Introduction
The green asparagus (Asparagus officinalis L.) is a vegetable widely consumed by its turgid texture and high nutritional value. Freshly harvested spears are very susceptible to senescence, mainly related to their high respiration rate. They undergo several physiological changes during storage, such as transpiration, chlorophyll degradation, and total phenols changes (1). Usually, the spears present a high quantity of phenolic compounds, being the flavonoids with the greater antioxidant activity potential (2). Microorganisms easily contaminate the vegetables at various points during the production, handling, and packing process. Therefore, eliminating microorganisms during postharvest processing is vital to assure microbial safety (3).
Various postharvest treatments have been studied to prolong the quality of fruits and vegetables, including chemical disinfectants such as sodium hypochlorite (chlorine) and ozone, which are highly effective in reducing microorganisms’ load (4). Ozone in the water or gas phase is an interesting alternative because it is an effective disinfectant recognized for its high oxidizing potential on the microbial cell membrane. The disinfecting effect of ozone is approximately 150% more effective than the commercial disinfectant known as chlorine (5).
Ozone has been generally recognized as a safe disinfectant approved by the United States Food and Drug Administration (FDA) (6). Ozone application has gained increasing commercial interest as a postharvest technology to control spoilage in fruits and vegetables, especially because it leaves no residue in the treated product (7).
The quality of fruits and vegetables is evaluated mainly from the sensory, nutritional, and microbiological aspects. Consumers often quickly assess the sensory quality in retail stores and consider attributes such as appearance, color, and texture as a priority. Impairment of these properties would influence the product’s shelf life and purchase intent, particularly when these attributes fall below the acceptable level (8).
Response surface methodology (RSM) has been commonly used for optimizing, designing, developing, and improving processes where a response or responses are affected by several variables (9). It is a technique of statistical and mathematical methods to assess numerous factors and their interactions established on the fit of a polynomial model. It must depict the behavior of the dependent variable to make statistical predictions to optimize the response (10). The correct experimental design must be selected to designate which treatments should be done in the experimental region being studied. For this purpose, experimental designs for quadratic response surfaces, such as three-level factorial, central composite (CCD), and Box-Behnken (BBD), should be applied (11). We have reviewed some RSM studies published in fresh food industry processes, such as green pepper (12), french beans (13), chinese cabbage (10), onion (14), broccoli (15), spinach (16).
The objective of the current study is to determine through the response surface methodology the concentration of gaseous ozone and storage time, which allows obtaining the minimally processed green asparagus with the highest content of phenols and overall appearance, and lowest microbial count.
Materials and methods
Material
The research material was green asparagus spears variety UC157 F1, purchased from Chao province (coordinates 08°32′25″ S and 78°40′39″ O), department of La Libertad, Perú. The green asparagus (maximum 07 hours after being harvested) showed no physical damage (bumps, bruises, etc.) and were free of pests and any strange odor. According to NTP 011.109:2008 (17), they were classified with AB tip quality and 14-20 mm caliber.
Minimum process treatment
Spears were washed by aspersion with potable water to remove surface impurities and immersed in a chlorine dioxide solution at 100 ppm for 5 min at room temperature. Afterward, they were cut to a length of 17 cm, considering the measure from the tip to the base. The excess humidity was eliminated. The spears were conditioned in trays of expanded polystyrene with a weight of 150 g ± 3 g, and then transferred to the treatment chamber, where the gaseous ozone generated was injected by an ozonification machine (Ozonomatic brand, model OZ-500) with a flow rate of 500 mg/h and through a 7 mm diameter hose, subjecting them to different concentrations between 0 - 10 ppm, during 20 min, controlled by using a digital gaseous ozone meter (Crowcon brand, model Gasman O3, range 0-100 ppm, sensitivity ± 0.1 ppm). Finally, the trays with spears were removed from the chamber and covered with polyvinyl chloride (PVC) film and stored in a refrigeration chamber with temperature control for 28 days at 1 °C ± 0.1°C and 85-90% ± 2% relative humidity (usual storage conditions in the commercialization of asparagus), control performed with a thermohydrometer (Fluke brand, Model 971).
Analytical techniques
Total phenol content
2 g of sample was homogenized under darkness in 80% aqueous ethanol for 2 h, at room temperature, and centrifuged at 4200 rpm for 15 min; the supernatant was evaporated in an oven at 40 °C. The residues were dissolved in 5 mL of distilled water, extracting 100 μL that was diluted with 3 mL of distilled water, and then 0.5 mL of Folin-Ciocalteau reagent was added. After 3 min, 2 mL of 20% (w/v) sodium carbonate solution was added, and the resulting material was vigorously mixed. The color absorbance developed after 1 h was measured in a visible light spectrophotometer (Thermo Electron Corporation, model Spectronic Genesys 6) at 765 nm, using gallic acid as standard. The results were expressed as mg gallic acid/g sample (1, 18).
Overall appearance
It was determined following the method of Albanese et al. (2007) with modifications by the author. Thirty untrained panelists, aged between 25 - 45 years and habitual consumers of green asparagus were selected for the test. The criteria to be evaluated were: spear shape based on entire terminal buds and completely closed bracts; color according to the degradation of chlorophyll (less intense); spear firmness according to the lignification that was observed when applying pressure to the touch; dehydration of the shoot that referred to a wrinkled product; and rot damage that was evidenced by the tissue softness. All participants involved in the evaluation were thoroughly informed about the characteristics of the product to be evaluated. The factors mentioned were responsible for the loss of freshness in asparagus. A structured hedonic scale of 9 points was used, where 1: I dislike it very much, 0: I neither like it nor dislike it, and 9: I like it very much. The evaluation was carried out in a single session, in an environment with natural lighting, and the average of the values was reported (19)
Total count of viable mesophilic aerobic bacteria, psychrophiles, and molds
10 g of the sample were aseptically separated and homogenized in 90 mL of 0.1% peptone water. A series of dilutions were prepared in 9 mL of peptone water with 1 mL of aliquot. In duplicate, the count of viable mesophilic aerobic and psychrophilic bacteria was determined using the plate count agar (PCA) and standard methods agar. The plates were incubated at 35 °C for 48 h for viable mesophilic aerobic bacteria and 7 days at 7 °C for psychrophilic bacteria. Count molds were evaluated on pink flare red agar (RBRM) and incubated at 28 °C for 5 days. Results were reported in CFU/g (1, 19).
Statistical analysis
A central composite rotational design (CCRD) of the response surface methodology (RSM) technique was adopted to optimize the quality characteristics in fresh green asparagus. The design was chosen for this study because it was suitable for modeling quadratic response surfaces methodology. Two variables (ozone concentration and storage time) known to influence fresh vegetable production were investigated. The ranges of these variables are shown in Table 1. The model terms were calculated for multiple regression analysis, while analysis of variance (ANOVA) was used to assess the model terms' significance. The experimental design and the accompanying statistical analysis were performed using Minitab 19 program. With the same data and choosing the factors considered to be the most important in the quality of the asparagus, as a complement to the design, the desirability function (FD) was built, which determined the ozone concentration and storage time values that optimize the overall appearance, viable mesophilic aerobic bacteria count, and phenol content.
Table 1 Central composite rotational design of the response surface methodology
Treatment | Ozone concentration (codified) | Storage time (codified) | Ozone concentration (ppm) | Storage time (days) |
---|---|---|---|---|
1 | -1 | -1 | 0 | 0 |
2 | 1 | -1 | 10 | 0 |
3 | -1 | 1 | 0 | 30 |
4 | 1 | 1 | 10 | 30 |
5 | -1.4142 | 0 | 0 | 15 |
6 | 1.4142 | 0 | 10 | 15 |
7 | 0 | -1.4142 | 5 | 0 |
8 | 0 | 1.4142 | 5 | 30 |
9 | 0 | 0 | 5 | 15 |
10 | 0 | 0 | 5 | 15 |
11 | 0 | 0 | 5 | 15 |
Results
Table 2 presents the results of total phenol content, overall appearance, and microbiological counts based on the RSM's central rotating composite design treatments. Its behavior was modeled as a function of ozone concentration and storage time.
Table 2 Experimental results applying the central composite rotational design.
Ozone concentration (ppm) | Storage time (days) | Total phenols content (mg/g sample) | Mold count (CFU/g) | Psychrophilic bacteria count (CFU/g) | Mesophilic bacteria count (CFU/g) | Overall appearance (points) |
---|---|---|---|---|---|---|
0 | 0 | 18.73 | 0 | 0 | 6,696 | 9.0 |
0 | 15 | 16.22 | 714 | 68,593 | 31,289 | 6.8 |
0 | 30 | 13.90 | 1,569 | 195,667 | 62,261 | 5.1 |
5 | 0 | 19.78 | 0 | 0 | 2,819 | 9.0 |
5 | 15 | 18.39 | 337 | 28,839 | 10,573 | 7.9 |
5 | 30 | 15.79 | 826 | 128,350 | 27,731 | 7.2 |
10 | 0 | 19.90 | 0 | 0 | 2,613 | 9.0 |
10 | 15 | 18.42 | 78 | 19,267 | 3,814 | 7.9 |
10 | 30 | 16.35 | 260 | 87,495 | 7,538 | 7.4 |
5 | 15 | 18.02 | 404 | 29,813 | 11,205 | 8.1 |
5 | 15 | 18.27 | 385 | 31,964 | 10,176 | 8.1 |
Table 3 presents the models and lack of fit for phenols, overall acceptability, mold, psychrophilic bacteria, and viable mesophilic aerobes counts.
Table 3 Models and lack of fit for phenols, overall acceptability, mold, psychrophilic bacteria, and viable mesophilic aerobes counts.
Variable | Equation | R2 (%) | P value | Lack of fit |
Phenols | y=18.578 + 0.4207 ozone - 0.1239 time - 0.02907 ozone*ozone - 0.001164 time*time + 0.00427 ozone*time | 98.76 | 0.000 | 0.469 |
Overall appearance | y=8.974 + 0.032 ozone - 0.2228*time - 0.00316* ozone*ozone + 0.00187 time*time + 0.00667 ozone*time | 95.05 | 0.001 | 0.996 |
Molds | y=7.9 - 12.61 ozone + 44.64 time + 1.323 ozone*ozone + 0.2226 time*time - 4.363 ozone*time | 99.09 | 0.000 | 0.577 |
Psycrophiles | y=2534 - 3835 ozone + 2345 time + 399 ozone*ozone + 134.3 time*time - 360.6 ozone*time | 99.47 | 0.000 | 0.074 |
Mesophiles | y=7642 - 2599 ozone + 1345 time + 225.5 ozone*ozone + 14.94 time*time - 168.8 ozone*time | 99.51 | 0.000 | 0.054 |
Figure 1 presents contour and response surface methodology of (a) total phenol content and (b) overall appearance for ozone concentration and storage time.
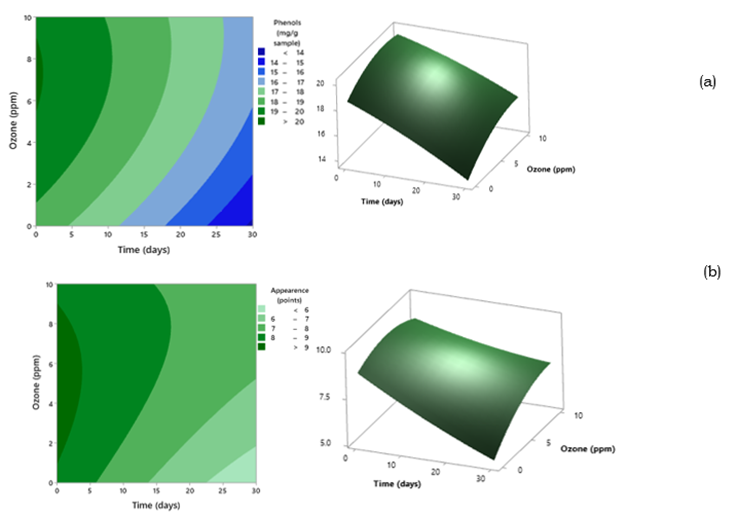
Figure 1 Contour and response surface methodology of (a) total phenol content and (b) overall appearance for ozone concentration and storage time
Figure 1a shows that the initial application with gaseous ozone between concentrations of 6 to 10 ppm generated an increase in total phenols content. Then, a decrease of these compounds was observed until the end of storage, consistently reporting higher values in the ozonated samples (16-17 mg/g sample) compared to the control sample (not ozonated). The analysis of variance showed that the quadratic model presented a significant effect (p<0.05), high coefficient of determination (R2 = 98.76%), and lack of fit p > 0.05, which explains a good fixation of the model data (Table 3).
Figure 1b shows that gaseous ozone concentrations of 6 to 10 ppm and storage time of 20 to 25 days produced the highest overall perception with acceptable levels of 6 to 8 points in the asparagus spears than samples treated at lower concentrations, including not ozonated. The analysis of variance showed that the quadratic model presented a significant effect (p<0.05), high coefficient of determination (R2 = 95.05%), and lack of fit p > 0.05, which explains a good fixation of the model data (Table 3).
Figure 2 presents contour and response surface methodology blanket for microbiological counts of (a) molds, (b) psychrophilic bacteria, and (c) viable aerobic mesophilic bacteria for ozone concentration and storage time.
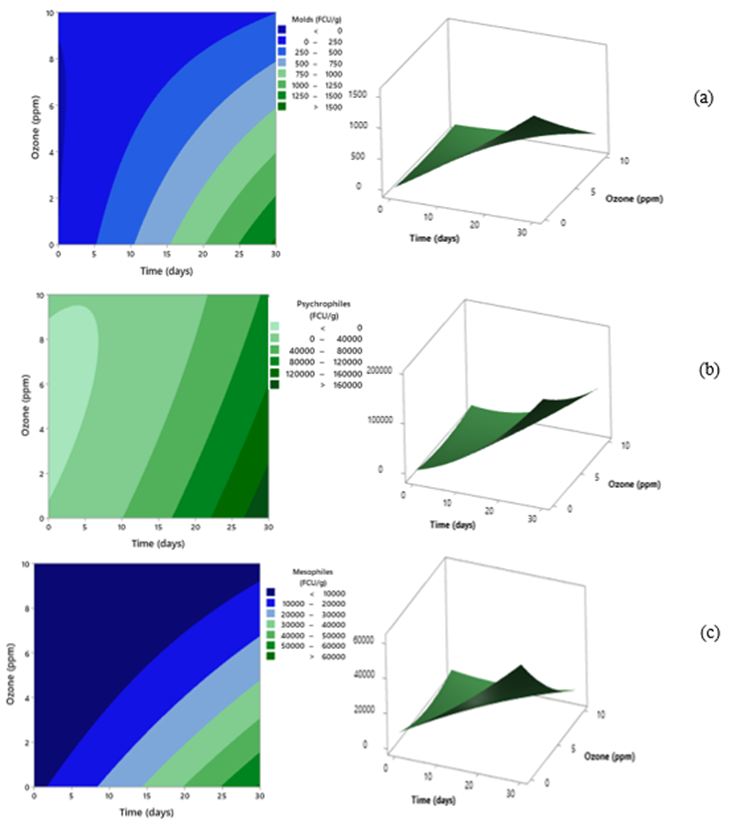
Figure 2 Contour and response surface methodology blanket for microbiological counts of (a) molds, (b) psychrophilic bacteria, and (c) viable aerobic mesophilic bacteria for ozone concentration and storage time
Figures 2a, b, c show that gaseous ozone concentrations of 6 to 10 ppm and final storage (20 to 25 days) generated the lowest microbial counts (5 x 102, 4 x 103, and 1 x 104 CFU/g for molds, psychrophilic, and viable mesophilic aerobic bacteria, respectively) compared, with the samples treated at a lower concentration, including the control. The analysis of variance showed that the quadratic model presented a significant effect (p<0.05) for microbial counts; high coefficient of determination (R2 = 99.09, 99.47 and 99.51%, respectively) and in all cases, a lack of fit p > 0.05, which explains a good fixation of the model data (Table 3).
Figure 3 presents the optimization of quality characteristics in fresh green asparagus.
The contours of the RSM were obtained using the superposition technique; as a result, the region of interest that determines the optimal area corresponded to a concentration of 10 ppm of gaseous ozone up to 25.9 days of storage, indicating characteristics of 16.99 mg/g sample of phenols; 7.61 points in general appearance, and 5.3 x103 CFU/g sample of viable aerobic mesophilic bacteria.
Discussion
Ozone concentration effect and storage time on total phenol content
The results obtained can be explained due to the activation reaction that ozone treatments produce in the defense mechanisms before the onset of oxidative stress and the changes in the activity of enzymes that participate in the synthesis of polyphenolic compounds such as phenylalanine aminolyase (PAL) (20, 21). The increase in phenolic compounds can be attributed to the modification of the cell wall during exposure to ozone; this modification can increase the extraction and release capacity of conjugated phenolic compounds (22). Tabakoglu and Karaca (2018) indicated that storage vegetables at low temperatures with gaseous ozone application can increase the phenols’ content (23). Yeoh et al. (2014) suggested that the increase in total phenols, due to the initial application of gaseous ozone, may produce the inhibition of enzymes such as polyphenol oxidase (PPO) and peroxidase (POD), which often cause oxidation of these compounds in freshly cut fruits and vegetables (24). Gutierrez et al. (2018) associated that fresh-cut leafy vegetables in contact with gaseous ozone played an important role in protecting plant tissues against biotic and abiotic oxidative stress because it promotes the synthesis of phenolic compounds (25).
The positive effect of gaseous ozone treatment on the content of phenolic compounds was also observed in bell pepper (26), green and red bell pepper (27), and rocket (28).
Ozone concentration effect and storage time on overall appearance
The product’s visual quality is important because any color alteration can be recognized as a symptom of senescence. Likewise, there are studies with the application of ozone in the disinfection of fruits and vegetables that do not harm the original quality of the vegetables despite observing slight changes of color. The application of gaseous ozone could prevent the deterioration of the product and increase its shelf life (29). The overall appearance represents all the visible food attributes and can be a fundamental element in purchasing the vegetable (30).
Similar results with exposure to gaseous ozone were found in strawberries (31), lettuce (32), bell pepper strips (33), pomegranate (30), and coriander (34).
Ozone concentration effect and storage time on microbial inactivation
The ozone inactivates microorganisms by the progressive oxidation of vital cellular components because it breaks the cell wall, achieving its disintegration. Gram-negative bacteria (E. coli, viable aerobic mesophilic bacteria, psychrophilic bacteria, total coliforms, etc.) are more susceptible to ozone because they possess thin layer peptidoglycan. An external membrane made of lipoproteins and polysaccharides, which are easily oxidized (35). Yeoh et al. (2014) indicated that microorganisms could be affected by temperature, concentration, duration of exposure to ozone, and biological factors, such as the type and composition of vegetables and the type and microbial load (24). Sheng et al. (2018) mentioned that the effect of ozone could be attributed to the fact that it interferes with the diffusion of nutrients on the surface of the cut vegetable, inhibiting the growth of bacteria, molds, and yeasts (36). Gutiérrez et al. (2018) indicated that ozone treatments effectively extended the shelf life of different vegetables, reporting that ozone treatments between 0.15-5.0 ppm succeeded in reducing the growth of bacteria and molds (25). Variations in the results could suggest that molds, yeasts, and spore-forming bacteria, due to their sensitivity, require increasing ozone concentrations and longer exposure times for inactivation, which is one of the main parameters governing the effectiveness of ozone (37).
Similar behaviors were found in products like lettuce, parsley, and spinach (35), tomato strips (38), fresh-cut lettuce leaves (39), green asparagus (40), lettuce, and green bell pepper (37).
Optimization
The technique of RSM contours superposition was used to optimize the independent variables, such as phenol content, general appearance, and count of viable mesophilic aerobic bacteria. They are considered important for maintaining the quality of green asparagus spears during the logistics and marketing chain and their antioxidant activity (Figure 3).
Conclusions
This research showed that the application of gaseous ozone at 10 ppm was not only a great disinfectant agent, but it was also a functional postharvest technology that maintains the quality characteristics, such as overall appearance and total phenols content of the minimally processed green asparagus spears, allowing a shelf life of 26 days at 1 ° C.