Introduction
Several imaging techniques have been developed based on the detection of X-rays and γ-rays taking advantage of their capabilities to penetrate matter. The images generated by transmission techniques are used in different fields such as medicine, in diagnostic techniques, in civil construction, with thickness and density gauges and in the detection of nuclear materials [1,2], just to mention three cases. When access to both sides of the sample is not possible and therefore the use of γ-ray transmission becomes impossible, techniques based on Compton scattering emerge as an alternative. Different methods have been developed, including arrangements that allow for the formation of 3D images [3] and arrays based on Time-of-Flight techniques [4,5]. The present work is focused on the Compton Camera, an existing imaging device whose working principle is based on the Gamma-ray Compton Backscattering (GCB) technique [6].
The Compton Camera uses the scattered radiation -via Compton effect- by the sample to construct a bi-dimensional map that represents the volumetric matter distribution of the sample within the field-of-view of the device. It has been successfully tested in different situations: thickness measurements, location of buried high-density objects in sand, inspection of hidden objects behind metallic walls and in the diagnosis of metallic surface [7-9]. In order to increase the range of applicability of the Compton Camera, it is mandatory to improve the quality of the images.
In this work, some changes to the original device are proposed and evaluated using numerical Monte Carlo simulations. The interest is focused on intrinsic noise reduction.
Physical principie of the Compton Camera
The working principle of the Compton Camera is described in detail in Ref. [7] and following Fig. 1 it can be summarized as follows. A point-like 22Na source provides positrons which after emission annihilate generating two 7-rays of 511 keV that travel in opposite directions. The detection of photon y1 by the Position Detector (PD) is used to establish the flight direction of y2 going to the sample. If y2 is scattered and subsequently registered in the Backscattering Detector (BSD), this detector, working as a trigger, activates the collection of the event in the PD: when an energy deposition in the BSD (trigger) occurs a user-defined time window is opened, during which the associated electronics records information related to the position of detection in the PD.
The collection of events registered in the PD builds a two-dimensional matrix representing the distribution of the backscattering probability in the sample within the Camera's field of view. The practical usefulness of the Camera arises from the fact that the backscattering probability, and the intensity in each pixel of the image, depends on thickness, density and multielemental composition of the sample. The contrast is defined by the relative difference in intensity of counts between pixels associated to different regions with different concentration of electrons each one.
Intrinsic noise of the device
In the ideal case, any 7-coincidence between BSD and PD should be only produced by the detection of photons generated in the positron annihilation following the operation principle described before. In this work, the "noise" of the device refers to coincidence events that contribute to the image but arise from physical events that do not follow the working principle described above. In the absence of a sample, i.e., with the imaging device by itself, 7-rays will interact with the different parts of the device randomly activating coincidences between BSD and PD. The events coming from these aleatory coincidences will be referred to as the "intrinsic noise" of the apparatus.
There are different processes involved in the production of random coincidences. When using a 22Na source, one of the main source of intrinsic noise is the presence of 1275 keV γ-rays because they do not have any angular correlation with the 511 keV 7-rays. Figure 2 illustrates two possible cases of coincidence events that contribute to intrinsic noise of the apparatus: (a) Coincidence with the detection of a 1275 keV photon by the BSD. (b) A photon, after a Compton scattering in the BSD, is absorbed in the PD. In this latter case, there are not even two independent γ-rays.
The intrinsic noise adds a non-structured background all over the image, diminishing the contrast, that is, lowering the possibility of distinguishing features in the built pattern. For this reason, reducing the probability of counting aleatory coincidences is mandatory in order to increase the image quality and to extend the possible applications of the device. There are several techniques available that could be used to reduce the number of aleatory coincidences: flight time measurements (not possible for the present device), spectroscopy methods, time coincidences techniques, shielding systems and imaging processing [6]. This work is focused on reducing the number of aleatory coincidences modifying the shielding system of the device.
The working principle of the camera is based on the directional correlation of the annihilation photons. In order to estimate the response of the actual device in case of having an ideal radiation source, a point-like γ-ray source which emits only two 511 keV 7-rays traveling in opposite directions, a simple situation is studied, continuing the analysis presented in Refs. [7,9]: two side-by-side metallic plates placed with the contact line at the center of the field-of-view of the camera. In this situation, the interest is focused on the non-uniformity in the image produced by the thickness difference of the plates. Figure 3 shows the sketch of the setup, the experimental (using a 22Na source) and simulated (using the ideal source mentioned above) images and the projection onto the y-axes of the images. The simulation of the Compton Camera with the ideal γ-ray source was developed using Geant4 [10].
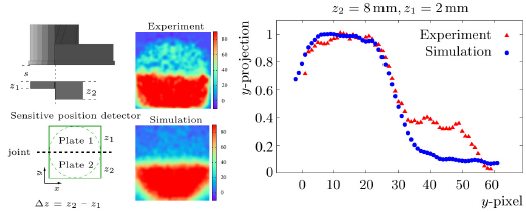
FIGURE 3 Sketch of case of study. Experimental and simulated images; the color scale is defined by the counts per pixel in the image, from lowest intensity in blue to highest intensity in red. Projection onto the y-axis for experimental and simulated data, normalized to maximum value of counts per pixel in the region defined by the thicker píate.
The 2D images shown in Fig. 3 are the result of subtracting pixel by pixel the reference image (image obtained when z 1 = 2 mm, z2 = 2 mm) from the total image (image obtained when z1 = z2 = 8 mm) and then applying a smoothing procedure using a Gaussian filter [11] with a = 1,3 pixels. The simulated image includes the redistribution of counts per pixel using a bi-dimensional Lorentz distribution with 5.9 pixels width, following Ref. [9], in order to reproduce photonic transport phenomena. The 2D images in Fig. 3 show two regions, the higher and lower intensity zones are associated to sectors with larger and smaller thickness, respectively. From the graph of the y-projection, there is clearly two intensity levels, the higher intensity level is associated to larger thickness and the lower intensity level is the reference level; the contrast is defined by the difference in counts between higher and reference intensity levels. The contrast in the image is notably increased when using an ideal source of two opposite 511 keV 7-rays, what leads to the conclusion that it is important to use a source that emits as few uncorrelated gamma rays as possible in order to reduce the number of random coincidences. If the number of random coincidences decreases the reference level (with respect to which the contrast is measured) becomes lower, a fact that increases the contrast.
Proposal
The non-correlated 7-rays from the 22Na source are an important noise source because they contain no useful position information. Because of this reason, a new positron source is required and a 68Ge source seems to be a good alternative [12]. 68Ge produces a very low number of photons without angular correlation, around 3 % compared with 99.9 % of 22Na, and has a half-life of 271 days, large enough to be considered for imaging applications.
On the other hand, in order to reduce the contribution to intrinsic noise of events in which both detectors register no independent γ-rays, as in the case shown in Fig. 2 (b), another part suitable for modification is the shielding system. The proposal consists in replacing the geometrical shape of the lead shielding: instead of a cone, a cylinder. The cylinder should cover the whole of the upper surface of the BSD as shown in Figure 4. The effect of including collimators made of tungsten for both PSD and sample is also studied.
In addition, the new shielding system provides the possibility to vary the PSD vertical position. PSD mobility permits to vary the irradiated region of the PSD, i.e., it would be possible to control the number of pixels that comprise the image.
By reducing the number of coincidences between photons with no useful correlation between them, the dead time produced by the trigger signals generated by them will be reduced too. Therefore, the detection system will analyze more signals with spatial useful information in the same acquisition time and therefore an improvement of the image is expected.
Results and discussion
In order to estimate intrinsic noise of the Compton Camera a very simple situation was simulated, that in which the camera stands by itself, i.e., within an environment of only air and without any test sample. In presence of a sample, the noise should be different to the no-sample situation due to the additional possibilities for aleatory coincidences. Furthermore, the noise in a sample situation will depend on the specific sample and its characterization is beyond the scope of this work, although it is expected that reducing intrinsic noise will also reduce the noise in a situation with sample. The intrinsic noise of the camera is determined by counting the number of aleatory coincidences in a no-sample situation. Figure 5 shows the number of entries in the PSD, in coincidence with the BSD, as a function of the shielding thickness that covers the upper surface of the BSD, using a 68Ge source, for three different configurations of the collimation system: (i) removing PSD and sample collimators, (ii) removing just PSD collimator and (iii) including both, PSD and sample collimators, see Fig. 5 (right side). The number of simulated radioactive decays in each case was the same and the number of PSD entries was normalized to the value that resulted when using the actual geometry of the Compton Camera (Fig. 4, left side).
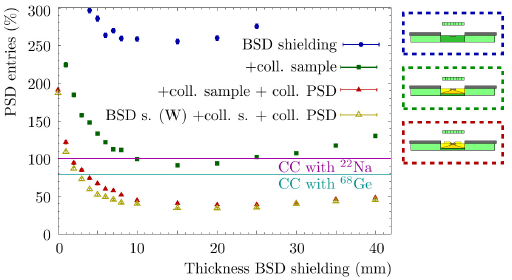
FIGURE 5 Number of entries in the PSD in coincidence with the BSD as a function of the thickness of the BSD shielding, placing the Compton camera in air without test sample. In magenta, the intrinsic noise of the actual camera and, in cyan, intrinsic noise the actual geometry using a 68 Ge source. Figures on the right illustrate the geometrical configuration used in each case with a 68 Ge source, including BSD shielding (Pb and W 'materiais were tested), the collimator to the sample (coll. sample) and collimator to PSD (coll. PSD). Each point was normalized to the intrinsic noise of the actual Compton Camera configuration.
According to Fig. 5, the intrinsic noise decreases by approximately 20 % with a 68Ge source and the actual shielding-collimation system. In addition, the number of aleatory coincidences decreases with increasing thickness of the BSD shielding for all cases until it reaches a minimum when the thickness is about 15 mm (~ 60 % of additional intrinsic noise reduction). In order not to increase the weight of the instrument too much, it is recommended to use a BSD shielding thickness between 6 and 7 mm with which an additional intrinsic noise reduction of ~ 45 % is achieved. Using a tungsten BSD shielding, the intrinsic noise decreases an additional 10 % with 7 mm thickness respect to lead shielding with the same thickness; if thickness of the BSD shielding is 1 cm or larger the noise reduction is statistically equal for tungsten and lead shielding.
On the other hand, the intrinsic noise decreases when PSD and sample collimators are included (red and yellow points in Fig. 5). PSD and sample collimators are responsible for collimating the beam and, in addition, decreasing the number of gamma rays that reach the BSD directly. For this reason, it is recommended to use PSD and sample collimators in addition to the BSD shielding proposed in this work.
Summary and perspectives
The simple change to the shielding system of the BSD of the actual device already achieves a clear intrinsic noise reduction of the Compton Camera. The suggested configuration in this work for the shielding-collimation system consists of PSD collimator, sample collimator and a lead shielding block with 7 mm thickness covering the upper surface of the BSD. A 68Ge source is a good candidate replacing the 22Na source.