INTRODUCTION
Starch is a natural polymer obtained from renewable sources that is biodegradable and available in abundance. In many industrial applications, the native starch has some disadvantages such as low solubility, rapid thermal decomposition, high retrograde and low resistance to shear. To overcome these disadvantages and obtain better properties, the starch can be modified by physical, chemical and enzymatic processes.[1-3]
In recent years, the microparticles (MP) obtained from polysaccharides have become the subject of several investigations, with important applications in the field of microcomposites, packaging, cosmetics, biomedical applications, colloidal stabilizers and the oil industry, all of which can be attributed to the fact that they provide a high ratio of surface area / volume, which favors phenomena of mass and energy transfer, thereby helping to resolve a variety of problems in different industries. [4,5] The starch micro-particles SMPs have been prepared by various methods such as ultrasonication, [6]Field Effect Scanning Electron Microscopy (FE-SEM high pressure homogenization, emulsification, [7] acid hydrolysis, [8] microprecipitation, [9] extrusion, [10] and a combination of hydrolysis and ultra-sonication; [5,11] the particles obtained are under 500 nm in size.
Graft copolymerization (Figure 1) is a method for modifying the properties of polymers, particularly when vinyl monomers are used; a product with better physical and chemical properties is obtained, in agreement with the nature of the monomer. Free radical grafting methods are the most commonly employed. [12,13] Microwaves were born as a potential source of thermal energy that constitutes a new method for heating materials. [14,15] Microwave-assisted free radical polymerization is being implemented as a current method in polymer science to achieve grafted materials with or without an initiator (catalyst). [15-19]"ISBN" : "0141-8130", "ISSN": "01418130", "PMID" : "20951725", "abstract": "Polyacrylamide grafted starch (St-g-PAM The aim of the present article is to prepare potato starch microparticles by combining the hydrolysis and sonication methods, and to enable the copolymerization of acrylamide (monomer) graft through the chains of the potato starch micro-particles using microwaves. Also reported are the physicochemical characterizations of starch, starch microparticles and microparticles grafted with acrylamide: Several analytical methods were employed: FTIR, SEM, XRD and TGA.
EXPERIMENTAL
Materials
The potato tubers (Solanum Tuberosum) of the "pastusa" variety were purchased in a local market in Tunja, Boyacá, Colombia. The sulfuric acid 98% was purchased from J.T. Baker; the acrylamide (monomer) was obtained from Merck, Germany; the sodium bisulfite, potassium persulfate and acetone were purchased from Sigma Aldrich and used as received.
METHODS
Extraction of potato starch and preparation of microparticles
Starch from the potatoes obtained (Solanum Tuberosum) was extracted by means of the process described by Otárola et al., [20] Novelo and Betancur. [21] The potatoes were peeled, cut into small slices and placed in sodium bisulfite at a concentration of 1500 mg/mL, at a ratio of 1: 3 (w / v) for 30 min. The pieces were liquefied for 2 min and the mass obtained was placed in an Erlen-meyer flask with sodium bisulfite at 1500 mg/L, with a ratio of 1: 1 (v / v). This starch mixture was filtered to remove the fiber, and the filtrate was allowed to stand for 8 h at 4° C. Then, the supernatant liquid was removed, and the starch suspension was washed repeatedly with distilled water, the last wash being centrifuged for 12 min at 2500 rpm to obtain the starch. Subsequently, the starch obtained was dried for 12 hours at 50° C in an oven, finally packaged in a hermetic polyethylene bag for later use.
The starch microparticles were prepared following the procedure described in the work of Mohammad Amini and Razavi. [5] Simultaneous treatments of acid hydrolysis and sonication for the suspension of starch were carried out as follows: 50 mL of potato starch suspension with a concentration of 15% (w / w) prepared in 3.16 mol/L sulfuric acid was immediately put through sonication with an ultrasonic device (Cole - Par-mer ultrasonic processors) with a nominal power of 20 KHz 350 W, which was maintained at 40 ° C and sonicated at 80% amplitude for 30 min, after which the solutions were centrifuged at 10.000 rpm for 10 min and were washed repeatedly with distilled water. The samples were lyophilized.
Preparation by microwave irradiation of acrylamide grafted to starch microparticles (SMP-g-PAM), employing potassium persulfate (PPS) as free radical generator.
The SMP-g-PAM copolymers were obtained by coupling the method reported in the study of Singh et al., [22] 0.1 g of starch microparticles being dissolved in 5 mL of distilled water. The required amount of acrylamide (0.2-0.7 g) was solubilized in 1.5 mL of water and added to the starch microparticle solution, stirred well and transferred to the reaction tube (10 mL) and an amount of potassium persulfate (PPS) (0.01-0.040g) was added. The tube was inserted into a microwave oven (CEM, Discovery Synthesis) and the microwave oven was activated at ~ 3 W, to maintain a constant temperature of 50° C during the reaction time (1-7 min). After completing the reaction, the vessel was transferred to an ice water bath and the gel remaining in the reaction vessel was placed in sufficient acetone. The precipitate obtained was taken and dried at 60° C for 12 h. The percentage of grafting i.e the percent increase in weight of starch microparticles upon grafting after complete removal of the to be grafted acrylamide monomer. The success of a grafting is usually measured in terms of the grafting efficiency the fraction of the monomer that is grafted onto the polymer vs. that which is either unchanged or is consumed in side reactions such as homopolymerisation. By means of the following equations (1 and 2) the percentage and efficiency of the graft were calculated respectively.
SEM characterization
With the use of a scanning electron microscope from JEOL JSM-5900-LV (Japan), samples of starch, starch microparticles and the SMP-g-PAM (MCI-2) sample were studied for their morphology, the samples being covered beforehand with a thin layer of gold using the ion spray method.
Analysis of Fourier-transform infrared spectroscopy
The spectra of starch, starch microparticles and SMP-g-PAM sample (MCI-2) were carried out in solid state, employing a spectrophotometer (FIR Spectrum 400 by Perkin-Elmer, USA) between 400 and 4000 cm-1.
RESULTS AND DISCUSSION
Extraction of potato starch and microparticles preparation
The starch weight content of the "pastusa" variety of potato (Solanum tuberosum) obtained after the extraction treatment was 15.35%, with a moisture content of 6.9% and an average particle size of 25µm determined by SEM (Figure. 5a.), a result similar to that reported by other authors. [23]
Regarding the appearance of the suspension of potato starch with 15 wt.% solid content, as the sonication time is increased, the transparency improved and the fraction of sedimentation decreased. After 30 min of simultaneous ultrasound and sulfuric acid hydrolysis treatment, the starch suspension became totally transparent without any change to the solution fluidity, which is a good reference of a decrease in size of the starch granule[6]Field Effect Scanning Electron Microscopy (FE-SEM. The microparticles observed by SEM show agglomerations, and no individual particles can be observed. According Mohammad & Razavi, [5] particles smaller than 100 nm can be produced with simultaneous treatments of ultrasound and sulfuric acid hydrolysis. The reduction in size was determined, placing two grams of lyophilized particles in 5 mL of distilled water at 25° C, stirring well and then passing the resulting solution through a Millipore filter of 0.2µm (200nm), where it was observed that all the solution had passed through the filter without leaving particles stuck to the filter surface, suggesting that the particles obtained have a size smaller than 200 nm.
Preparation by microwave irradiation of SMP-g-PAM.
The SMP-g-PAM copolymers were prepared by microwave irradiation, different copolymer samples were prepared with various potassium persulfate (PPS) concentrations, acrylamide concentrations and reaction times. To maintain a constant temperature of 50° C in the reactions, the microwave equipment was programmed to ~ 3W. The reaction conditions are show in Table 1. In which it is observed that the maximum percentage of graft (613) is get with the use of the highest amount of acrylamide that was used, while the maximum yield or efficiency of the reaction (100%) was obtain with the longest reaction time (7 min), these results were expected due to the higher concentration of the monomer, greater grafting can be generated, and as the reaction time is longer, the complete conversion of the entire graft monomer can be reached.
Effect of monomer concentration.
The effect of the concentration of acrylamide (monomer) on the % grafting was analyzed in the amounts of 0.2-0.7 g with 0.025 g of potassium persulfate and 0.1 g of SMP. Increasing the concentration of monomer led to an increase in the % efficiency and % grafting. With a chosen concentration of 0.3 g, at a variable power (~ 3 W), an irradiation exposure time of 3.0 min and a constant temperature 50° C, an efficiency of 79.3% was observed, which is almost twice as high as that obtained when 0.2 g of acrylamide were used; for other concentrations of acrylamide, little increase in % efficiency of the reaction was observed. The increase in % graft is due to the higher availability of acrylamide in reaction medium, Figure 2.
Initiator concentration K2S2O8 effect.
In a concentration range of 0.010-0.040 g of initiator, 0.3 g of fixed acrylamide and 0.1 g of SMP, the effect of the variation of the initiator was evaluated. It was observed that the percentage of graft and the efficiency increased with the increase in the concentration of the initiator up to 0.035 g; at a higher concentration there is a decrease in the % graft, which is due to the accumulation of free radicals, which implies the rapid termination of the growing chains and hence of the graft. This is an indication that, with the use of small amounts of initiator and longer reaction time, high percentages of grafting can be obtained, as can be seen in Figure 3. This fact can also be explained as due to the formation of polyacrylamide homopolymer.
Effect of microwaves reaction exposure time.
The effect of microwaves reaction exposure time on grafting was studied in a time range from 1-7 min with fixed concentrations of SMP (0.1 g), acrylamide (0.3g) and persulfate (0.025g) at a constant temperature, observable in Figure 4. The % G increased with the time of exposure to the microwaves, which is due to a greater availability of energy, resulting in a greater generation of free radicals in the SMP chains; similarly, there was an increase in the percentage of efficiency. During 3 min reaction time, the efficiency percentage increased linearly, hence the increase was slower, reaching a maximum of 100% efficiency at 7 min. The percentage of grafting presented the same behavior as the efficiency, the maximum percentage obtained was 315% at 7 min of reaction.
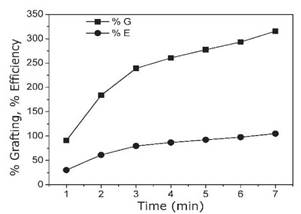
Figure 4 Effect of reaction time on % G and% E using 0.3 g of monomer, 0.1 g of SMP, 0.0250 g of PPS, temperature 50 °C.
These results present a higher degree of graft than those reported by Singh et al, [22] which may be due to the different type of starch being used; furthermore, being in the form of microparticles, the starch has a greater surface area to interact with the reagents and yield a higher graft percentage. Microwaves benefit the percentage increase of the grafted monomers and diminish the reaction times. [24,25]
SEM characterization.
The micrographs of native starch (a), starch microparticles (b) and SMP-g-PAM (MCI-2) co-polymer sample were observed in the figure 5. The native starch particles evidently show a smooth surface and round shape, with sizes ranging from 9 to 100 µm. SEM observations showed that the treatments of acid hydrolysis and ultrasound induced granule disturbance of the starch are due to chemical and mechanical changes to the granule surface, showing a destruction of the crystalline zones. Figure 5b shows the micrograph of the particles resulting from the effects of sonication and acid. Moreover, the mechanical effect and / or the hot spots of the ultrasound increase the action of the acid, providing open conduits, disrupting the growth of the crystalline rings and the hilum of the granules. The SMP-g-PAM (MCI-2) copolymer sample shows morphological changes as a result of the grafting of PAM chains in starch microparticles. Therefore, the morphology of the starch microparticles is lost after grafting and is transformed into particles with well-defined morphology. The micrograph of copolymer shows an increase in particle size and the formation of porous cavities, craters on the surface of the starch microparticles attributable to the successful grafting of the acrylamide chains.
Analysis of Fourier-transform infrared spectroscopy.
Figure 6 displays the Fourier transform infrared spectra of native starch (a), starch micropar-ticles (b) and grafted copolymer (MCI-2) (c). The native starch and starch microparticles register a band at 3321 cm-1 attributed to the stretching vibrations of hydroxyl group (O-H), a reduced band at 2924 cm-1 due to the C-H stretching vibrations. The peaks at 1021 cm-1 and 1151 cm-1 registered stretching vibrations of C-O-C. For SMP-g-PAM (Figure 4c.) showed a band at 3334 cm-1 and a shoulder peak at 3189cm-1 attributed the O-H stretching band of the hydroxyl group of starches and N-H stretching band of the amide group of acrylamides that overlay each other. A small band at 2939 cm-1 is assigned to the C-H stretching vibration; the appearance of strong absorption bands at 1657 cm-1 and 1613corresponds to C=O and N-H amide stretching vibrations respectively. The grafted product showed one more additional peak at 1413 cm-1, which is due to the C-N stretching. In comparison to the sample of starch microparticles, the appearance of these new bands in grafted starch microparticles evidence the successful grafting of PAM chains onto the chains of potato starch microparticles. These peak positions were similar to those reported for grafted potato starch, corn starch, Phaseolus aco-nitifolius starch, maize starch and cassava starch. [17,19,25-27]acrylamide could be efficiently grafted onto potato starch under microwave irradiation and for the grafting 02 removal from the reaction vessel was not required. Under optimal conditions, grafting and efficiency observedwere 160% and 89%, respectively. Grafted starch was characterized by using Fourier transform infrared spectroscopy (FTIR
XR diffraction study.
Figure 7 displays the wide-angle X-ray diffractograms obtained for native starch (a), starch microparticles (b) and grafted copolymer (MCI-2) (c). XRD patterns of the native starch exhibited define diffraction peaks at 5.8, 15.1, 17.3, 19.9, 22.4, and 24.3, 2-theta angles, which are typical of the starch granules of semi-crystalline, this being attributed to the amylopectin fraction that constitutes the starch granule. Conversely, the SMP diffractogram (Figure 7b) shows a wide halo from 10° to 35°, related with amorphous compound. The SMPs are totally amorphous, due to the starch having completely lost its crystallinity during the combined processes of acid hydrolysis and continuous sonication. These results match with other studies that show that ultrasonication disturbs the crystalline fraction of native starch [4,6,11]structural and thermal behavior of starch nanocrystals (SNCs The SMP-g-PAM (MCI-2) copolymer (Figure 7c.) also shows a halo between 10 and 45 ° similar that of starch microparticles, though with a greater intensity due to microwave heating and grafting of acrylamide. The results show that the starch microparticles amorphous region increased following graft copolymerization.
TGA characterization.
Thermogravimetric analysis in an inert atmosphere provides a method for assessing the thermal stability of experimental polymers for their industrial applications. The TGA curves of native starch (a), starch microparticles (b) and grafted copolymer (MCI-2) (c) are present in Figure 8. The starch essentially shows two weight loss zones with a characteristic thermogram within 20° C and 550° C. The first weight loss is between 30° C and 170° C, this is attributed to a small quantity of water present in the sample. The major mass loss is found in the second region (250 °C -320 °C) and was due to degradation of the starch chains. The starch microparticles showed three stages of weight loss at 27 °C, 120° C, 170° C, the large mass loss was found in the temperature range from 120° C - 270° C, values lower than those found in native starch, this is due to the loss of crystallinity as a consequence of the treatments used to obtain them, in addition this fact indicates that starch microparticles have a high number of hydroxyl groups on their surface and it is through them that the thermal degradation start [28,29]. The SMP-g-PAM presents a first weight loss of 11.43% around 70° C, a second weight loss of 21.22% at 271° C and a big loss of 38. 5% at 370° C, a higher temperature than the SMP, due to the grafted PAM chain in the starch microparticles backbone. These results indicate that the grafted copolymer has a higher thermal stability compared to starch microparticles.
CONCLUSIONS
Starch microparticles were prepared effectively by simultaneous treatment with ultrasound and sulfuric acid hydrolysis. The starch microparticles obtained were modified chemically through microwave irradiation synthesis, employing a low quantity of potassium persulfate. It was found that the percentage grafting was high, with 0.7 g of monomer, 0.025 of PPS to an exposure time of 3 min and 50 °C of reaction temperature. The starch, starch microparticles and grafted co-polymer were characterized by various analytical techniques. The semi crystalline character of starch was disturbed by the simultaneous application of ultrasound and sulfuric acid hydrolysis treatment, which was confirmed by the study of x-ray diffraction. On the other hand, the SMP-g-PAM showed increased thermal stability, due to the grafting of acrylamide chains, the modification of the starch microparticle backbone and was confirmed by means of FT-IR and TGA analysis.