1 Introduction
The current population growth and economic development are increasing, which is strongly related to the generation of urban solid waste, and this provides a challenge towards environmentally sustainable development [1,2]. In this context, solid waste management in Latin America has been a challenge because of its continuous increase in quantity and diversity, and Colombia is a particular case since approximately 97% of the waste generated is disposed of in landfills [3], being this the most common approach but causing a problem because of the production of highly contaminated leachates [4]. Likewise, the landfill technique is one of the most used alternatives not only in the country but also in the department of Norte de Santander.
As a consequence of the compacting of solid waste in landfills, there are a series of physical and chemical changes that lead to the generation of leachates [5]. Similarly, the decomposition of these wastes and the percolation of rainwater over the cells where they are disposed to contribute to their production volume [6]. These leachates can be transported and contaminate surface and groundwater, which supports the need for adequate treatment before disposal [7-9]. The location of sanitary landfills in Norte de Santander supports the previous problem since they are built near streams or river crossings, which eventually flow into larger water sources that serve as the water supply for the population, which also highlights the impact on the communities surrounding these sites.
These leachates are characterized by a dark brown or black color with a very high concentration of chemical oxygen demand (COD), ammonia nitrogen, heavy metals, and other organic and inorganic contaminants [10], in addition, many types of contaminants of emerging concern (CECs) have been found in concentrations that may pose a risk to nearby water sources and surface water ecosystems [11], highlighting its high potential to contaminate soil and groundwater nearby, if not collected and treated. Therefore, leachate management is an important aspect [12], hence in the context where this research is developed, the technologies for the treatment and control of this residual liquid are direct recirculation to waste cells and evaporation by solar or artificial action [13, 14] but have represented little success because of technical and financial sustainability as treatment systems [15] and particularly under these alternatives is not providing a real solution to the problem, but the impact is being transported to other scenarios.
Knowing the high pollutant loads and recalcitrant characteristics of leachates, it is necessary to propose alternatives for their treatment capable of withstanding their toxic conditions, that is where the Advanced Oxidation Processes (AOP) based on the in situ generations of highly reactive oxygen species, are efficient in the decomposition and satisfactory mineralization of toxic and recalcitrant pollutants [16, 17], but the intensive use of reagents and energy that they require, makes the operating costs become a limitation of the system [18, 19], therefore, they can be implemented as a pretreatment to obtain wastewater with conditions suitable for subsequent biological treatment because the recalcitrant characteristics are reduced and the biodegradability is enhanced [20, 21]. This technique is known as AOP-Biological couplings and makes the proposed system an alternative of low energy cost, efficient removal of pollutants, and generation of non-toxic by-products [22], which provides promptly and more promising results.
Several investigations validate the AOP-Biological couplings as viable for the decontamination of leachates, as is the case of [23] who in the biological process obtained removals of 38% and 24% for COD and DOC, and after the process coupled to photocatalysis, the elimination of pollutants was favored up to 68% and 76% for COD and DOC. Similarly, [24] evaluated the efficiency of photocatalysis coupled to an anaerobic biological process mediated by SMA, for the removal of pesticides, resembling the low biodegradability and recalcitrant conditions shared with leachates, where they obtained an improvement in COD removal from 46.4% in the biological process to 72.2% after coupling. Research has also been reported, in which the coupling to the biological process achieves enhanced removal of contaminant load in leachates, as in the case of [25] who in photocatalysis obtained a reduction of COD of 50% and after coupling to bioreactors the elimination of COD amounted to 87%.
Based on the described information, it is proposed to apply the described technique to the decontamination of leachates generated in a sanitary landfill in the department of Norte de Santander, with an ambient temperature of 33 °C and a warm climate, opting for adequate conditions to use the sun's energy as a source of UV radiation. First, the capacity of the SMA to treat the leachate is studied, then the study conditions in the AOP are optimized to determine the maximum mineralization in the operational aspects analyzed; finally, the coupling between the two processes comprises the biological process receiving the effluent coming from the AOP and thus learning how the chemical oxidation improves the conditions of the leachate for its subsequent biological degradation and enhance the degradation.
For this research, the process of heterogeneous photocatalysis catalyzed by Titanium Dioxide (TiO2) Degussa P25 and photoassisted by UV solar radiation is proposed, knowing that the use of a semiconductor photocatalyst subjected to specific radiation, allows oxidation-reduction processes that make possible the removal of contaminants in leachates [26, 27] and also the use of Hydrogen Peroxide (H2O2) that works as a coadjuvant in the oxidation [28]. Such AOP is coupled to an anaerobic biological process, assisted by the SMA test following the volumetric method [29].
This study developed the heterogeneous photo-catalysis process in a Response Surface method (RSM) with a factorial design in the variables of pH and catalyst concentration, as well as fixed cumulative energy ranges where the DOC response variable will be measured. Regarding the SMA biological process, the technique consists of the quantification of methane production through the use of a displacer substance. All this to apply a technological and economically viable option for the degradation of leachate, promoting the protection of natural resources and public health.
2 Materials and methods
Figure 1 illustrates the 4-phase methodological process that was carried out to meet the objectives of the research: in Phase 1, the leachate sample is taken from the landfill and characterized in the laboratory for the physicochemical parameters of interest that will be detailed further on; this summarizes the conditions of the wastewater to be treated and the starting point for the other processes. Subsequently, in phase 2, the biological part of the research is developed, which is related to the characterization of the sludge inoculum and the application of the Specific Methanogenic Activity Test, which results in the leachate's treatability under anaerobic conditions. Phase 3 comprises the development of the heterogeneous photocatalysis process, which will provide the optimum treatment conditions in terms of pH and catalyst dosage. Phase 4 comprises the coupling between the optimal processes developed in phases 2 and 3, and thus to know the contribution of the proposed system to the elimination of the pollutant load in the leachate.
All the analyses previously described were developed in the Environmental Quality Laboratories of Francisco de Paula Santander University, at Eliseos Campus, and under all the biosafety protocols required to apply the techniques, they were also based on all the guidelines proposed in the Standard Methods for the Analysis of Water and Wastewater (AWWA - APHA - WPCF) in the cases in which they were applied. The methodological phases are detailed below.
2.1 Leachate characterization
The characterization of the leachate was developed according to the Standard Methods for the Analysis of Water and Wastewater and the parameters analyzed: Dissolved Organic Carbon (DOC) following the protocol established in section 5310 B and using the Teledyne Tekmar TOC Torch equipment. Chemical Oxygen Demand (COD) by the closed reflux method established in section 5220 C.
The Total Suspended Solids (TSS) and the Volatile Suspended Solids (VSS) were analyzed by the Gravimetric method at 103-105 °C established in section 2540 B using an analytical balance, a desiccator for porcelain capsules and crucibles, a digital oven, and an electric muffle.
The pH and temperature parameters were taken with a multiparameter (Waterproof PCTestr 35) at the landfill on the day of leachate sample collection.
2.2 Leachate Anaerobic biodegradability
2.2.1 Characterization of inoculum (sludge)
The activated sludge inoculum used during the research was extracted from the second anaerobic lagoon of the wastewater treatment of the primary production process of wine palm (corozo) oil from the oil extraction plant of the Pal Norte Company, which is located in the farm La Natalia Kilometer 15 on the township of Campo Dos in the municipality of Tibú. This sludge was characterized in the physicochemical parameters of pH, temperature, TSS, VSS, and COD, to determine its effectiveness in the use of inoculation of anaerobic biological reactors. The sludge volume index (SVI) test was applied to this sludge inoculum to determine its sedimentation properties. The measurement of the physicochemical parameters listed above was developed following the standard methods for water and wastewater analysis, edition 20/AWWA-APHA-WEF.
Anaerobic sludge is favored by high values in TSS and SSV parameters because their presence indicates a higher organic loading rate and more biogas generation [30]. Temperature and pH are important and controlling factors in the use of anaerobic sludge because they significantly affect the process [31]. Finally, the characterization of these parameters allows determining that the collected sludge has optimal characteristics for the growth of microorganisms and that it is suitable for use as inoculum in biodegradability tests [24].
2.2.2 Specific Methanogenic Activity (SMA) test
This test allows quantifying the maximum methane production capacity by the group of microorganisms present in anaerobic sludge [32]. To evaluate the biodegradability of the substrate, NaOH (3%) was used as a displacing substance; it is important to maintain a pH higher than 12, to facilitate the reaction of the substrate with the CO2 generated.
The theoretical methane production was calculated under the conditions of temperature and atmospheric pressure of SMA development and was corrected according to the expression described in equation 1 [33]:
Where:
K(t) is the correction factor (gCOD.L-1).
P the atmosphere pressure (atm).
R the constant of gases (0.08206 atm.L. (mol. °C)-1).
K is the digested organic load corresponding to one mole of CH4 (64 gCOD.mol-1).
T the operational temperature of the assembly (°C).
To conduct the test, a concentration of 2.0 gVSS.L-1 of inoculum sludge was used because it was a test without agitation. The volume of sludge to be added was calculated considering that the mixture of inoculum and substrate should not exceed 90% of the useful volume of the biological reactor (900 ml); it was calculated as follows [29] according equation (2).
This type of test can be performed with DOC concentrations ranging from 3,500 - 4,500 mg.L-1 for the substrate [29] since this test does not involve agitation. In addition, the addition of a certain amount of nutrients is required to make the degradation kinetics in the reactor approach a zero-order reaction, depending only on the concentration of microorganisms present in the inoculum.
The location of Reactor 1 (R1) should be at a higher level than Reactor 2 (R 2 ), which prevents R1from being affected in case of sodium hydroxide (NaOH) suction due to negative pressures. In addition, the hose inside R2 is placed in a "U" shape to act as a barrier and hinder the passage of NaOH to R 1 . Figure 2 details the process earlier described.
Considering the methane production, the specific methanogenic activity (SMA) is calculated from the expression described in equation 3.
Where:
m is the maximum slope of the methane production curve (Vol. accumulated CH4 vs time).
M the sludge mass (Volumeof sludgeadded * Initialsludgeconcentration) (g).
Vch 4 the theoretical volume of methane produced (L).
For the calculation of the slope (m) a curve of "Accumulated CH4 volume" vs "Test time" must be constructed, the latter may be suspended once the curve becomes asymptotic [29].
The theoretical volume of methane is calculated with the expression described in equation 4:
Where:
2.3 Optimal conditions for leachate treatment using heterogeneous photocatalysis
The photocatalytic decomposition of the leachate was performed by heterogeneous photocatalysis, using a laboratory-scale solar Cylindrical Parabolic Collector (CPC), with an approximate reaction volume of 20 L, area of 0.83 m2, composed of two aluminum parabolic structures which refract the light, equipped with borosilicate glass tubes through which the fluid circulates, secured in a concrete structure. This CPC configuration of the photocatalytic reactor is the most widely used in water treatment because it combines an efficient radiant field and suitable hydrodynamic conditions in the removal of various pollutants, as well as in other engineering applications [34-36].
TiO2 (Degussa P-25) was used as catalyst [34, 37, 38]. H2O2 was used as the oxidizing agent, which is highly efficient when used in the development of wastewater decontamination treatments, mainly because of the generation of OH radicals, which enhance the removal of pollutants [39, 40], as showed by some investigations where the addition of H2O2 to the system led to an improvement in the average removal of pollutants than the TiO2-UV system alone [41].
UV radiation favors the generation of hydroxyl radicals since they irradiate the TiO2 nanoparticles to produce photogenerating holes where oxidation reactions take place for the removal of the studied pollutants [42]. Similarly, various investigations on photocatalytic processes have determined that they achieved high pollutant mineralizations at cumulative energies greater than 100 kJ.L-1 [43, 44] and considering that the aim of this phase is to eliminate only a percentage of the pollutant load to be subsequently subjected to the anaerobic biological process (coupling), a range of accumulated working energy from 220 kJ.L-1 a 60 kJ.L-1. is established.
The problem sample was prepared in the CPC tank, equipped with a 0.5 HP Humboldt pump and Qmax of 32 L.min-1. The UV radiation measurement was conducted with an adaptation of a pyranometer functioning as a solar radiation sensor, connected to a multimeter. A general scheme of the photocatalytic reactor is shown in Figure 3.
Response Surface Method (RSM) is a statistical tool based on fitting a polynomial equation to experimental data and has been applied to heterogeneous photocatalysis processes having TiO2 dose and pH level as independent variables in response to pollutant removal [45,46]. Therefore, in this research, the statistical program Statgraphics Centurion XV was used to combine randomly the various experiments associated with the catalyst dose of 100, 350 y 600 mg.L-1 of TiO2 and the pH levels 3, 6, and 9, under a factorial design 32. The 9 randomized experiments with their TiO2-pH combinations are represented in Table 1.
The selection of TiO2 and pH levels allows establishing a wide working range to know the behavior of the elimination of the pollutant load present in the leachates under the described operating conditions and thus, through the optimization of the results, to choose the most adequate operating condition in terms of economic and treatment efficiency.
Table 1 Photocatalysis optimization experiments.
Experiment | TiO2 Concentration (mg.L 1) | pH |
---|---|---|
1 | 100 | 9 |
2 | 350 | 6 |
3 | 600 | 3 |
4 | 600 | 9 |
5 | 600 | 6 |
6 | 100 | 3 |
7 | 100 | 6 |
8 | 350 | 9 |
9 | 350 | 3 |
The number of experiments described in Table 1 was carried out considering the established amounts of catalyst and pH units, constant concentrations of leachate (in terms of COD according to the characterization results), and H2O2. The duration or hydraulic retention time (HRT) of each test was a function of the solar radiation and therefore of the maximum accumulated energy reached, which depended on the climatic conditions of the area where the project was developed.
It is important to highlight that, during each test, the accumulated energy readings were taken in 10-minute intervals that define the time lapses. Subsequently, the mathematical conversion that 1 mV represents 5 W.m-2 must be applied because the results obtained directly are from the Multimeter reading, which gives the values in units of mV. Finally, equation 5 is applied to calculate the energy in the units of interest.
Where:
Q n : total accumulated energy kJ.L-1.
Q n-1 : previous accumulated energy kJ.L-1.
Δ tn : irradiation time (600 seg).
I n : average irradation (W.m-2 UV).
A f : irradiated reactor surface (0,83 m2).
V T : volume discussed (20 L).
Similarly, samples of the treated solution were collected at the beginning 0 kJ.L-1, at the intermediates of 20 kJ.L-1 y 40 kJ.L-1 and at the end of the process of 60 kJ.L-1. The stored samples were monitored by COD and DOC to determine the degree of mineralization of contaminants present in the leachate.
2.4 Leachate treatability in the coupling of the photocatalytic process and the anaerobic biological process
This stage evaluates heterogeneous photocatalysis as a pretreatment before the anaerobic biological process to assess whether chemical oxidation can improve conditions in biological oxidation. This coupling allows the fact that the structures of the pollutants are modified to convert them into less toxic and more biodegradable by-products [20, 22], being able to be disposed of without causing drastic alterations in the environment.
The methodological design used in this part of the research consisted of running the two treatments in sequence: first, the leachate is treated by heterogeneous photocatalysis, and then the effluent from this process is the input for the specific methanogenic activity test (anaerobic biological process). In this case, the advanced oxidation process is evaluated with the optimal experiment found during the experimental development in section 2.3., in terms of catalyst dose, pH level, and accumulated energy; the effluent leaving the AOP is the input for the SMA test, where the whole process described in section 2.2.2 is developed again.
Finally, the treatment ends when the specific methanogenic activity test is completed, where the VSS, TSS, and DOC parameters were monitored during its development, finally reporting the contribution that the coupled process has towards the elimination of pollutants and concluding if the previous chemical oxidation process (TiO2-UVsolar heterogeneous photocatalysis) improves or not the conditions of the toxic effluent for its subsequent degradation in anaerobic biological reactors and if the use of the coupled processes is much better than developing each process separately, enhancing the efficiency of the treatment.
3 Results and analysis
3.1 Characterization of the leachate
Samples of the problem leachate were taken from the main leachate collection point, coming from the solid waste compaction cells. Each of the samples taken was characterized to get an approximate range of the contaminant load for subsequent treatment and were analyzed by COD, DOC, TSS, VSS, pH, and temperature parameters, the latter two being measured at the collection point with a multiparameter, extracting a sample of the leachate in a beaker. The results of this first phase of the research are detailed in Table 2.
Table 2 Leachate characterization results.
Parameter | Units | Value |
---|---|---|
COD | mg O2.L-1 | 7,920-8,334.36 |
DOC | mg.L-1C | 2,756 - 2,777.59 |
TSS | mg.L-1 | 18,860-19,965.33 |
VSS | mg.L-1 | 8,192-8,821.33 |
pH | - | 7.29 -7.8 |
Temperature | oC | 32.6 -33.2 |
The results obtained in Table 2 allow inferring that the leachate presents high concentrations of DOC, which classifies it as young since mature leachates usually have a lower concentration of this physicochemical parameter [47] since the contaminants are more stabilized. This result is consistent because the target sample was taken from the key point of generation, i.e., the leachate comes directly from the disposal cells.
Similarly, [48] determined that those landfills in which only ordinary waste is treated stand out for high concentrations of organic matter in terms of TOC and BOD, which supports the DOC value reported in Table 2 and knowing that the leachate comes from ordinary solid waste disposal cells.
Leachates have recalcitrant characteristics that make them poorly biodegradable, this condition is calculated based on the BOD5/COD ratio and is normally less than 0.3 for these cases [49], however [50] validated the calculation of biodegradability as the DOC/COD ratio for leachates, having for our case a value of 0.34, which can corroborate the low biodegradability of this waste liquid [27].
The TSS and VSS contents reinforce the high content of organic matter present in the leachate. The pH condition classifies it in a value close to neutrality, while the temperature recorded is consistent with the landfill location area (min 24 °C - max 34 °C).
It is important to clarify that the physicochemical properties of the leachates are highly variable, attributing the reason to various factors such as the composition and depth of the waste, the availability of moisture and oxygen, the design and operation of the landfill, the age of the waste, the precipitation rate, among others [51].
3.2 Anaerobic biodegradability of leachate in biological reactors
3.2.1 Characterization of the inoculum (sludge)
The parameters analyzed in the anaerobic sludge were Total Suspended Solids (TSS), Volatile Suspended Solids (VSS), Chemical Oxygen Demand (COD), pH, and Temperature, and its sedimentability was determined using the Sludge Volumetric Index (SVI). The results of the inoculum characterization are presented in Table 3.
Table 3 Results of sludge characterization (inoculum).
Parameter | Unit | Value |
---|---|---|
pH | - | 6.6 -7.1 |
Temperature (T) | °C | 39 - 40.6 |
COD | mgO2.L-1 | 6,900 |
TSS | mg.L-1 | 24,124 |
VSS | mg.L-1 | 13,460 |
VSS/TSS | - | 0.557 |
SVI | ml.g-1 | 13.265 |
The results in Table 3 allow inferring that the sludge inoculum presents a suitable state for the growth of anaerobic microorganisms and methane production, according to the ranges reported by [52], pH of 6.5 to 7.5 and temperatures higher than 35°C.
The concentration of de 24,124 mg.L-1 of TSS and 13,460 mg.L-1 of VSS and their ratio of 0.557, show a sludge with outstanding characteristics because of having a high content of microorganisms [52]. The SVI value of 13.256 ml.g-1, places it within the range of sludge of excellent sedimentability, since a sludge with excellent sedimentation characteristics is considered having an SVI value below 80 ml.g-1 [53]. These results categorize the analyzed sludge as suitable to be inoculum in anaerobic biological reactors, favoring the degradation of the leachate.
3.2.2 Evaluation of the biological treatment (SMA)
The biodegradability test was conducted in 7 different reactors, each with a storage capacity of 900 ml. The first 3 corresponded to tests with leachate from the landfill (R1, R2, R3). The next two contained Volatile Fatty Acids (VFA) (R4 and R5). And the last two contained samples of the previously inoculated sludge (R6 and R7). Figure 4 shows the scheme described above.
Reactors R6 to R7 contained as feed a mineral medium containing macronutrients (N-NH4+, P-PO3- 4, Mg, Ca) and micronutrients (Fe, Ni, Zn, Co), alkalinity (NaHCO3 o KH2PO4 + K2HPO4), and a reducing agent (Na2S.7H2O). The reactors R 4 andR5 contained as substrate a mixture of VFA (acetic-C2, propionic-C3; and butyric-C4) [29]. Table 4 describes the culture media for each reactor with their corresponding addition volumes.
With reactors, R 1, R 2 and R 3 the degradation of the leachate was evaluated, while with R 4 and R 5 the effectiveness of the sludge against a substrate other than the leachate, in this case, AFVs, was determined.
Finally, reactors R 6 and R 7 were established as the targets to evaluate the sludge behavior.
The HRT was divided into two phases: the first phase corresponded to the initial set-up of the biological test in the SMA, which was carried out over 15 days, and where the acclimatization of the microorganisms was sought. The second phase corresponded to the reseeding of the reactors, which took place over 8 days and aimed to determine the percentage of leachate degradation after the previous acclimatization of the microorganisms.
Finally, an HRT was obtained for the biological process of 23 continuous days, in which samples were taken of the displaced volume of NaOH in each reactor, this was done twice a day at regular time intervals. It is important to ensure a temperature between 27°C and 34°C since this is the condition for the growth of methanogenic microorganisms [54].
In the first 8 days of treatment, an extensive amount of NaOH was produced, because of the development of the first phase of anaerobic digestion (hydrolysis), which comprises the breakdown of complex organic molecules [55]. While in the following 7 days, methane production was minimal compared to the initial days, since the bacteria were acclimatized, however, methane production rose again in the last 8 days, since for this period the reactors were reseeded, in this second phase the anaerobic microorganisms were adapted to the substrate. The accumulated volume during the biological test was 1300 ml for R 1 (leachate reactor), 521.2 ml for R2 (VFA reactor) and 1223.5 ml for R3 (white reactor). Figure 5 shows the results presented above.
The results of the parameters analyzed for the biological test in the 3 reactors during assembly and reseeding are presented in Tables 5 and 6 (R 1 -Leachate, R2-VFA, R3-White).
Table 5 Results of the SMA biological test in the first phase.
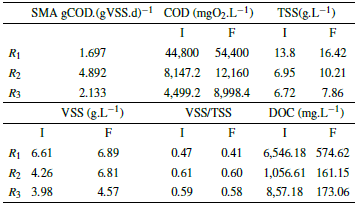
Nota: I: Initial phase. F: Final phase.
Table 6 Results of the SMA biological test in the second phase.
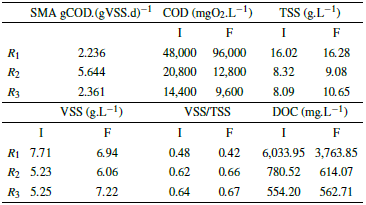
Nota: I: Initial phase. F: Last phase.
Methane production is increased, which is a mixture of methane and carbon dioxide, and the carbon dioxide is removed by a sodium hydroxide solution, so only methane is measured, which are presented in the second phase are because of the degradation of organic matter for the three reactors, as shown by the COD data (Table 5 and 6). It should be noted that the COD for R1 (leachate) did not present degradation (Table 5 and 6). However, this does not mean that no CH4 production occurred, but on the contrary, in R 1 two processes occurred simultaneously, the degradation of organic matter and cell lysis, which because of the toxicity of the substrate, comprises the rupture of the cell membrane of the bacteria presenting a release of intracellular material [55], which directly affects the amount of organic matter in the reactor.
The COD values during the first phase suffered an increase mainly because of the acclimatization of the microorganisms [55], similarly generating an organic matter removal of 0%; while in the second phase the COD increased again for R 1 (there was no degradation), while the COD for R 2 and R 3 decreased, presenting a removal efficiency of 38.46% and 53.13%.
In addition, it was observed that both TSS and VSS increased for the 3 reactors in the first phase, since the microorganisms were in a process of acclimatization, and cell lysis occurred [55]. While the VSS/TSS ratio decreased because of the increase in DOC, showing a reduction in the microorganisms present in the reactors. For the second phase, the TSS increased for R1 because the substrate was very toxic, producing the death of some microorganisms, while the VSS decreased due to the increase in DOC, this means that in the reactors there was a greater presence of inorganic than organic matter. On the other hand, in reactors R 2 and R 3 there was an increase in the TSS, due to the decrease of feed in the reactors, but the VSS of these reactors increased due to the decrease of COD.
The results of the mineralization measured in DOC for the first phase were 91.22%, 84.74%, and 79.87%, for R1, R2 y R3 respectively, while for the second phase there was less mineralization for the reactors evaluated, which is evidenced in the percentages of 37.63%, 21.32%, and 0%.
According to the results, it was possible to establish that anaerobic biological treatment alone is not efficient for leachate treatment, so integrated systems should be implemented because a single technology cannot achieve the expected treatment and a preliminary treatment is recommended to stabilize the concentration of contaminants [56,57]. However, several authors have got high degradations in terms of COD under anaerobic biological treatments such as the UASB reactor, but high costs were reported in the complete removal of contaminants [58,59], similarly [60] got COD degradations of less than 50% under the same technology and under long treatment times (310 days).
Even with the identified limitations, in wastewater treatment, anaerobic processes have gained importance and special attention mainly because of their economic merits over aerobic biological systems [61].
3.3 Optimal conditions for leachate treatment by photocatalysis
The leachate sample used for treatment was 1.02 L, which was mixed with water to complete 20 L of solution, to obtain a concentration of 400 mgO2.L-1 of COD of the pollutant load, because it seeks to treat the concentration range used and accepted in the anaerobic biological process, additionally it can have a better performance, since it would reduce the turbidity level that could prevent the solar rays from passing through the entire influent, preventing the absorption of light in the solution and the adsorption of pollutants on the surface of the catalyst [62].
The concentrations of TiO2 comprised 100, 350 and 600 mg.L-1 and pH values of 3, 6, and 9 units, as established for each assay. The oxidant (H2O2) was worked constantly with a concentration of 300 mg.L-1, a value optimized by [63] in a photocatalytic leachate treatment using (TiO2/UV). When the solution to be treated was ready, it was recirculated through the solar reactor, until the accumulated energy of 60 kJ.L-1 was achieved.
The results from each test are presented in Table 7, which show that at basic (high) pH the system does not have a good performance, reaching removal percentages below 50%, these values could be explained considering that at pH values above the isoelectric point of TiO2 (pH = 6.5) the adsorption of organic matter decreases [64]. With acid pH high efficiencies are achieved, hence the optimum dose to perform the coupling, according to the mineralization percentages is 100 mg.L-1 de TiO2 at a pH of 3 units.
It is important to highlight that acidic solutions help the adsorption of the pollutant on the surface of the TiO2 catalyst, similarly favoring the percentage of degradation in the photocatalytic process [65]. This is justified by several investigations that have shown that at acidic pH the surface of the catalyst is positively charged, which leads to the attraction of negatively charged organic pollutant compounds, generating an important photocatalytic activity [66, 67]. Similarly, the best results for heterogeneous photocatalysis are obtained at acidic pH [68, 69].
The photocatalytic process achieved a mineralization of 57.90% in terms of DOC for the highest energy, corroborating this using the results of the Response Surface Analysis performed by Statgraphics software as shown in Figure 6.
Considering the interpretation of DOC as total mineralization of carbonaceous organic matter [70], and analyzing the behavior of the parameter, it is identified that the treated problem sample was mineralized in 57.907 % for the maximum accumulated energy evaluated (60 kJ.L-1). This result is acceptable since the effluent is not required to present a total degradation since it will serve as pretreatment in front of the biological process.
According to the above, photocatalysis cannot be disqualified as an alternative to treat leachate, since the main interest is to make a coupling with biological processes, where this partially decontaminated effluent is polished by the action of the microorganisms of the biological reactor, which is justified because the AOPs emerge as an adequate pretreatment because chemical oxidation allows eliminating non-biodegradable and hazardous compounds, and in combination with a biological process significantly reduces costs [57, 71].
3.4 Treatability of the leachate in the coupling of the photocatalytic process and the anaerobic biological process
The first stage of the coupling comprised the evaluation of the photocatalytic process by performing the test again with the optimum dose (concentration of 100 mg.L-1 of TiO2 at a pH of 3 units), obtaining a mineralization percentage of 56.56%, with an HRT of 3.40 hours. The lower HRT reported than the previous one, which was 4.66 hours, is because of this optimum test the solar radiation conditions were stronger, i.e., the day was sunnier.
The second stage consisted of evaluating the treatability of the effluent produced in the photocatalytic test, which became the effluent of the biological test. Here, only the physicochemical parameter of DOC was analyzed, which was used as a comparison input for the subsequent anaerobic biological degradation and to measure the efficiency of the AOP.
The assembly of the biological coupling test (SMA) lasted 23 continuous days similarly: one day 1, the initial assembly was carried out with the conditions described in the method, remaining similar for the first 15 days. After this, a reseeding was carried out, which comprised adding the same concentrations of the problem sample: VFA and nutrients as one day 1, to have acclimatized sludge and getting better yields, ending the test after the following 8 days.
Table 8 summarizes the results of the mineralization percentages in terms of DOC in each of the treatment phases applied for the decontamination of the landfill leachate.
According to the information described in Table 8, the development of the anaerobic biological process alone did not provide any pollutant degradation because of the recalcitrant characteristics of the leachate, as mentioned in the previous section regarding this phase of the research. The heterogeneous photocatalysis process with SMA contributed a 57% mineralization of the leachate in terms of DOC, which corroborated the capacity of these AOPs to treat non-biodegradable substances and with toxic compounds.
Finally, the combination of the two processes provided an increase in leachate mineralization of 21% in terms of DOC, for a total contribution of 78% of the coupling, which shows the capacity of the AOP as a strong pretreatment to convert toxic wastewater into another with more suitable characteristics for subsequent degradation in anaerobic biological reactors, which is showed by the capacity of the SMA to receive the treated effluent and provide an additional mineralization of 21%, about the first test conducted where there was no mineralization.
The treatment time of the coupling was less than 24 days: biological treatment of 23 days and photocatalytic treatment of 3.4 hours, which shows an efficient and low-cost alternative since the AOP-Biological configuration reduces the time of chemical oxidation and enhances biological oxidation, which contributes to the decrease in treatment costs and improves process efficiencies.
Current research validates the results obtained, such as the case of [72] who with a treatment between a bioreactor with anoxic conditions and a TiO2/UV photocatalysis process achieved 82% COD removal from a leachate, under optimal conditions of acid pH and the second-lowest catalyst dose. [73] treated landfill leachate under a system combining an advanced process and an anaerobic biological process and found that the biological alone provided 11.9% COD removal, while the coupling of the two systems provided 68% COD removal for the same parameter, concluding that the coupling reduces reagent consumption and costs.
4 Conclusions
Heterogeneous photocatalysis as a primary treatment presented good degradation yields (57.90%), with 100 mg.L-1 de TiO2 being the concentration with the best performance, since the lower the turbidity, the greater the absorption of light in the solution, indicating that photocatalysis presents good yields in acid solutions since they help TiO2 to better adsorb the contaminants on the surface of the catalyst.
In the first phase of the biological process (SMA), there was no biodegradation of the organic load in the three reactors, since during this period, the microorganisms acclimatized to the operating conditions. In the second phase, in R1, there was no COD removal, showing that the leachate had high toxicity and low biodegradability (despite the results obtained in its characterization), while in R 2 y R 3 percentages of 38.46% and 53.13% were generated, respectively, indicating that the inoculum was not a limiting factor in the biodegradation process. Consequently, it can be concluded that the toxicity and biodegradability of a substance are directly related, since the higher the level of inhibition of the microorganisms, the lower the percentage of biodegradability.
For accumulated energies of maximum 60 kJ.L-1 photocatalysis acts on the leachate, transforming it into less toxic and more biodegradable substances without completely mineralizing it.
During the biological process of the coupling, the yield of R 1 (leachate sample) is attributed to the previous treatability of the leachate in the photocatalytic process, which generated an effluent assimilable by the microorganisms facilitating the degradation identifying better yields compared to the anaerobic biological process alone. This is because photocatalysis is efficient for non-biodegradable pollutants and biological treatments are always the most economical processes, therefore, only when the pollutants are difficult to degrade, photocatalytic processes are suitable.
The mineralization rate of photocatalysis for leachate treatment is higher compared to the mineralization rate presented in biological reactors. Through the photocatalytic processes, DOC removals of 57.90% are achieved in 4.16 hours (the time it took to reach cumulative energy of 60 kJ.L-1), while in the biological coupling process the additional contribution was 21% in 23 days.