1. Introduction
The remarkable progress of computers and software, miniaturization and better detector efficiency lead to continuous improvement of X-ray CT, especially in reducing radiation doses in patients. The Monte Carlo method is considered to be a powerful tool to simulate a physical phenomenon of radiation interaction with the matter and obtain a precise estimation of several physical parameters of a real situation (1, 2).
The Monte Carlo method used to simulate the radiation interaction with matter allows understanding the energy delivered into the volume of interest, and to know the dynamics of the particles produced, how are their trajectories, the mean free path and the maximum range in relation to the properties of the emitting radionuclide. In nuclear medicine application the positron-emitting radionuclide is attached to a particular molecule and later is incorporated into the body through inhalation, injection, or ingestion (3, 4).
Positron Emission Tomography (PET) is a technique in the field of nuclear medicine where a patient or a living creature who has been previously administrated small quantities of radioactive material through inhalation, injection or ingestion is metabolized into a tissue or organ and then the positrons emitted from the nucleus interact with electrons of the medium producing annihilation gamma photons 5 The diagnostic image is formed by the emission of gamma photons in opposite directions that reach the PET detector array. Having an array of detectors configured ring-shaped, reconstructs the volume image based on distributed positrons and therefore in vivo measurements can determine the location and size of the lesion.
PET technique allow the detection of vascular and physiological diseases of the heart in real time, detect pathologies in early stages in people with Alzheimer's disease, Down Syndrome, etc. In addition, PET can be combined with other techniques such as computerized tomography CT and make more accurate diagnoses by combining the metabolic image with the anatomical image 6. Before interacting with the electrons, positrons delivered its energy to the medium through collisions and when they have reach an energy of 1.022 MeV they annihilate with electrons to form two photons of 511 keV, that traveling in opposite directions, about 180 ±0.5 degrees 7. The mean free path of positrons is responsible for the resolution of the diagnostic image. The lower the path before annihilation, volume interactions occur will be smaller, improving image quality (8, 9). See Figure 1.
The spatial resolution is also improved by the sensitivity and size of PET detectors. Annihilation processes can be lost in the detection if they fail to reach the detectors or whether electronics of coincidence window record an event as no true. Basically, when achieve lower path before annihilation, it will have information more precise about spatial location of the lesion and the outline of the volume of interest will delimit with more accuracy, allowing discard normal metabolic processes compared with potential tumor growths (10, 11).
The radioactive isotopes used in the PET are produced in a medical cyclotron in which usually a high energy proton beam is fired into an appropriate target. To produce 18F and 18O water target undergoes the nuclear reaction 18º(p,n)18F, indicating that a proton (p) is absorbed and a neutron (n) lost. The most common cyclotron configuration for PET is the negative ion design, which consist of two "dee" electrodes, which are about 1 m in diameter. Subsequently, radionucleide is taken to a radiochemistry laboratory, where they are combined with a chemical substance called tracer for latter administration. The mainly used radiotracers are shown in Table1, (12.
The purpose of this work was to determine the behavior of positrons from different emitting radio-nuclides with respect to its interaction with a volume of soft tissue, performing a statistical mean free path and the maximum range of the particles in order to assess what kind of radionuclide from the point physically it is more convenient according to the size of the lesion. In this work was used Geant4 Monte Carlo simulation code 13.
2. Materials and Methods
The work was carried out using the Geant4 code in the model designed for the Monte Carlo calculations with spheres of 10 mm-radius, considering that sphere radius must be greater than maximum range of positrons used in nuclear medicine for water, Assuming that the most maximum range isotope used in nuclear medicine is 15O with 8.2 mm and to ensure that the origin of all annihilation photons are within the water sphere. The materials of elemental spheres were chosen: water, muscle, adipose tissue and compact bone tissue, the components by weight they are taken from 11.
An isotropic radioactive source with spherical shape of 0.2 mm of diameter is located in the center of the sphere with 10.000 initial radioactive nuclei. Positrons interact with the matter creating io-nizations and delivering energy until to produce the annihilation gamma photons. Subsequently, the simulation data are sent in ROOT software for processing and analysis of multiple interactions.
In the Figure 2a it shows the simulation with 10 decays, in the Figure 2b it shows the simulation with 3 only decays of 18F in water with the aim of seeing the trajectories of positrons (broken lines) and gamma photons (straight lines).
List physical reference Geant4 LBE 5.3 was used because allows information about the decay processes at the same time that positrons are emitted. With this feature we can know the history of events during the positrons interactions until the positron annihilation with electrons can be estimated 13.
Results for various positron emmiting radionuclides and several tissues were compared in relation to energy deposited into sphere mass to determine the dose absorbed by the tissue 4.
3. Results
In the Figure 3 it show the average range of positrons emitted from 18F, 11C and 15O, we might show at the center of each sphere the intensity and the length lines that represent the region where positrons delivered its energy based on initial energy of positrons. Below are shown the maximum length and average distance calculated through Gaussian fitting.
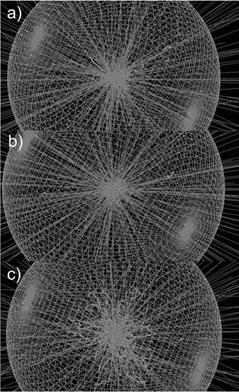
Figure 3 Simulation of several positron sources into a water sphere and its maximum length a) 18F=2.4 mm b) 11C=5.0 mm c) 15O=8.2 mm.
As the statistics of the data obeys a Gaussian distribution, the Full Half Maximum FWHM= = 2.355
where
is the standard desviation. The FWHM corresponds to average range of positrons and 4.291
corresponds to maximum length reached by positrons 15. In the Figure 3 can be seen the difference of trajectories of positrons for each positron emitting isotope.
Simulation data are stored in ROOT software 16) and are shown the results in following histograms, see Figure 4. In Figure 4 shows the largest frequencies are given for shorter maximum lengths. It is clear to see that as much energy delivered by positrons in a small range and the largest contribution is obtained by 18 F.
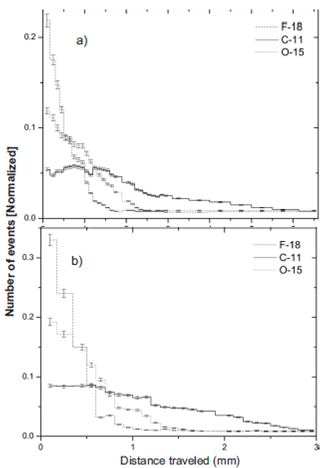
Figure 4 The maximum distance traveled by positrons in a sphere of 10 mm radius. (a) water (b) Compact bone.
The relation between maximum traveled length in water sphere and compact bone for 18F is 1.880, 11C is 1.673 and 15O is 1.920, this meaning that there is a relationship between tissue density and maximum length. A higher density of tissue decreases the maximum length. In Table 2 shown the maximum lengths in different tissues.
In Figure 5 the functional relationship between the energy of positrons corresponding to the three radionuclides simulated 18 F, 11C and 15 O and the maximum length of the positrons have a linear behavior. Allowing predict the range of positrons for any me-dium in terms of energy. Taking account decay time for each radionuclide and the relationship between the number of positrons simulated and the activity was evaluated the absorbed dose into the volume. The results are shown at the Table 3.
This work can be extended to situations where knowing the volume and density of a tumor the radiopharmaceutical covers fully the region of interest not exceeding the amount of radioactive material in order to obtain an accurate diagnosis reducing the absorbed dose to the patient and the workers that delivered it. According to the results shown in Figure 5 it can be determined that the range of the positrons are almost linear with respect of their energy and the maximum displacement occurs in adipose tissue.
The absorbed dose into the volume depends of initial energy of positrons. The 18F due to have lowest energy compared with 11C and 15O allows reduce the absorbed dose in a tissue. See Table 3.
In situations where its known the volume and density of a tumor, the radiopharmaceutical chosen must to cover fully the region of interest not exceeding the amount of radioactive material in order to obtain an accurate diagnosis reducing the absorbed dose to the patient and the workers that delivered it.
4. Conclusions
One of the important factors in the spatial resolution of a PET system is the maximum length of positrons, the shorter the range the higher the resolution. According to the results 18F is 48% shorter than 11C and 31 % shorter than 18O, which allows to conclude that 18F offers a better alternative for the study of tumors of small volumes, this is one of the reasons for the widespread use of FDG. The other isotopes are very useful for studies involving larger volumes such as cardiac perfusion and brain diseases. See Table 1.
Using different positron emitters is established that type of radionuclide it is needed for each kind of lesion and its volumetric configuration. The Monte Carlo method can accurately assess the maximum and averages ranges for any tissue and generally a lot of materials that are defined in the database Geant4 simulation code or they can also be defined in the code starting from its elemental chemical composition.