ concentration (through TRP or/and L-type Ca2+ channels) thus leading to vasorelaxation (Figure 3).
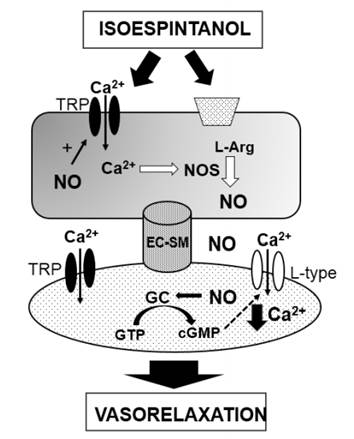
Figure 3 Possible mechanisms involved in the vascular actions of isoespintanol. TRP: Transient receptor potential channels; EC: Endothelial cell; SM: smooth muscle.
Although the in vitro model is very useful and simple to assess the vascular actions of new drugs or compounds more data are needed, especially human intervention studies, to extend the present knowledge to clinical area.