1 Introduction
The transformation of molecules derived from biomass (starting materials or building blocks to produce chemicals) has been recognized in recent decades as the key to converting biomass into fuels and chemical products. Within the chemical processes, a very important approach for the conversion of biomass is through the transformation of platform molecules. These building blocks were selected by researchers and comprise a series of compounds that can be easily transformed into other products with high added value. The derivatization of compounds from biomass, is a key step in the valorization of the chemical products [1-2].
One of these building blocks is furfural, an important non petroleum based and chemical feedstock. Several procedures were redefined to obtain valuable products through clean processes and recyclable solid catalysts from furfural, that include the reduction of furfural to furfuryl alcohol and tetrahydro-furfuryl alcohol, the preparation of furoic acid from furfural via oxidation reaction and the furan synthesis via decarbonylation of furfural using a Palladium catalyst [3].
Although, there are numerous heterocyclic compounds containing furfural as substructure, processes for their synthesis, have in general a high E factor, the reaction conditions are rigorous and the yields and selectivity of the reactions are very low. Likewise, the synthesis of these compounds requires the use of toxic reagents and large quantities of reaction solvents [4].
For this reason, our research group has been dedicated during several years to the study to the synthesis of heterocycles through more environmentally friendly processes that include: reactions in the absence of solvent and the use of recyclable hybrid materials based in heteropolyacid (Keggin, Wells-Dawson and Preyssler type) [5].
The main objectives of our research group are to design new materials for sustainable organic synthesis and generate heterocycles with proven or potential biological activity, containing in their skeleton substructures obtained from the building blocks present in biomass (furfural, 5hydroxymethylfurfural, levulinic acid, cinnamic acid, etc) [6].
Some of our recent works in the design of new materials include: vanadium substituted Keggin poly-oxometalate incorporated in poly (acrylic acid-co-acrylamide) polymer [7], bifunctional mesoporous catalysts based on Preyssler heteropolyacids [8], Preyssler heteropolyacids immobilized on magnetic composites [9] and nanoparticulate silica with organized multimodal porous structure impregnated with 12-phosphotungstic acid [10]. In general, the new materials are used in the suitable synthesis of heterocycles for example dihydropyrimidinones [11], pyrroles [8], 4H-pyrans [12], flavones and chromones [13].
The chromone ring system, 1-benzopyran-4-one, is the core fragment in several natural product as flavonols, flavones, and isoflavones [14]. The chromone substructure has been defined as a privileged structure in drug discovery, due to its use in a wide variety of active compounds such as anti-inflamatory, anti-cancer, anti-HIV agents, cancer preventive and efficient agents in the treatment of cystic fibrosis. The most important chromone-based compound present in the nature are 2-arylsubstituted chromones (flavones), which are present in the different food source as tea, fruits, wine, and oil. The different substituent of the chromone ring determines their different biological activities [15].
One of the most classic methods for the synthesis of 2-arylchromones is the cyclodehydration of 1-(2-hydroxyphenyl)-1,3-diketones. Particularly, the cyclodehydration of 1-(2-hydroxyphenyl-3-(2-furyl)-1,3-propanodione to obtained 2-(2-furylchromone) were usually catalyzed and it has been performed in different reaction media. Catalyst and reaction medium include: acetic acid [16], hydrogenchloride in acetic acid [17 - 19], sulfuric acid in acetic acid [20], and heteropolyacid in acetonitrile [21].
For its part, the chromanone substructure are present in flavanone compounds (2-phenylchroma-nones). They also exhibit ample range of bioactivities that include hypotensive, anti-fungal, anti-bacterial, and antitumoral [22].
The classical method for the synthesis of fla-vanones consists of an intramolecular conjugated addition of o-hydroxychalcones to the corresponding cyclic carbonylic system [22]. Several catalysts were used to performed the 1-(2-hydroxyphenyl)-3-(2-furyl)-2-propen-1-one to the corresponding 2-(2-furyl)-chromanone that including: aq. buffer, T= 20 °C, pH= 12 [23], phosphorus pentoxide in ethanol [24], ytterbium (III) triflate [25], polyethylene glycol-400 [26], 1-butyl-3-methylimidazolium under microwave irradiation [27], sodium perborate in water/acetonitrile [28], sodium acetate in ethanol [29], and methane sulphonic acid [30].
In this article we present a systematic study about the use of a hybrid system bases on the combination of silica-alumina and Keggin heteropolyacid as catalyst in the selective cyclodehydration of 1-(2-hydroxyphenyl)-3-(2-furyl)-1,3-propanodione in solvent-free conditions (Scheme 1). Similarly, 1-(2-hydroxyphenyl)-3-(2-furyl)-2-propen-1-one was cy-clized to 2-(2-furyl)-2-chromanone (Scheme 2). The optimal conditions, using the most active material (SUIPMoT250) was used in the synthesis various of 2-(2-furylchromones) and 2-(2-furylchromanones).
2 Experimental
2.1 General
All organic and inorganic chemical were purchased from Aldrich or Fluka and used without purification. The 1-(2-hydroxyphenyl)-3-(2-furyl)-2-propen-1-ones and 1-(2-hydroxyphenyl)-3-(2-furyl)-1,3-propanediones were prepared using previously reported methods [20, 21]. The yields were calculated from pure products. All the products were characterized by comparison of melting point (mp), thin layer chromatography (TLC) and nuclear magnetic resonance (NMR) data with those reported. Samples were prepared in CDCl3 with tetramethylsilane (TMS) as an internal standard. The chemical shifts and coupling constants (J) were expressed in d and Hz, respectively.
2.2 Support preparation
The supports were prepared using three different methodologies previously developed in our laboratory with some modifications [31]:
Methodology A: the mixed oxide was prepared from tetraethyl orthosilicate (TEOS) and aluminum tri-sec-butoxide (TSBA). The molar ratio TEOS: TSBA: absolute ethanol (EtOH) and water (H2O) was 1:1:2:8. First TEOS was hydrolyzed for 3 h at 45 °C. Then, an ethanol solution of TSBA was added and the mixture was stir during 24 h. The formed solid were drying at 50 °C for 48 h and at 95 °C for 4 h. The support was named as SUIPMo0T25.
Methodology B: a solution of TEOS in absolute ethanol was prepared. Then, alumina Aldrich, 10 um particle size was added. The TEOS hydrolysis was performed by addition of distiller water at 40 °C. The molar ratio TEOS:EtOH:H2O was 1:1:4. After 24 h, the solid was filtered, dried at 50 °C for 48 h, and at 95 °C for 4 h. The materials was named SUIIPMo0T25.
Methodology C: a solution of TEOS, TSBA, EtOH and H2O (1:1:2:8) was prepared and stirring at 45 °C 3 h. The formed solid were drying at 50 °C for 48 h for 95 °C for 4 h. The material was named SUIIIPMo0T25.
2.3 Catalysts synthesis
The supports (SUI, SUII, SUIII) were impregnated using the incipient wetness method with ethanol-water solutions (50% v/v) of PMo Aldrich (H3PMo12O40.nH20). Six catalysts with two amounts of the PMo (10 % w/w and 20 % w/w) were prepared. The materials were named SUIPMo10T25, SUIPMo20T25, SUIIPMo10T25, SUIIPMo20T25, SUIIIPMo10T25, and SUIIIPMo20T25 respectively.
2.4 Catalysis calcination
Particularly, SUIPMo20T25 was calcinated different temperatures (150, 250, and 400 °C) to obtaine the corresponding materials: SUIPMo20T150, SUIPMo20T250, and SUIPMo20T400.
2.5 Catalysts characterization
The complete characterization of these materials was reported by our research group in previous works. Only some characterizations were made to verify the identity of the material. In the supplementary materials we added de characterization of the most active catalyst (SUIPMo20T250).
2.6 Catalytic test for the 1-(2-hydroxyphenyl)-3-(2-furyl)-1,3-propanedione cyclization
Initially, catalytic tests in order to analyze the effect of catalyst type, material calcination temperature, % active phase, catalyst amount, time, and temperature on reaction yields were made. The general procedure was performed in a test tube reaction and immersed in an oil bath.
A mixture of 1-(2-hydroxyphenyl)-3-(2-furyl)-1,3-propanedione and catalytic amount of the catalyst in solvent-free condition was subjected to the temperature and time of the experiment. Reactions were monitored by gas chromatography (GC), with Shimadzu GC-2014 equipment according to the following conditions; detector temperature, 320 °C; injector temperature, 200 °C; split ratio, 1:10; pressure, 60 kPa; purge flow, 3.0 mL min-1; and using a flame ionization detector (FID). The reaction yields were defined as the molar ratio of the product 2-(2-furyl)-chromone to the initial concentration of reactant.
2.7 General procedure for the synthesis of 2-(2-furyl)-chromone
A mixture of the corresponding 1-(2-hydroxy-phenyl)-3-(2-furyl)-1,3-propanediones (0.5 mmol), catalytic amount of catalyst (SUIPMo20T250, 1 mmol %) in solvent-free condition was subjected to 110 °C for 1 h. When the reaction time was over, the catalyst was washed (toluene, 2 x 1 mL) and filtered off, and the product was recrystalized using ethanol as solvent. Stability tests of the catalyst were carried out running six consecutive experiments under the same reaction conditions. After each test, the catalyst was separated from the reaction mixture by filtration, washed with toluene (2x1 mL), and then it was dried under vacuum and reused.
2.8 General procedure for the synthesis of 2-(2-furyl)-chromanone
A mixture of 0.5 mmol 1-(2-hydroxyphenyl)-3-(2-furyl)-2-propen-1-one and the catalytic amount of catalyst (SUIPMo20T250, 1 mmol %) in solvent- free condition was heated in a sealed tube with stirring for 4 h at 140 °C. When the reaction time was over, 2 mL of toluene was added in portions (2x1 mL), and the catalyst was filtered. The extracts were combined and the organic solution was concentrated the vacuum, giving the pure product. The solid products were recrystallized from methanol. Similarly, to the 2-(2-furyl)-chromone synthesis the stability tests of the catalysts were carried out running six consecutive experiments.
3 Results and discussion
The materials were prepared and characterized following a procedure described in the literature by our research group [31]. Table 1 presents the results of the surface area and the acid strength of some synthetized materials
Optimum reaction conditions were examined for 2-(2-furyl) chromone synthesis using 1-(2-hydroxyphenyl)-3-(2-fury)-1,3-propanodione as test reaction substrates in absence of solvent (solvent-free condition) at 110 °C for 1 h.
In the test experiment without the presence of catalyst, traces of 2-(2-furyl)-chromone was obtained (2 %) (blank experiment, Entry 1 in Table 2).
Table 2 Effect of different synthetized materials on 2-(2-furyl)-chromone yields.
Nota: Reaction conditions: substrate (0.5 mmol), catalyst (1 mmol %), temperature (110 °C), solvent-free, time (1 h), stirring.
In a second test, when the support (SUIPMo0T25) was utilized and the same reaction condition a very poor yield of only 15 % was obtained (Entry 3 in Table 2). Similar results were obtained with the support SUIIPMo0T25 and SUIIIPMo0T25 (Entries 4 and 5 in Table 2).
In the next experiment, bulk Keggin heteropolyacid (PMo) were checked in the same reaction condition. In this case, the reaction yields improve to 53 % (Entry 2 in Table 2).
In previous works we found that the preparation of hybrid material based in a support and a HPAs increases notably the yields of the reaction. Likewise, the supported/HPAs material allow a better separation of the reaction medium and its subsequent reuse.
For example, similar materials were employed in the selective pyranylation/depyranilation of phenols with excellent reaction yields [31].
For this raison, the next experiments were performed using the prepared hybrid materials. Six experiment using the system SUI, SUII and SUIII with two content of active phase of the catalyst (10 and 20 %) were tested.
The catalysts with high content of PMo (20%) showed elevated reaction yields unlike the others with low PMo content (10%) (see Table 2: SUIPMo10T25 62 % vs SUIPMo20T25 69 %; SUIIPMo10T25 48 % vs SUIIPMo20T25 55 %; and SUIIIPMo10T25 44 % vs SUIIIPMo10T25 5 3 % respectively).
Then, we studied the hybrid materials that contain the PMo, which was supported in the different prepared materials (varying the synthesis method) (SUI, SUII, and SUIII).
With both catalysts (high, 20% and low,10% content) a decrease in the yields of 2-(2-furyl) chromone was observed when passing the material prepared with support I (SUI) to support III (SUIII). The observed order for the catalysts with higher content of PMo was: SUIPMo20T25 > SUIIPMo20T25 > SUIIIPMo20T25 (Entries 9,10 and 11).
The best chromone yield was observed when the catalysts with the highest acidity were used (entries 3-5 in Table 1), independent of the material surface area, (SUIPMo20T25 69 % entry 9 in Table 2). The higher acidity associated with the smaller surface area is related to a lower interaction between the support and the PMo. The supports SUII and SUIII have higher surface area and then a higher number of surface groups compared to SUI carrier, increasing the PMo-carrier interaction and leading to well-dispersed PMo-phase. This increase leads to a decrease of the acidic strength in SAII and SAIII-supported catalysts [31].
The low interaction between the SUI and the PMo due to its low surface area could cause loss of catalytic activity in the different reuses of the catalyst, due to a greater leaching during the isolation and recovery phase of the catalyst (this operation is carried out using free toluene). For this reason, it is necessary to perform a leaching test. The fresh catalyst (SUIPMo20T250, 0.5 mmol) was put in contact with dry toluene (5 mL) in order to evaluate the possible catalyst solubilization. The catalyst was refluxed (110 °C) in toluene for 5 h, separated by filtration and dried in vacuum to constant weight. The observed mass loss was less than 0.2 %.
The reaction was also evaluated in order to study the effect of the catalyst dehydration temperature. The tests were performed using the materials SUIPMo20 without dehydration and dehydration at different temperatures: 150, 250, and 400 °C (SUIPMo20T150, SUIPMo20T250, and SUIPMo20T400 respectively.
The highest yield was attained using SUIPMoT250 (90% Entry 13 in Table 2).
In general, the yields employing dehydrated HPAs are higher than those using HPAs without dehydration. When HPAs are dehydrated, the number of water molecules in the secondary structure is lower, so that the acid strength and, consequently, the catalytic activity increases [21].The reaction is reversible, the secondary product is water, therefore, the presence of water discourages the displacement of the reaction equilibrium (chromone formation is best performed using anhydrous aprotic solvent or solvent free conditions). Finally, the catalytic system calcined at 400 °C (SUIPMoT400) gave very poor yields 39 % (see entry 14 in Table 2). In this case the low reaction yields are due to their low acidity as consequence of HPAs Keggin unit degradation [31].
Afterwards, the best catalyst, SUIPMo20T250 was studied in the next experiment and excellent result were obtained. The influence of temperature reaction on 1 -(2-hydroxyphenyl)-3-(2-furyl)-1,3-propanodione cyclization were analyzed using 1 mmol % of, SUIPMo20T250. Six temperature were studied (25, 80, 90, 100, 110 and 120 °C. No reaction was observed at 25 °C (entry 1 in Table 3), and the yields was very low at 80 °C (23 %, entry 2 in Table 3).
Table 3 Effect of different reaction conditions on 2-(2-furyl)-chromone yields (%).
Nota: Reaction conditions: substrate (0.5 mmol), catalyst: SUIPMo20T250 (1 mmol %), solvent-free, stirring.
When the reaction temperature increases to 90 and 100 °C (49 and 68 %, entries 3 and 4 in Table 3) a moderate yield of 2-(2-furyl)-chromone was obtained. A further temperature increase, to 110 °C leads notable increase of the yield (90 %, entry 5 in Table 3). This temperature favors the displacement of the equilibrium of the reaction by loss of water.
Finally, at 120 °C, the reaction yield is a little (85 % entry 6 in Table 3). In this case secondary products begin to be detected by CG analysis. For this reason, a temperature of 110 °C was employed in the next experiment.
The test performed using different reaction time under the same reaction conditions (substrate: 0.5 mmol, catalyst: SUIPMo20T250, 1 mmol %, temperature: 110 °C, and solvent-free conditions) shows that the yields of 2-(2-furyl)-chromone increased when the reaction time increased to 1 h (entries 5 and 9 in Table 3)
The catalyst amount was also studied in the same reaction condition using a variable mmol % of the SUIPMo20T250 (0.25, 0.5, 1.5 and 2 mmol %). The yield of 2-(2-furyl)-chromone increased from 68 % (entry 10 in Table 3) to 90 % (entry 5 in Table 3) and no appreciable changes of the yields were obtained with an increase of 2 mmol % in the catalyst amount (89 % entry 12 in Table 3).
The reuse of the catalyst of SUIPMoT250 was analyzed by the synthesis of 2-(2-furyl)-2-chromone in solvent-free condition at 110 °C using an amount of 1 mmol % (Table 4).
Table 4 Catalyst reuse. Effect on 2-(2-furyl)-chromone yields (%).
Nota: Reaction conditions: substrate (0.5 mmol), catalyst (SUIPMo20T250, 1 mmol %), solvent-free, temperature (110 °C), time (1 h), stirring.
The catalyst was recovered and reused five times and it can be observed that the materials maintains the catalytic activity. Only a loss of 5% the catalyst mass was lost and by FT-IR, it was noticed that the SUIPMo20T250 preserved the Keggin structure after all catalytic cycles. The results showed that the reutilization in six consecutives test were: 90, 89, 89, 87, 86, and 86 % respectively (Entries 1-6 in Table 4).
The auspicious results obtained in the synthesis of 2-(2-furyl)-chromones motivated us to use the SUIPMo20T250 catalyst in the synthesis of 2- (2-furyl) -chromanones. The reaction carried out by cyclodehydration of 2-hydroxyphenyl-3-(2-furyl)-2-prpopen-1-one as can be seen in Figure 2.
Similarly, to the synthesis of 2-(2-furyl)-chro-mone different reaction conditions including temperature, reaction time and amount of catalyst were studied in solvent-free conditions. However, when the reaction was studied at 110 °C quantitative amount of substrate was recovered, and no reaction product was detected. Next, the reaction temperature was raised to 140 °C (sealed tube). In this condition good yield of 2-(2-furyl)-chromone were obtained within secondary product formation.
The experimental conditions were: substrate (0.5 mmol), catalyst (1 % mmol), temperature (140 °C), reaction time (4 h), in a sealed tube with stirring. In this condition, the yield of 2-(2-furyl)-chromanone was 72 %. The reuse of the catalyst of SUIPMo20T250 was analyzed and the results showed that the reutilization in six consecutives test were: 72, 70, 68, 66, and 66 % respectively (entry 4 in Table 5).
Table 5 2-(2-Furyl)-chromones and chromanones synthesis using SUIPMo20T250 as catalyst.
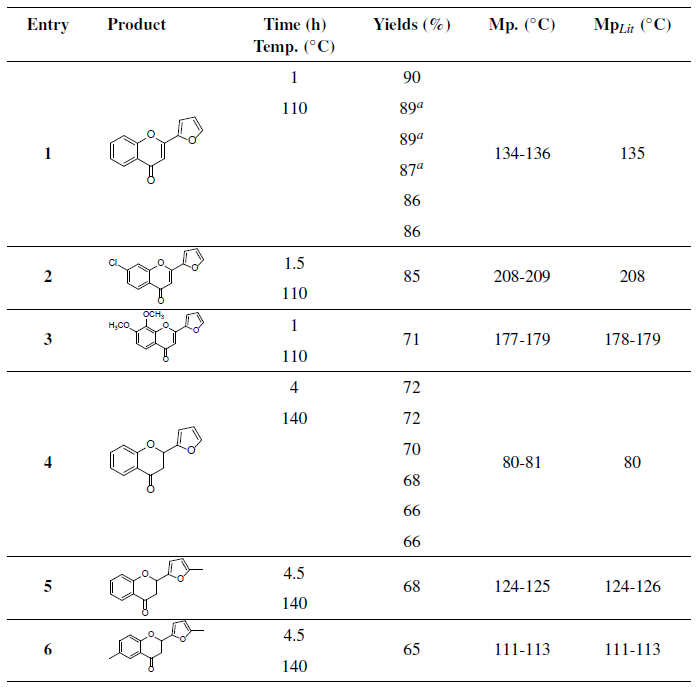
Nota: 1-Reaction conditions for 2-(2-furyl)-chromone synthesis (Entry 1-4): substrate (0.5 mmol), catalyst (SUIPMo20T250, 1 mmol %), solvent-free, stirring. 2-Reaction conditions for 2-(2-furyl)-chromanone synthesis (Entry 5-7): substrate (0.5 mmol), catalyst (SUIPMo20T250, 1 mmol %), sealed tube, stirring.
Finally, under the optimized reaction condition, three 2-(2-furyl)-chromones and three 2-(2-furyl)-chromanones were synthetized. The results are given in the Table 5. In all the examples the expected products were obtained with very good yields and excellent selectivity without appreciable amounts of secondary products. It can be observed in Table 5 that the proposed methodologies give very good yields of 2-furyl-2-(chromones) (71-90 %) and (2-(2-furyl)-chromanones (65-72 %) respectively.
In order to quantify how much 'greener' the methodology is, the Atom Economy (AE), Atomic efficiency factor (E), Process Mass Intensity (PMI), Reaction Mass Efficiency (RME), Material Recovery Parameter (MRP) and EcoScale were calculated for each reaction product. The results are given in the Table 6.
Results show high efficiencies, very low recovery parameters, and acceptable to excellent green reaction conditions, incontrast with classic synthesis methods [22].
4 Conclusions
The preparation and characterization of materials based on a Keggin Heteropolyacid supported on silica-alumina was reported with the object of recycling the catalyst efficiently after the reaction, and to carry out reaction for the 2-(2-furyl)-chromones and chromanones synthesis (containing the furyl group, building block obtainable from biomass). The supports were prepared by sol-gel methods and the supported catalysts by impregnation techniques in a mixture of ethanol-water (1:1) was satisfactory. The material maintained their structure after the synthesis and were characterized by routine techniques and the acidity was determined by potentiometric titration.
Three 2-(2-furyl)-chromones and three 2-(2-furyl)-chromanones synthetized using the most suitable catalyst (SUIPMo20T250) under solvent-free conditions. The catalytic methodology represents an environmentally friendly procedure in comprehensive, compared with other systems (soluble acids such as acetic, hydrochloric, and p-toluensulfonic acids or high cost catalyst as ytterbium (III) triflate).
The of the hybrid materials based in silica/alumina/HPAs give very good yields (65 to 90 %), and easy separation and recovery of catalyst. The catalytic activity was practically constant in consecutive runs, and the high recovery of the materials allows low cost and environmental impact.
The utilization of these material in the suitable synthesis of the other heterocycles containing building block present in biomass are in progress in our laboratory.