INTRODUCTION
Infusion of leaves from Passiflora quadrangularis L (known as Badea) is used in Colombian folk medicine for hypertension, anxiety and diabetes. This plant belongs to the Passifloraceae family, with 167 species found in Colombia, from the Ancistrothyrsus, Dilkea and Passiflora genera [1]. In previous studies, the ethanolic extract of leaves administered orally was able to decrease blood pressure in Wistar rats with L-NAME-induced hypertension, as well as prevent against vascular remodeling [2]. Aqueous and ethanolic extracts have also been shown to have anxiolytic-like properties [3].
The main constituents of P. quadrangularis L. are flavonoids [4,5], β-carboline harmane alkaloids [6] and triterpene saponins [4,5,7,8].
Triterpene saponins are natural products present in many organisms, especially in plants, and exhibit interesting biological and pharmacological activities, including anti-inf lammatory, antimicrobial, antiviral [9,10] and antitumor effects [11].
Hypertension is a major modifiable risk factor for cardiovascular disease and premature mortality worldwide [12]. Natural products have been important sources of new drugs, and the treatment of hypertension with plant extracts or plant derived products is well documented [13].
This work shows the isolation and structural elucidation of two known monodesmosidic triterpene saponins from P. quadrangularis L. with vasoconstrictor effects: 3-O-β-Dglucopyranosyloleanolic acid (compound 1) [14] and 3-O-[β-D-glucopyranosyl-(1→2)-β-Dglucopyranosyl]oleanolic acid (compound 2) [8].
MATERIALS AND METHODS
Plant material and extraction
Leaves of P. quadrangularis L. were collected in Bella Vista - Algeciras - Huila, Colombia (latitude 2.54750 - longitude -75.28727), with an elevation 1100 MASL, and an average temperature of 24 °C. Voucher specimens were deposited at the Colombian National Herbarium (COL N° 602879). The leaves were dried at 40 °C in a circulating air oven. Then, the dried material was pulverized and macerated with 96% ethanol for 72 hours, then filtered and concentrated with a rotary evaporator.
Extraction and isolation
The ethanolic extract of P. quadrangularis L. (39 g) was dissolved in methanol and stored at 18 °C for 14 hours until the wax precipitated. The extract without wax (37 g) was separated into two fractions, with dichloromethane (CH2Cl2) and methanol (MeOH), in different proportions, obtaining a soluble (22 g) and insoluble (15 g) CH2Cl2 fraction. The soluble CH2Cl2 fraction was further fractionated by flash column chromatography. The elution system was based on previous thin layer chromatographic (TLC) analysis using hexane, ethyl acetate (EtOAc) and MeOH. Silica gel 60 (0.040-0.063 mm) (Merck®) was used as the stationary phase and the mobile phase increased in polarity. Then, 15 mL fractions 180-237 (1.1 g) and 254-270 (1.5 g) were collected according to the TLC profile.
Fractions 180-237 were mixed and f lash chromatographed on silica gel using CH2Cl2/ MeOH (9:1) as the mobile phase. This process led to sub-fractions 5-6, which yielded 331 mg of pure compound 1 [Figure 1]. Fractions 254-270 were also separated by flash column chromatography. The system of elution was CH2Cl2/MeOH (8:2) as the mobile phase and silica gel as the stationary phase; sub-fractions 35-43 were obtained, yielding 1g of pure compound 2 [Figure 1].
HRMS analysis was performed using an Applied Biosystems QSTAR XL spectrometer, UV spectra were recorded on a Perkin-Elmer FT-IR, System spectrum BX spectrophotometer, the melting point was measured on Gallen Kamp apparatus and NMR (1 H and 13C) spectra were recorded on a Bruker DRX-400 spectrometers. Chemical shifts (δ) were given in ppm downfield from tetramethylsilane (TMS) as an internal standard and coupling constants (J values) were in Hertz.
Compound 1: 3- O - β -D-glucopyranosyloleanolic acid
MP: 230-233ºC (EtOAc)
HRMS: m/z [M+Na+] calcd for C36H58O8: 641.4030
IR (KBr): 3417, 1693, 1463, 1386, 1077 cm-1. 1 H NMR (400 MHz, CD3OD) δ: 3.25 (1H, m, H-3), 5.23 (1H, bs, H-12), 3.40 (1H, dd, J = 2.0, 12.0 Hz, H-18), 1.04 (3H, s, H-23), 0.90 (3H, s, H-24), 0.80 (3H, s, H-25), 0.93 (3H, s, H-26), 1.15 (3H, s, H-27), 0.94 (3H, s, H-29), 0.84 (3H, s, H-30), 4.30 (1H, d, J =7.2 Hz, glc-H1), 3.83 (1H,dd, J=2.0, 9.8 Hz, glc-H6a), 3.65 (1H,dd, J=5.2, 9.8 Hz, glc-H6b)
13C NMR (400 MHz, CD3OD) δ: 39.8 (CH2, C-1), 27.0 (CH2, C-2), 90.8 (CH, C-3), 40.1 (C, C-4), 57.0 (CH, C-5), 19.4 (CH2, C-6), 33.7 (CH2, C-7), 40.1 (C, C-8), 48.3 (CH, C-9), 37.9 (C, C-10), 24.1 (CH2, C-11), 123.6 (CH, C-12), 145.2 (C, C-13), 42.7 (C, C-14), 28.8 (CH2, C-15), 24.0 (CH2, C-16), 47.6 (C, C-17), 42.9 (CH, C-18), 47.7 (CH2, C-19), 31.6 (C, C-20), 33.8 (CH2, C-21), 34.0 (CH2, C-22), 28.5 (CH3 , C-23), 16.0 (CH3 , C-24), 17.0 (CH3, C-25), 17.7 (CH3, C-26), 26.4 (CH3, C-27), 180.8 (C, C-28), 33.8 (CH3, C-29), 24.0 (CH3, C-30), 106.7 (CH, C-1’), 77.6 (CH, C-2’), 78.2 (CH, C-3’), 71.6 (CH, C-4’), 77.9 (CH, C-5’), 62.8 (CH2, C-6’)
Compound 2: 3-O-[β-D-glucopyranosyl- (1→2)-β-D-glucopyranosyl]oleanolic acid
MP: 248ºC (EtOAc/Ether)
HRMS: m/z [M+Na+] calcd for C42H68O13: 803.4588
IR (KBr): 3400, 1645, 1458, 1365 cm-1.
1 H NMR (400 MHz, CD3OD) δ: 3.20-3.40 (1H, m, H-3), 5.23 (1H, bs, H-12), 1.07 (3H, s, H-23), 0.90 (3H, s, H-24), 0.80 (3H, s, H-25), 0.93 (3H, s, H-26), 1.15 (3H, s, H-27), 0.94 (3H, s, H-29), 0.86 (3H, s, H-30), 4.42 (1H, d, J=7.2 Hz, glc-H-1’), 4.66 (1H,d, J=7.6 Hz, glc-H-1”), 3.54 (2H, m, H-6’), 3.82 (2H, m, H-6”)
13C NMR (400 MHz, CD3OD) δ: 39.8 (CH2, C-1), 27.1 (CH2, C-2), 91.5 (CH, C-3), 40.4 (C, C-4), 57.0 (CH, C-5), 19.3 (CH2, C-6), 33.6 (CH2, C-7), 40.5 (C, C-8), 48.3 (CH, C-9), 37.9 (C, C-10), 24.0 (CH2, C-11), 123.6 (CH, C-12), 145.2 (C, C-13), 42.7 (C, C-14), 28.5 (CH2, C-15), 24.5 (CH2, C-16), 47.2 (C, C-17), 42.9 (CH, C-18), 47.6 (CH2, C-19), 31.6 (C, C-20), 33.6 (CH2, C-21), 33.8 (CH2, C-22), 28.5 (CH3, C-23), 16.0 (CH3, C-24), 17.0 (CH3, C-25), 17.7 (CH3, C-26), 26.4 (CH3, C-27), 181.2 (C, C-28), 33.6 (CH3, C-29), 24.0 (CH3, C-30), 106.4 (CH, C-1’), 77.6 (CH, C-2’), 78.3 (CH, C-3’), 71.9 (CH, C-4’), 78.7 (CH, C-5’), 62.8 (CH2, C-6’), 105.4 (CH, C-1”), 81.0 (CH, C-2”), 77.9 (CH, C-3”), 71.5 (CH, C-4”), 78.4 (CH, C-5”), 63.0 (CH2, C-6”)
Aortic rings preparation
Wistar male rats (200-300 g) were euthanized using a CO2 chamber. The descending thoracic aorta was dissected by thoracotomy and immersed in Krebs solution for its preservation. The surrounding tissue of aorta was removed, and fragments were cut into 3 mm lengths. Thoracic aortic rings were connected using an isometric transducer (fort 10/ WPI) and submerged in Allhin organ chambers containing 10 mL of Krebs solution, with bathing medium maintained at 37 °C, pH 7.38-7.42 and continuously exposed to a carbogen mixture of 95% O2 and 5% CO2.
A basal tension of 2 g was applied to each aortic ring with a stabilization period of 60 min, during which time the Krebs solution was periodically changed, and tension was reset. Once equilibrium was reached, the aortic rings were exposed to phenylephrine (PE 1µM) until the contractile response reached a steady tension and the subsequent concentration of acetylcholine (ACh 1µM) was added to verify the functionality of the endothelium. PE contractile response was used as a reference for normalization of the test compounds response.
Compounds 1 and 2 and ethanolic extract were added cumulatively between 10 and 400 µM and 10 and 320 µg/mL respectively, and clonidine (as reference drug) was added between 1 nM and 100 µM every 15 min using a logarithmic scale. In the other experiments, aortic rings without endothelium, test agents such as alpha-antagonist (yohimbine 1 and 100 µM, prazosin 1µM and phentolamine 1µM) and a blocker of L-type calcium channels (verapamil, 10 and 100 µM) were added to the organ bath 5-10 min before the cumulative addition of compounds 1 and 2, extract or clonidine.
Independent and endothelium dependent mecha nisms were ex a mined w ith SN P (1nM to 100µM) and ACh (10nM to 10µM), respectively, in rings pre-contracted with saponins (400 µM, Emax) and the extract (320 µg/mL, Emax). When necessary, the endothelium was removed by rubbing the intimal surface with a thread, after which 1µM ACh was added to check for absence of the endothelium in the aortic rings; relaxation up to 10% was accepted. After this, they were washed with Krebs solution until reaching basal tension again and compounds 1 and 2, ethanolic extract and clonidine were added cumulatively.
The experimental procedure was approved by the institutional ethics committee (Act 14, November 2017, Faculty of Sciences, at the Universidad Nacional de Colombia). The animals were supplied by the Bioterium of the Pharmacy Department at the Universidad Nacional de Colombia.
Data analysis and statistics
The response of aortic rings was expressed as a percentage of the initial contraction to 1µM PE. Dose-response curves were analyzed to give the negative log of the concentration of compounds 1 and 2, ethanolic extract and clonidine, producing a 50% half maximal effective concentration (pEC50) by sigmoidal curve-fitting analysis. All results are expressed as means ± the standard error of the mean (S.E.M.); n=5-14, where n indicates the number of animals or number of aortic rings. Differences in concentration response curves between the extract and isolated compounds with antagonists were analyzed by the unpaired Student´s t-test or Mann- Whitney as appropriate, with a criterion set for statistical significance at p< 0.05. The responses to ACh and SNP are expressed as a percentage of PE contraction. Doseresponse curves were analyzed to give the negative log the concentration of ACh or SNP, producing a 50% half maximal effective concentration (pIC50) by sigmoidal curve-fitting analysis. Excel (Microsoft® Office Excel 2013) and GraphPad Prism 6 software were used for data analysis.
Drugs and solutions
The following salts and drugs were used: NaCl, CaCl2, NaHPO4, NaHCO3, glucose, KCl, MgCl2, KH2PO4, MgSO4, ascorbic acid (Panreac), acetylcholine, phenylephrine, sodium nitroprusside (Sigma - Aldrich), dimethylsulfoxide (DMSO) (Loba Chemie), clonidine, verapamil, yohimbine, prazosin and phentolamine (Cayman Chemical). All substances were dissolved in DMSO. This vehicle was used at a final concentration of 0.1%.
RESULTS
Structural elucidation of saponins isolated from Passiflora quadrangularis L.
For compound 1, the molecular formula C36H58O8 was deduced based on the highresolution mass spectrometry (HRMS) spectrum which displayed a pseudo-molecular ion peak at m/z 641.4030 [M+Na+]+. The structure idenfication of compound 1 was attained through the analysis of its 1H and 13C NMR spectral data resumed as follows. Carbon signals were observed for a free carboxyl group at position C-28 (δC 180.8), and for one anomeric carbon of a β-D-glucopyranoside (δC 106.7, associated to a methine proton signal at δH 4.30, 1H, d, J=7.2), which along with the oxygenated methine signal at δC 90.8 (assigned to C-3 of the aglycone) and to the remaining 13C and 1H signals in the NMR spectra, supported the structure of an unsaturated pentacyclic triterpene acid, suggesting the proposal of an oleanolic acid derivative [14,15] for compound 1. As the result, compound 1, being isolated for the first time from this plant, was finally identified as 3-O-β-Dglucopyranosyloleanolic acid [Figure 1]. As a proof of the glucoside location at C-3, in the HMBC 2D-NMR correlation spectrum for compound 1 (see suppl. mat. fig S6), the C-3 signal was correlated with those of the anomeric H-1´ and with those of protons H-23 and H-24 of the geminal methyl groups attached to C-4.
The striking similarity of the 13C NMR spectra of compounds 1 and 2 readily suggested that compound 2 was also a derivative of oleanolic acid, though with two glucose (Glc) residues attached to C-3, as proved by the presence of two signals of anomeric carbons (δC 106.4 and δC 105.4) in its spectrum. In the HRMS, compound 2 exhibited a peak at m/z 803.4588 [M+Na+]+ that was consistent with the presence of two Glc residues. The inter-glycosidic linkage of this disaccharide was determined to be Glc (1→2) Glc by the chemical shift of a CH signal of the 13C NMR spectrum (δC 81.0, C-2’) [14]. Also, in the HMBC spectrum, the C-3 signal showed correlations with H-1´, and with those methyl protons H-23 and H-24 (see suppl. mat. fig S13). Additionally, The C-1” anomeric carbon correlations with protons H-2´ and H-2”, thus establishing C-2’ as the linking position of the second sugar unit. Consequently, compound 2 was identified as the monodesmosidic 3-O-[βD-glucopyranosyl-(1→2)-β-D-glucopyranosyl] oleanolic acid (C42H68O13), previously reported [8] [Figure 1].
Effect of yohimbine, phentolamine, prazosin and verapamil in aorta contracted with compound 1, compound 2 and ethanolic extract without endothelium
The α1 -adrenergic agonist PE, 1µM, used as reference agent in all assays, induced a maximal vasoconstrictor response of 1613 ± 55mg.
The ethanolic extract of Passiflora quadrangularis L. induced concentration-dependent contraction in intact and denuded rings without significant differences p>0.05 (maximum effect-Emax): 98±1.66%, pEC50: 4.08±0.009 and Emax: 105±1.92%, pEC50 4.0±0.007 respectively [Table 1]. Pretreatment with yohimbine (1µM), phentolamine (1µM) and prazosin (1µM) attenuated the ethanolic extract induced contraction in a concentration dependent manner in denuded rings (p<0.05 in all cases) [Figure 2-A, Table 2]. Pre-treatment with verapamil (10µM) attenuated the ethanolic extract induced contraction in a concentration-dependent manner in denuded rings (p<0.05) [Figure 2-A, Table 2]. SNP but not ACh, was able to decrease the vasopressor effect of compounds 1 and 2 [Figure 3-A; 3-B, Table 3].
Table 1: Emax and pEC50 of compound 1, compound 2 and ethanolic extract in aortic rings with endothelium or denuded.

The results are expressed as mean ± S.E.M of the number of dates. pEC50 (-logCE50) and Emax (percent of 1µM, PE maximal contraction). Units for pEC50 are expressed as g/mL for whole crude ethanolic extract and M for Compound 1 and 2. There are not statically differences for compound 1 and 2, p>0.05.
Table 2 Effect of yohimbine, phentolamine, prazosin and verapamil in aorta contracted with compound 1, compound 2 and ethanolic extract without endothelium.
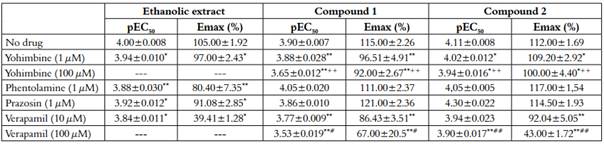
The results are expressed as mean ± S.E.M of the number of dates. pEC50 (-logCE50) and Emax (percent of 1 µM, PE maximal contraction). Units for pEC50 are expressed as g/mL for whole crude ethanolic extract and M for Compound 1 and 2. *p<0.05 vs. No drug; +p<0.05 vs. Y (1 µM); #p<0.05 vs. V (10 µM).
Compound 1 induced a vasoconstrictor response in intact rings (Emax 109±1.96%; pEC50: 3.92±0,011) and denuded rings (Emax: 115±2.26%; pEC50:3.90±0.007) [Table 1]. Pre-treatment with yohimbine 1 µM and Verapamil 10 µM attenuated the compound 1-induced contraction in a concentration-dependent manner in denuded rings (p<0.05) [Figure 2-B, Table 2], whereas pretreatment with prazosin (1 µM) and phentolamine (1 µM) did not show significant differences (p>0.05) [Table 2]. Yohimbine and verapamil 100 µM were also evaluated (p<0.05) [Figure 2-B). Concentration-response curves ACh and NPS showed the same behavior as the extract [Figure 3-A; 3-B, Table 3].
Compound 2 induced a vasoconstrictor response in intact rings (Emax: 109±1.14%; pEC50: 4.09 ± 0.011) and denuded rings (Emax: 112 ± 1.69%; pEC50: 4.11 ± 0.008) [Table 1]. Pre-treatment with yohimbine (1 µM and 100 µM) and verapamil (10 µM and 100 µM), attenuated the compound 2 induced contraction in a concentration-dependent way in denuded rings (p<0.05) [Figure 2-C, Table 2]. In contrast, pre-treatment with prazosin (1 µM) and phentolamine (1 µM) did not show any significant differences (p>0.05) [Table 2]. Concentration-response curves ACh and NPS presented the same behavior as the extract [Figure 3-A; 3-B, Table 3].
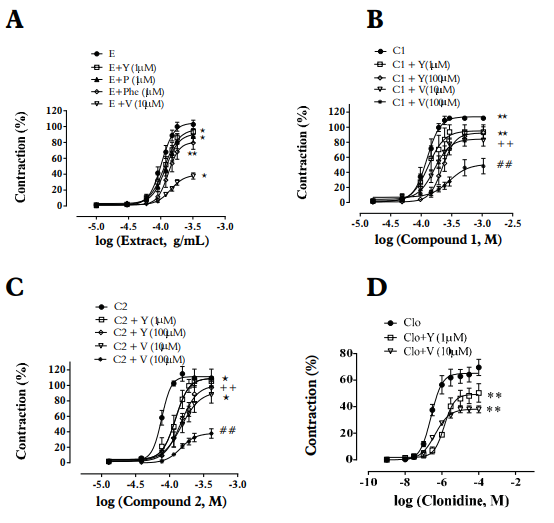
Figure 2 Effect of yohimbine (Y), α2-adrenoceptor antagonist; phentolamine (Phe), α1 - and α2-adrenoceptor antagonist, prazosin (P), α1 -adrenoceptor antagonist; and of verapamil (V), blocker of L-type calcium channels on the contraction effect induced by ethanolic extract (E) - A, compound 1 (C1) - B, compound 2 (C2) - C and clonidine (Clo) - D in endothelium-denuded the aortic rings of Wistar rats. E (10-320 µg/ml), C1, C2 (10-400 µM) and Clo (1nM- 100 µM) induced contraction was performed in arteries contracted with phenylephrine (1 µM) in the absence or presence of Y (1 µM), P (1 µM) or V (10 µM). Y (100 µM) and V (100 µM) only for C1 and C2. Data are reported as the mean ± S.E.M. (n = 5-9). *p<0.05 vs. E, C1, C2 or Clo); +p<0.05 vs. Y (1 µM); #p<0.05 vs. V (10 µM). Student’s t-test - Paired.
Table 3 Effect of ACh and SNP in aortic rings with endothelium contracted with C1, C2 (400µM) and E (320 µg/ml).
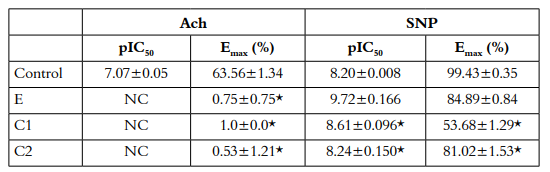
The results are expressed as mean ± S.E.M of the number of dates show in Figure 3. pIC50 (-logCE50, M) and Emax (percent of 1 µM, PE maximal contraction).NC: Not calculated because the relaxation is too small. *p<0.05 vs. Control
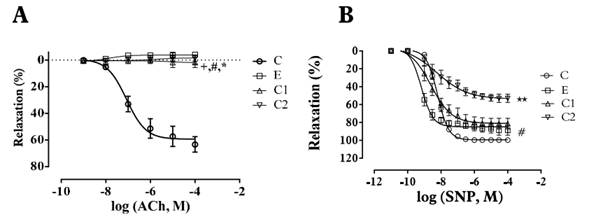
Figure 3 Effect of extract (E), compound 1 (C1) and compound 2 (C2) on the relaxation effect induced by ACh - A and SNP - B in endothelium-intact aortic rings of Wistar rats. Arteries contracted with E (320 µg/ml), C1, C2 (400 µM) induced contraction. Data are reported as the mean ± S.E.M. (n = 5-9). +p<0.05 (E vs ACh or SNP); #p<0.05 (C1 vs. Ach or SNP); * p<0.05 (C2 vs. ACh or SNP). Student’s t-test - Paired.
Pre-treatment with yohimbine 1 µM and Verapamil 10 µM decreased the clonidine induced contraction in a concentration-dependent manner in denuded rings (p<0.05) [Figure 2-D, Table 4]. Clonidine was used as a comparative α2-adrenergic agonist with respect to compounds 1 and 2.
Discussion
This work shows that compounds 1 and 2, saponins isolated from Passiflora quadrangularis L., induced vasoconstrictor effects in isolated aortic rings. This response could be related to Ca2+ influx via voltage operated Ca2+ channels, and/or on α2- adrenergic receptor activation.
However, other compounds could be implicated in the cardiovascular response induced by P. quadrangularis L. Among them: harmane-type or β-carboline-type alkaloids, vitexine-type flavonoids and cycloartenol saponins, that could add to the oleanane saponins response decrypted in this study.
In previous studies, the extract of leaves of P. quadrangularis L. and other species of this genera, showed sedative and anxiolytic effects and triterpene saponins could be responsible for these effects [3, 16], as well as for preventing the hypertension induced by nitric oxide deficit in rats [2].
It is known that stimulation of post-junctional α2-adrenergic receptors on arterial smooth muscle mediates a pressor response, whereas their presynaptic activation on central nervous system induces a reduction in sympathetic outf low, manifested as a reduction in the arterial blood pressure, bradycardia and sedative effects [17]. Thus, an agent with alpha-2 agonism properties elicits vasoconstriction in isolated aortic rings. That could be the case of compounds 1 and 2.
Saponins show therapeutic interest for targeting several diseases, with reports of antitumor, hepatoprotective, neuroprotective, antihyperglycemic, osteogenic [18], anti-inflammatory, antifungal, antibacterial [9] and antihypertensive effects [19] 14-week-old SHRs were randomly divided into three groups; the first was administered low-dose saponin, the second with high-dose saponin and the third with a placebo as the control group. An additional eight healthy male Wistar rats were used as the normal group. The blood pressures (BPs. The triterpenoids, specially from plants, also display biological and pharmacological properties like anticancer, antimicrobial and antiinflammatory activities, and cardiovascular effects [10, 21].
For saponin structure-activity relationships, both the aglycone and the number of sugar units seem to play an important role in their biological activity. In addition, 1→2 (present in compound 2) and 1→4 linkages give higher activity than the 1→3 linkage [11]. Related to the present work, 3-O-[βD-glucopyranosyl-(1→2)-β-D-glucopyranosyl] oleanolic acid was previously reported by Orsini [8]; while 3-O-β-D-glucopyranosyloleanolic acid was previously described in Passiflora alata C. [14], but not in P. quadrangularis L. The vasoconstrictor effects of the two compounds are reported for the first time.
Compound 1, compound 2 and the ethanolic extract, elicited maximal vasoconstrictor effect in aortic rings with intact endothelium, response that not changed after de-endothelization. This leaves to suppose that site of action of those metabolites places at vasculature media layer. Given that alpha adrenergic receptors are located in smooth muscle (α1 , α2 ) [21,22], and they are connected to calcium signalling [23]. Compounds 1 and 2 could mediate calcium currents activation on smooth muscle via α stimulation, being key metabolites responsible for the global effect seen with P. quadrangularis L.
The idea of a possible mechanism of compounds 1 and 2 related to activation of α2-adrenoceptor/ calcium currents pathway is supported observing that their potency (pEC50) and efficacy (Emax) of contraction were reduced more against yohimbine and verapamil than against prazosin and phentolamine [Figure 2, Table 2]. Ethanolic extracts also contain other compounds with possible effects on α1 -adrenoceptor or unspecific receptors, while compounds 1 and 2 have effects on α2- adrenoceptors or calcium channels.
Previous studies have described the influence of calcium channel blockers on the stimulation of postsynaptic α1 - and α2-receptors in resistance vessels in pithed rats. The vasoconstriction induced by postsynaptic α2-adrenoceptor stimulation is extremely sensitive to Ca2+ entry blockers evoked by Ca2+ antagonists, whereas the vasoconstriction produced by α1 -receptor stimulation is much less likely to be affected by Ca2+ antagonistic drugs.
For example, the effect of methoxamine, a selective α1 -adrenoceptor agonist, is unchanged by Ca2+ entry blockers. Also, the effect of α1 -agonists is not dependent on the extracellular current of Ca2+, but on its intracellular stimulation from the sarcoplasmic reticulum. These in vivo findings have been confirmed in other studies [24].
It has been suggested that the response of vascular smooth muscle to α- adrenergic agonists like norepinephrine and PE depends on both, extracellular Ca2+ influx and the liberation of Ca2+ from intracellular stores. The effect of selective α2- adrenergic agonist seems to depend exclusively on extracellular Ca2+ and via Gi -mediated inhibition of adenylyl clyclase [17, 25].
According to radioligand studies, calcium entry blockers have low affinity for adrenoceptors α2. Therefore, the decrease in vasoconstriction induced by compounds 1 and 2 stimulation of the α2-adrenoceptor cannot be attributed to the direct action of Ca2+ antagonistic drugs on the receptor. It is known that the postsynaptic stimulation of α2-receptors by a suitable agonist is accompanied by an influx of Ca2+, which allows the activation of contractile proteins; calcium antagonists inhibit this internal flow of Ca2+ and therefore impair the contractile process [24].
The α2-adrenoceptors agonist clonidine and related compounds are recognized as clinically efficacious antihypertensive drugs [26]; in the present study, cumulative concentration response curves of clonidine were constructed in denuded arteries. Yohimbine and verapamil attenuated clonidine-induced contractions in accordance with the above; compounds 1 and 2 showed similar behaviors, suggesting that these compounds induced contraction which involves α2-adrenoceptor activation. In other studies of contracted aortic rings without endothelium with clonidine, this response decreased following the removal of extracellular Ca2+ and pre-treatment with nifedipine [25].
This study suggests that these substances induce contraction mainly dependent on Ca2+ influx from the extracellular space via voltage-operated calcium channels. But this effect could be, at least a partially, due to α2-adrenergic receptors stimulation. Dexmedetomidine for example, an α2-adrenoceptors agonists, induces contraction through the of Ca 2+ influx [27]. However, contraction mediated throw α1 -adrenergic cannot be totally discarded.
Considering previous reports that suggest the usefulness of P. quadrangularis L. extract in lowering blood pressure in vivo [2, 29], as well as its sedative effect [3], the monodesmosidic triterpene saponins identified in this work would induce moderately selective α2-adrenoceptor activation of vascular smooth muscle and a2 presynaptic stimulation on central nervous system [29]. Notwithstanding, P. quadrangularis L. could contain other compounds which cardiovascular effect have yet to be studied.
Assays with ACh and SNP support the idea that the vasoconstrictor mechanisms seen on this work do not depend on endothelial-linked pathways, since ACh was unable to affect the vasoconstrictor response induced by compounds 1 and 2. Therefore, SNP would reduce, via cGMP[30], the vasoconstrictor effect elicited throw α2-adrenoceptor stimulation, extracellular calcium mobilization and adenylyl cyclase inhibition [17, 31] [Figure 3, Table 3].
According to these results, cGMP cascade induced by NO released by SNP would be more efficiently inhibited by compound 2 [32] and therefore, compound 2 would blockade the influx of Ca2+ into the cell, while compound 1 would stimulate the activation of α2-adrenergic receptors [Figure 3, Table 3], but further studies regarding the signal transduction pathway associated with the calcium release induced by compounds 1 and 2 are needed to determine the detailed mechanism.
P. quadrangularis L. has several types of saponins [7, 8]. Two known monodesmosidic triterpene saponins with vasoconstrictor activity in isolated rings of rat aorta were identified: 3-O-β-Dglucopyranosyloleanolic acid and 3-O-[β-Dglucopyranosyl-(1→2)-β-D-glucopyranosyl] oleanolic acid. 3-O-β-D-glucopyranosyl-oleanolic acid is reported here for first time for this plant. Further studies are required to determine the underlying mechanism of action of these compounds.
CONCLUSIONS
In conclusion, 3-O-β-D-glucopyranosyloleanolic acid and 3-O-[β-D-glucopyranosyl-(1→2)-β-Dglucopyranosyl]oleanolic acid are key metabolites responsible for the vasoconstrictor response elicited from P. quadrangularis L. Ca2+ influx via voltage-operated channels activation and/or α2- adrenoceptor stimulation mechanisms could be implicated.